Exploring Electromagnetic Radiation: Key Concepts and Uses
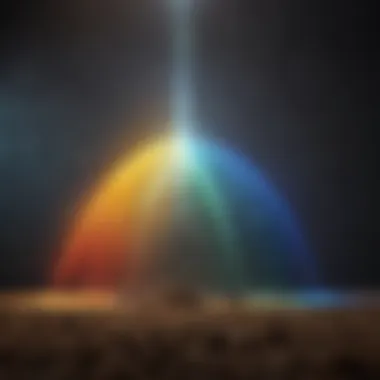
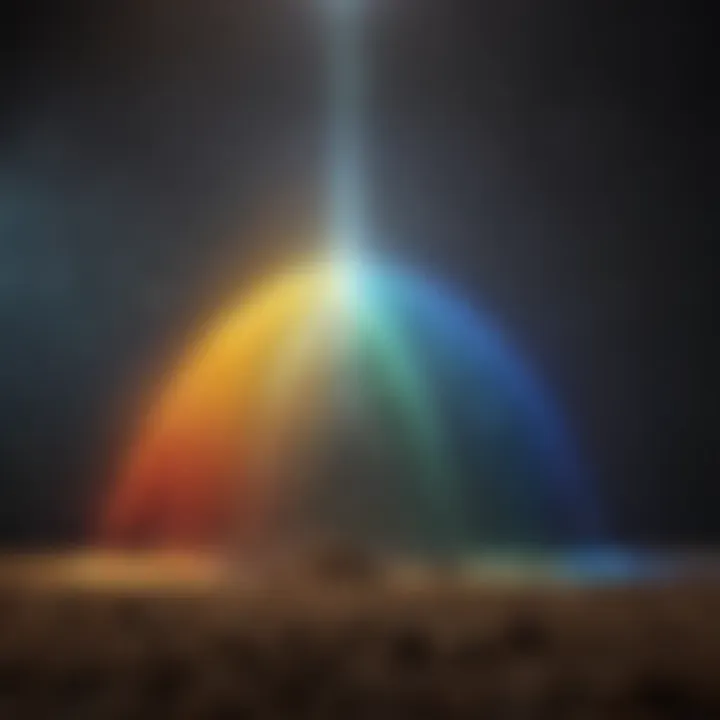
Intro
Electromagnetic radiation is a fascinating phenomenon that saturates our universe. It envelopes everything from the radio waves that play your favorite tunes to the gamma rays that hint at the most violent events in the cosmos. Recognizing its properties is crucial, as the implications stretch beyond mere scientific curiosity. By understanding the nuances of electromagnetic radiation, we can appreciate its role in areas like communication, medicine, astronomy, and beyond.
This article sets the stage to explore the fundamentals of electromagnetic radiation, diving into its origins, the spectrum it encompasses, and its multifaceted applications. It endeavors to dissect complex theories and present them in a way that anyoneβfrom students to seasoned researchersβcan grasp. Whether one is tuning in to the radio or gazing at stars through a telescope, the underlying principles of electromagnetic radiation are always at play.
Preamble to Electromagnetic Radiation
Electromagnetic radiation plays a pivotal role in both our everyday lives and the broader scientific landscape. It encompasses a range of phenomena that can shape technology, influence health, and even allow us to understand the universe at large. This section aims to shed light on the significance of electromagnetic radiation as a foundation for the topics that follow in this article.
One key aspect of electromagnetic radiation is that it occurs in various forms, each with distinct properties and behaviors. From radio waves, which can travel long distances, to gamma rays, with their high energy and penetrating power, understanding this range is crucial. How we define these forms not only helps us categorize the radiation but also allows us to harness it for various applications.
Moreover, this knowledge is instrumental in fields as varied as telecommunications, medical imaging, and environmental monitoring. For instance, the very devices we depend on, like smartphones and microwaves, operate based on principles of electromagnetic waves. Recognizing this connection bridges the gap between abstract science and everyday technology.
Understanding electromagnetic radiation also encourages awareness of its implications. As we delve into its nuances, we uncover both its benefits and potential health implications. This awareness is vital not just for scientists and professionals but also for the general public, who increasingly interact with various types of radiation in their daily lives.
In summary, this opening section sets the stage for an in-depth exploration. By unpacking the complexities of electromagnetic radiation, we prepare to delve into more technical principles and their practical applications. This journey will provide a comprehensive understanding of how these phenomena affect our world today.
