RNases in Molecular Biology: Roles and Mechanisms
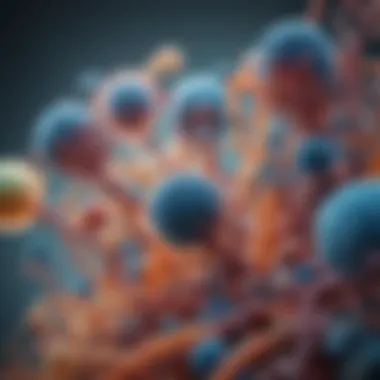
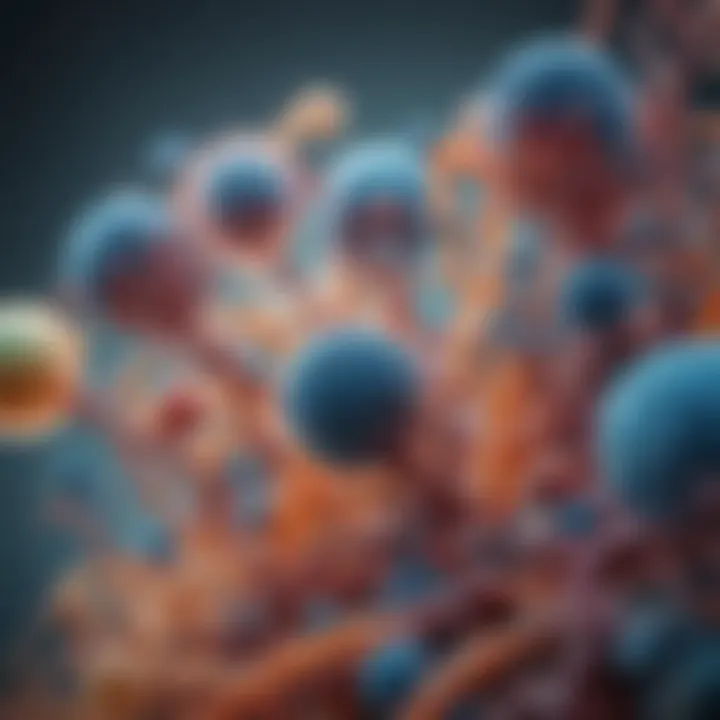
Intro
Ribonucleases, commonly known as RNases, are vital enzymes in the realm of molecular biology. They are responsible for the degradation of RNA, a process crucial for various cellular functions such as RNA metabolism, regulation of gene expression, and the maintenance of RNA homeostasis. In this exploration, we will delve into the classification, mechanisms, and significance of RNases, shedding light on their multifaceted roles in both normal physiological processes and pathological conditions.
Understanding RNases not only enhances our grasp of fundamental biological mechanisms but also opens doors to biotechnological advances and therapeutic interventions. This article aims to provide a comprehensive guide to the role and functionality of RNases in molecular biology, addressing their biochemical properties, interactions, and implications in disease and biotechnological applications.
Preface to RNases
In molecular biology, RNases, or ribonucleases, are essential enzymes that play a pivotal role in the metabolism and regulation of RNA. Understanding RNases facilitates insight into fundamental biological processes, ranging from RNA processing to degradation. The importance of RNases cannot be overstated, as they contribute significantly to the stability, functionality, and integrity of RNA molecules within cellular environments.
This section aims to provide a comprehensive introduction to RNases, emphasizing their definition, historical context, and the critical considerations surrounding their functions. By delving into these aspects, readers will gain a solid foundation for appreciating the subsequent discussions regarding different classifications, biochemical properties, and the