Defining Electromagnetic Radiation
Electromagnetic radiation can be defined as a form of energy that travels through space at the speed of light. This energy moves in waves, which can be described in terms of wavelength and frequency. Waves are an essential concept, as the properties of these wavelengths dictate how electromagnetic radiation interacts with matter and the environment.
The electromagnetic spectrum embodies this idea, encompassing all types of electromagnetic radiation from low-frequency radio waves to high-frequency gamma rays. Each type carries different energy levels, influencing how they behave and what applications they find useful. For example, the long wavelengths associated with radio waves permit them to traverse great distances, making them ideal for communication.
Historical Background
The journey of understanding electromagnetic radiation is rich and often fascinating. It starts with early investigations in the 19th century when scientists like James Clerk Maxwell laid the groundwork with his famous equations. Maxwell demonstrated that electric and magnetic fields could propagate through space as waves. This was a groundbreaking insight, vastly expanding our understanding of physics.
Further developments came with Heinrich Hertz, who was the first to conclusively prove the existence of electromagnetic waves in the late 1800s. His experiments led to the realization that waves could carry energy without needing a medium, upending earlier classical physics models.
As the 20th century unfolded, the relationship between electromagnetic radiation and modern technology deepened. The advent of wireless communication, notably radio and television, demonstrated practical applications. Todayβs smartphones and internet rely heavily on this technology, showcasing how foundational these scientific principles have become in our lives.
In retrospect, tracing the historical context of electromagnetic radiation not only enriches our understanding but also highlights the cumulative nature of scientific knowledge. Each contribution builds upon previous discoveries, illustrating the collaborative innovation that characterizes scientific endeavor.
Fundamental Principles of Electromagnetic Waves
Understanding the fundamental principles of electromagnetic waves is pivotal to comprehending how they influence both our everyday lives and various technologies. These principles provide a foundation for numerous applications, ranging from telecommunications to medical imaging. By grasping the essence of these waves, readers can appreciate their role in modern science and technology, which ultimately drives many innovations today.
Wave-Particle Duality
The concept of wave-particle duality lies at the heart of quantum mechanics and presents a framework for understanding electromagnetic radiation in a multifaceted way. It posits that all particles exhibit both wave-like and particle-like properties, depending on the context of the observation. This duality is essential in grasping how light and other forms of electromagnetic radiation behave in different scenarios.
For instance, when discussing light as a wave, one can visualize how it travels in waves, allowing phenomena such as interference and diffraction. These behaviors explain why we see colorful patterns when light passes through prisms or why sound waves can create echoes in a canyon.
On the flip side, when light is treated as a particle, or a photon, we begin to understand phenomena like the photoelectric effect, which was pivotal in shaping our comprehension of energy transfer at molecular levels. It illustrates how light can knock electrons off metals, demonstrating that energy can behave like particles. This duality is not merely of academic interest; it's foundational to many technologies today, including semiconductors and lasers.
"The dual nature of light is both bewildering and awe-inspiring; it reveals the complex fabric of reality that governs our universe."
Maxwell's Equations
James Clerk Maxwell formulated a set of four equations that describe the behavior of electric and magnetic fields and their interaction with matter. Often hailed as one of the cornerstones of classical physics, Maxwell's equations provide a comprehensive framework for understanding electromagnetic phenomena. Each equation addresses distinct aspects of how electric fields generate magnetic fields and vice versa.
- Gauss's Law: This equation illustrates how electric charges produce electric fields, explaining how the field behaves in space.
- Gauss's Law for Magnetism: It indicates that there are no 'magnetic charges' analogous to electric chargesβmagnetic field lines are continuous loops.
- Faraday's Law of Induction: This equation explains how a changing magnetic field generates an electric field, a principle that underpins many technologies, including electric generators.
- Ampere-Maxwell Law: It describes how electric currents and changing electric fields produce magnetic fields, tying together the relationship between electricity and magnetism.
Maxwellβs equations do more than act as theoretical constructs; they have practical implications in designing technologies like wireless communication systems, radar, and even fiber optics. The precision these equations offer allows for advancements in various fields, including telecommunications and radiation science, further emphasizing their importance in understanding electromagnetic waves and their applications.
The Electromagnetic Spectrum
Understanding the electromagnetic spectrum is central to grasping the broader principles of electromagnetic radiation. This spectrum encompasses a vast range of wavelengths and frequencies, each possessing unique characteristics and applications. By breaking it down, one can appreciate the significance of each region in various scientific and technological contexts. Moreover, recognizing how these regions intersect with everyday life emphasizes the importance of this topic in both academic and real-world scenarios.
Overview of the Spectrum
The electromagnetic spectrum can be defined as the entirety of electromagnetic radiation arranged by frequency or wavelength. This includes all forms of radiation, from extremely low-frequency radio waves to high-frequency gamma rays. Each category within the spectrum offers distinct behaviors regarding energy, penetration abilities, and interaction with matter.
In essence, hereβs what makes this spectrum so monumental:
- Diversity of Applications: From medical imaging to communications, different segments of the spectrum cater to diverse uses.
- Safety Considerations: Understanding which parts of the spectrum are harmful can inform safe practices and technology use.
- Scientific Insights: Research into various electromagnetic waves leads to enhanced understanding in fields like physics, biology, and environmental science.
Regions of the Spectrum
Radio Waves
Radio waves sit at the lower end of the electromagnetic spectrum, characterized by their long wavelengths. They are widely utilized in communication technologies, including radio and television broadcasting. Their ability to travel long distances makes them invaluable. The primary benefit ofradio waves is their efficiency in transmitting information over vast distances without needing complex infrastructure.
The unique feature of radio waves lies in their reflection and refraction capabilities. These properties allow for signals to bounce off various surfaces, delivering reliable communication even in challenging environments. One disadvantage, though, is their relatively low energy compared to other radiation forms, which can limit certain applications.
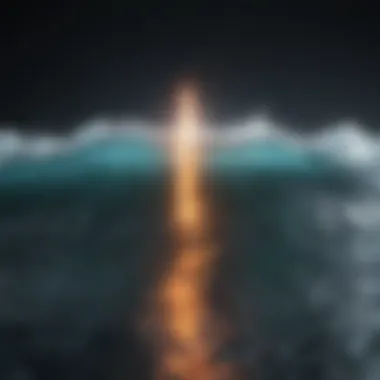
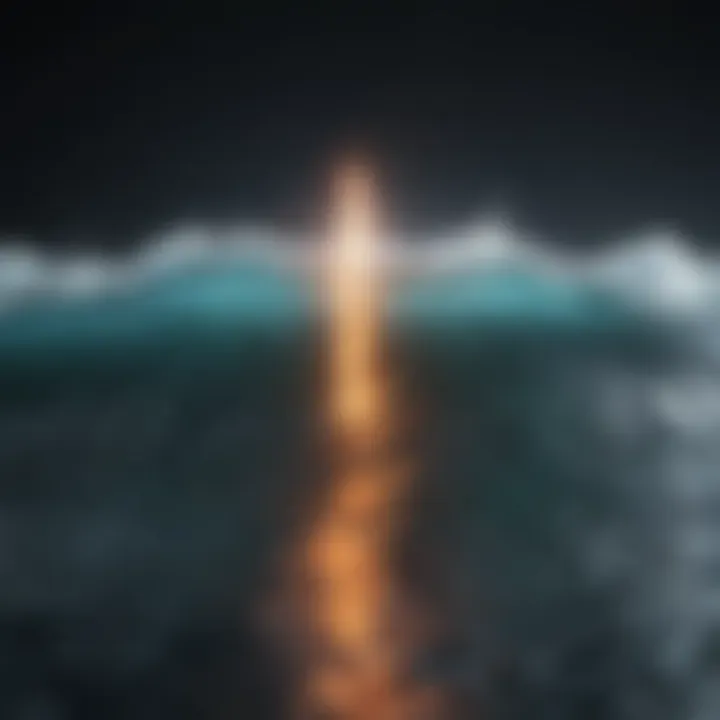
Microwaves
Next are microwaves, slightly shorter than radio waves. They play a crucial role in cooking food and in telecommunications. Microwaves have the unique ability to penetrate many materials; they heat food by agitating water molecules. This characteristic makes them efficient for cooking, which is compact and energy-saving.
Microwaves also facilitate communication technologies, such as satellite transmissions and Wi-Fi networks, making them tremendously useful in our interconnected world. Their downside, however, is that they can create interference with other devices, leading to potential communication disruptions in congested networks.
Infrared Radiation
Infrared radiation is positioned just above microwaves in terms of frequency. It is primarily associated with heat, making it essential for thermal imaging technologies and remote control devices. One of its notable features is its ability to detect temperature variations, allowing it to play a vital role in various thermal applications.
This type of radiation finds usefulness in security systems and night-vision technologies, enabling visibility in dark environments. Although advantageous, infrareds can be absorbed by clouds and dust, limiting their efficiency in certain atmospheric conditions.
Visible Light
Visible light is the part of the spectrum that human eyes can detect. It encompasses all the colors visible in the rainbow and is essential for various processes, such as photosynthesis in plants. The uniqueness of visible light lies in its relationship with life on Earth as it fuels the energy cycle.
While it is crucial for daily life and functioning, visible light's range can affect how it is studied and utilized. For instance, too much exposure to intense visible light can harm both humans and electronic devices, hence the importance of controlling light conditions in various environments.
Ultraviolet Radiation
Ultraviolet (UV) radiation spans just above visible light in the spectrum and has applications that include sterilization and medical treatments. UV rays can eliminate bacteria and viruses, specifically making it beneficial in water purification and medical environments.
A distinguishing feature is their ability to penetrate the first layer of human skin, which is vital in medical diagnostics and treatments. However, excessive exposure to ultraviolet light can lead to skin damage and increase the risk of skin cancer, highlighting the need for moderation in its applications.
X-rays
X-rays are another essential part of the spectrum, famous for their use in medical imaging. Their ability to pass through soft tissue but not bones makes them invaluable in diagnosing fractures and various medical conditions.
X-rays can reveal essential information inside the body without invasive procedures, making them a cornerstone in modern medicine. A downside is the concern around exposure to radiation, necessitating protective measures to minimize risks associated with repeated usage.
Gamma Rays
Lastly, gamma rays are at the extreme end of the spectrum, characterized by their high energy and penetrating power. They are pivotal in cancer treatment, particularly in radiation therapy, where they target tumor cells directly. The key characteristic of gamma rays is their ability to kill malignant cells or shrink tumors, providing significant benefits in oncological therapies.
However, the high energy of gamma rays also raises concerns regarding radiation exposure, making it vital to handle them with caution in medical settings and beyond.
Overall, understanding the different regions of the electromagnetic spectrum enriches our comprehension of its extensive applications and implications in both science and technology.
Interaction of Electromagnetic Radiation with Matter
The interaction between electromagnetic radiation and matter revolves around the innate responses of material substances to different types of radiation. These interactions serve as cornerstones for many scientific disciplines, including physics, chemistry, and biology. Understanding how electromagnetic waves behave when they encounter various materials is vital for applications ranging from medical imaging to telecommunications.
This section will delve into three major processes: reflection and refraction, absorption and transmission, and scattering effects. Grasping these concepts isn't just about understanding the mechanics; itβs about recognizing their significance in practical applications and how they affect our daily lives.
Reflection and Refraction
Reflection and refraction are two fundamental phenomena when electromagnetic waves hit the surface of materials. When a wave strikes a boundary between two media, part of the wave reflects back, and part refracts, or bends, as it enters the new medium.
Reflection happens when electromagnetic waves bounce back from a surface. This is evident when you look into a mirror; that's light reflecting off a smooth surface. Refraction is why objects seem bent or distorted when viewed underwater. It occurs due to the change in speed of waves when they move from one medium to another, such as air to water.
These processes are crucial in numerous applications:
- Optical Devices: Lenses in glasses or cameras depend on refraction to focus light correctly.
- Fiber Optics: The technology used in high-speed internet relies on total internal reflection to carry light signals over long distances.
Reflection and refraction also possess implications in fields like meteorology, where understanding how light bends can affect weather predictions.
Absorption and Transmission
Absorption involves the uptake of electromagnetic energy by matter, converting it into other forms, like thermal energy. When you expose a black shirt to sunlight, it absorbs more energy than a white shirt, illustrating how color and material properties influence absorption.
Transmission, on the other hand, refers to the passage of electromagnetic waves through a material. Different materials have varying capacities for transmitting radiation. For instance, glass allows visible light to pass through while blocking ultraviolet rays.
These principles bring about significant benefits, such as:
- Solar Energy: Solar panels convert sunlight into electricity through the absorption of electromagnetic radiation.
- Medical Treatments: Techniques like phototherapy utilize specific wavelengths of light for healing purposes.
Understanding how materials absorb and transmit electromagnetic radiation helps in designing efficient materials for various applications.
Scattering Effects
Scattering occurs when electromagnetic radiation interacts with small particles within a medium, causing the light to deviate from its original path. This phenomenon explains why the sky is blue. The shorter blue wavelengths are scattered more than the longer red wavelengths as sunlight passes through the Earth's atmosphere.
Scattering has practical implications and applications, including:
- Remote Sensing: Satellites rely on scattering data to analyze atmospheric conditions and land use.
- Medical Diagnostics: In techniques like laser scattering, examining dispersed light can yield information about cellular structures and their conditions.
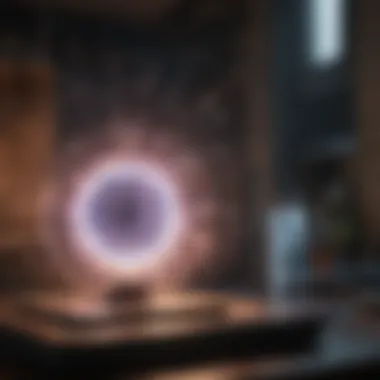
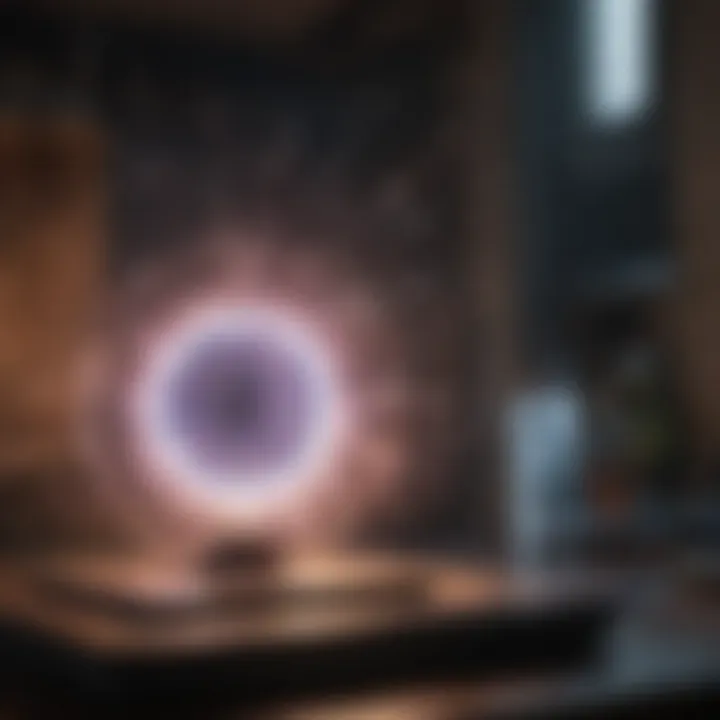
Integrating the knowledge of how electromagnetic radiation interacts with matter brings insight into a multitude of applications, guiding innovations ranging from environmental science to technology and health.
"Understanding these interactions is crucial, as they underpin many technologies and natural phenomena we take for granted every day."
Advancements in these fields promise not only to enhance existing technologies but also to pave the way for future innovations.
Technological Applications of Electromagnetic Radiation
The realm of technological applications of electromagnetic radiation is expansive. This section highlights its critical role across various fields, emphasizing the underlying principles at play as well as the wide-reaching benefits and considerations associated with these technologies. From facilitating instant communication to enabling life-saving medical diagnostics, the implications are profound, pushing the boundaries of innovation.
Communication Technologies
Electromagnetic radiation serves as the backbone of modern communication systems. The use of radio waves, microwaves, and optical signals has revolutionized how humans interact, both on personal and global scales.
- Radio Waves: These are paramount in broadcasting, allowing for mass communication through radio and television. Radio waves also underlie technologies like Wi-Fi and Bluetooth, connecting devices wirelessly.
- Microwaves: These waves are instrumental in radar technology and satellite communications, ensuring that data transmission is both swift and reliable.
- Optical Signals: Fiber-optic communication has emerged as a game changer, enabling vast amounts of data to be transmitted across the globe in real-time, without any significant loss of quality.
The efficiency and capabilities of communication technology continue to expand, allowing for innovations such as 5G networks that promise faster speeds and enhanced connectivity. This interconnectedness brings along considerations regarding security and bandwidth availability, though, making it an essential topic for ongoing research and development.
Medical Imaging
In the medical field, electromagnetic radiation has transformed diagnostics and treatment procedures. Techniques such as X-ray, MRI, and ultrasound imaging have proven invaluable for health professionals.
- X-rays: Widely utilized for examining bone fractures and other internal abnormalities; they provide quick visualizations, aiding in prompt decision-making.
- MRI (Magnetic Resonance Imaging): This uses radio waves and magnetic fields to create detailed images of organs and tissues, improving diagnostic accuracy significantly.
- Ultrasound: Relying on high-frequency sound waves, this method is commonly used for monitoring fetal development during pregnancy.
These technologies not only enhance our understanding of human anatomy but also improve patient outcomes through more accurate diagnosis and treatment. However, the health implications tied to exposure, even at low levels, remain a topic of active inquiry. Awareness of potential risks is invaluable in developing safer protocols for patient care.
Energy Generation
Electromagnetic radiation has found a foothold in energy generation, particularly in renewable energy sources. The use of solar panels, for instance, taps into the energy produced by the sun in a manner that contributes to sustainable development.
- Solar Power: Photovoltaic cells capture sunlight and convert it into electricity, providing a clean, renewable resource for countless households and industries.
- Thermal Energy: This method employs radiation to heat water or air, generating heat energy that can be harnessed for heating systems.
The transition toward utilizing electromagnetic radiation for energy generation offers significant benefits, not only by reducing reliance on fossil fuels but also by addressing environmental concerns. Yet, considerations regarding the efficiency of these technologies and the materials used in their production should not be overlooked, ensuring that advancements do not inadvertently harm ecological systems.
"The advancement of technology is the direct result of understanding and applying the principles of electromagnetic radiation, shaping how we live, communicate, and work."
The applications of electromagnetic radiation span industries, playing a pivotal role in daily life and cutting-edge innovations. As we further explore these applications, acknowledging both their benefits and associated risks can guide responsible usage and development.
Electromagnetic Radiation in Scientific Research
Electromagnetic radiation (EMR) plays a pivotal role in scientific investigation, acting as both a tool and a subject of inquiry. Understanding this form of radiation is essential for advancing knowledge across various fields, including physics, chemistry, and environmental science. By harnessing EMR, researchers can manipulate and analyze matter at a fundamental level, which provides insights that are often unattainable through traditional techniques.
In this section, we will explore two main areas where electromagnetic radiation proves indispensable: analytical techniques and environmental monitoring. Each topic not only highlights the significance of EMR in science but also demonstrates the wide range of applications that stem from this understanding.
Analytical Techniques
Analyzing materials and phenomena through the lens of electromagnetic radiation opens doors to answers that can drive innovation. Two prominent analytical techniques that rely on electromagnetic radiation are spectroscopy and microscopy.
Spectroscopy
Spectroscopy is a method that explores the interaction between electromagnetic radiation and matter. By examining how materials absorb, emit, or scatter light, scientists can glean information about the composition and properties of substances. The key characteristic of spectroscopy lies in its ability to identify chemical structures through spectral analysis, making it a popular choice in chemical and material studies.
One unique feature of spectroscopy is its versatility; it encompasses various forms, such as infrared spectroscopy, ultraviolet-visible spectroscopy, and nuclear magnetic resonance (NMR) spectroscopy. Each type holds its own advantages and disadvantages. For example:
- Advantages:
- Disadvantages:
- Non-destructive analysis of samples.
- Ability to provide detailed information about molecular structures.
- Requires precise instrumentation that can be costly.
- Interpretation of spectra can demand high levels of expertise.
"Spectroscopy offers a window into the molecular world, helping researchers transform obscure data into actionable insights."
Microscopy
Microscopy, on the other hand, uses electromagnetic radiation to magnify small objects, allowing scientists to observe them in fine detail. Microscopes employ various forms of EMR, from visible light to X-rays, enabling observations that span a broad range of scales. The key characteristic of microscopy is its capability to reveal structures that are beyond the reach of the naked eye.
One major advantage of microscopy is the improved visualization of biological specimens or materials at the atomic level. Techniques such as electron microscopy can achieve resolutions that are exceptionally high, providing unprecedented insights into the structural organization of matter. However, disadvantages include:
- Advantages:
- Disadvantages:
- Ability to examine live cells or materials in near-real-time.
- Advanced techniques reveal intricate details of structures.
- Preparation of samples can alter their native state.
- High equipment costs can be a barrier for some institutions.
Environmental Monitoring
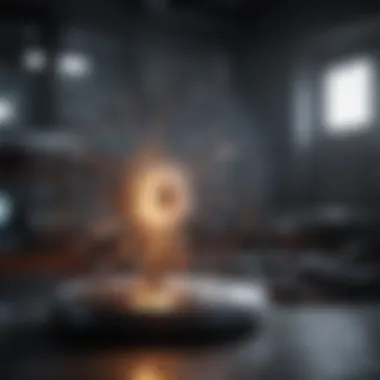
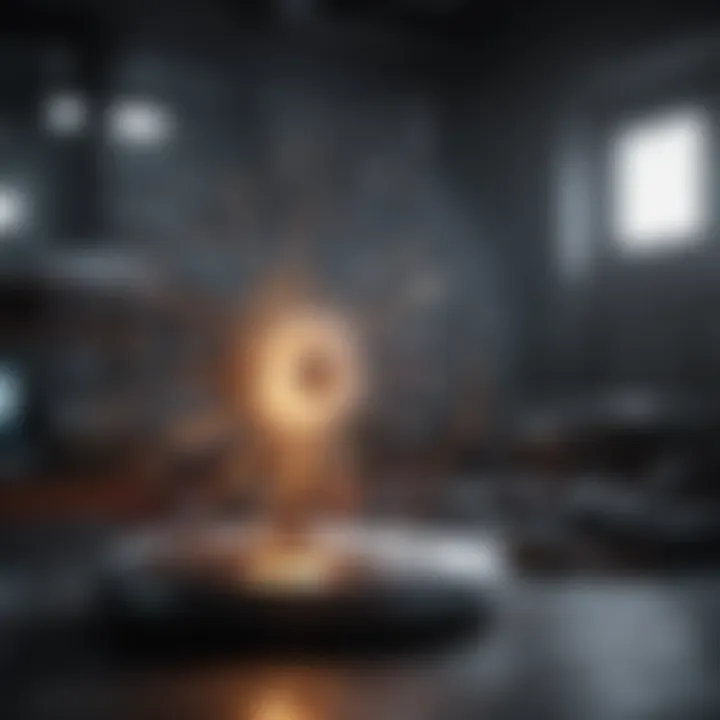
Environmental monitoring is another crucial area where electromagnetic radiation is applied effectively. By using remote sensing techniques, researchers can assess environmental conditions over time. This approach employs a variety of electromagnetic spectrum sections to measure variables like temperature, humidity, vegetation indices, and even pollutants in the atmosphere.
The benefits of employing EMR in environmental monitoring are manifold:
- Non-invasive assessments:
- Data collection from remote locations:
- EMR allows for large-scale surveys of ecosystems without direct interference.
- Satellites equipped with sensors capture crucial data from hard-to-reach areas, such as forests or oceans.
These capabilities not only enhance our understanding of environmental dynamics but also support efforts in conservation and disaster management. Overall, the role of electromagnetic radiation in scientific research is vital, enabling diverse applications that benefit society and the scientific community alike.
Health Implications of Electromagnetic Radiation
Understanding the health implications of electromagnetic radiation is crucial in todayβs tech-driven world. As we become increasingly reliant on electromagnetic technologies β whether itβs our smartphones, microwaves, or Wi-Fi signals β the associated health risks and safety standards also need careful examination. Itβs not just a question of technology; itβs about how these advancements affect our well-being and lifestyle.
Radiation Safety
When we talk about radiation safety, we are looking at the guidelines, measures, and protocols in place to protect individuals from the potential harmful effects of electromagnetic radiation. Regulatory bodies like the Federal Communications Commission (FCC) and the World Health Organization (WHO) establish safety limits based on research and studies. These limits aim to prevent thermal effects (where tissue gets hot) and non-thermal effects that might cause biological changes yet to be fully understood.
Itβs essential to highlight some key points regarding radiation safety:
- Maximizing Distance β Keeping a safe distance from sources of electromagnetic radiation is often advised. For example, avoiding prolonged mobile phone calls or using speaker mode can reduce exposure.
- Usage of Shields and Barriers β In certain industries, using shielding materials can help mitigate the exposure levels. This safeguard is common in places where diagnostic imaging occurs.
- Monitoring Technologies β Personal devices and tools can measure exposure levels, allowing individuals to make informed choices about technology use in certain settings.
"Awareness and awareness alone can transform interaction with technology and reduce unwarranted risks."
Understanding these safety aspects not only empowers individuals but also fosters a greater sense of responsibility in the development and use of technology.
Electromagnetic Hypersensitivity
Electromagnetic hypersensitivity (EHS) is a condition that some individuals report where they claim to experience adverse reactions to electromagnetic fields, such as headaches, fatigue, and even stress. While the scientific community remains divided on whether EHS is a medically recognized condition, it has sparked considerable debate and research into the effects of low-level electromagnetic radiation.
Some considerations in this area include:
- Subjectivity of Symptoms β Many who identify as suffering from EHS report symptoms that vary widely, leaving the root cause not solely attributed to electromagnetic radiation. Psychological factors may play a large role.
- Lack of Scientific Consensus β No definitive connection has been established through clinical studies, leading organizations like the WHO to state that, while symptoms are real for those affected, further research is needed to understand the mechanisms behind them.
- Personal Choice and Environment β For affected individuals, the choice to limit exposure can lead to a less stressful lifestyle, whether through reduced screen time or opting for wired connections instead of wireless.
As a society, exploring these health implications raises vital questions about how we balance technological advancement with personal well-being. The ongoing dialogue surrounding these topics is essential for educating the public and guiding research in the right direction.
Future Directions in Electromagnetic Radiation Research
Research in electromagnetic radiation is a continuously evolving field, tightly intertwined with advancements in technology and science. The future of this discipline holds immense promise, with emerging developments across several sectors promising to enhance not just our understanding but also our capability to harness electromagnetic radiation for societal good. The implications of emerging technologies and interdisciplinary approaches are particularly noteworthy, as they pave the path for innovative solutions to existing challenges while furthering scientific inquiry.
Emerging Technologies
In the realm of emerging technologies, several trends are pointing towards a transformative future. For instance, the development of terahertz technology stands out. This technology, operating in the frequency between microwaves and infrared radiation, has potential applications in medical diagnostics and security screening.
Another noteworthy advancement is the rise of quantum communication, which employs the principles of quantum mechanics to secure data transmission. Such technologies promise not only enhanced privacy but also the potential for unprecedented speed in communication systems.
Moreover, advancements in materials science are leading to the creation of novel photonic materials. These materials can manipulate light in ways previously deemed impossible, opening avenues for more efficient solar panels and improved LED technology. Here are a few key branches where these technologies can leave a significant mark:
- Telecommunications: Higher bandwidth and better signal integrity.
- Healthcare: Enhanced imaging techniques and treatments.
- Renewable Energy: More effective capture and conversion of sunlight.
Interdisciplinary Approaches
The complexity of electromagnetic radiation research necessitates collaboration across various scientific disciplines. By merging expertise from physics, engineering, biology, and environmental sciences, we can tackle multifaceted challenges.
For example, researchers investigating the impact of electromagnetic fields on living organisms are not only relying on physics but also drawing insights from biology and environmental science. This collaborative effort helps in understanding electromagnetic hypersensitivity, leading to better safety standards and health recommendations.
In another area, the integration of computer science with traditional electromagnetic research is leading to enhanced modeling techniques. Machine learning algorithms can analyze vast data sets to identify patterns and predict behaviors of electromagnetic waves in different media. This synthesis of fields generates innovative solutions that weren't achievable individually. Here are some areas where interdisciplinary approaches shine:
- Environmental Monitoring: Using remote sensing combined with atmospheric science to predict weather patterns.
- Medical Research: Collaborating with biology to better understand how radiation interacts with tissue.
The future of electromagnetic radiation research is not singular; it is an intricate web of technologies and disciplines, working together to address humanity's greatest challenges.
As we embrace these future directions, it is essential to remain mindful of ethical considerations, especially regarding health implications and environmental impact. Continuous exploration and innovation, coupled with responsible application of findings, will underpin advancements for years to come.
Ending
In this article, we have journeyed through the fascinating landscape of electromagnetic radiation, shedding light on its myriad principles and vast implications. This conclusion serves not merely as a wrap-up but as a reflection on the significance of what we've explored.
Summary of Key Points
Electromagnetic radiation manifests in various forms, each with unique characteristics and behaviors. Here are the key points we discussed:
- Wave-Particle Duality: The behavior of light and radiation as both waves and particles helps us understand its complex nature.
- Maxwell's Equations: These equations form the foundation of electromagnetic theory, tying together electric and magnetic fields.
- Electromagnetic Spectrum: This spectrum encompasses all types of radiation, from radio waves to gamma rays, each with distinct uses and effects.
- Interactions with Matter: The ways electromagnetic radiation interacts with matter through reflection, absorption, and scattering inform its applications in technology and science.
- Technological Applications: The advancements in communication, medical imaging, and energy generation stem from our understanding of radiation principles.
- Health Implications: Understanding the safety concerns and potential hypersensitivity to electromagnetic radiation is crucial for public health.
- Future Directions: Emerging technologies and an interdisciplinary approach show promise for continuing research and innovation in this field.
Each of these points reinforces the critical role that electromagnetic radiation plays not only in technology but in sustaining life and expanding our knowledge of the universe.
Final Thoughts
As we reflect on the principles of electromagnetic radiation, it is imperative to recognize the balance between its benefits and potential risks. The ongoing research in this field opens doors to new technologies while posing significant questions regarding human health and environmental impact. For students, researchers, educators, and professionals, diving deeper into electromagnetic radiation may yield extensive insights, paving the way for future inventions and discoveries. With the world increasingly reliant on electromagnetic technologies, the importance of understanding these concepts becomes ever more crucial. Knowledge in this domain empowers us to innovate responsibly, ensuring that we harness the power of electromagnetic radiation for a future that benefits all.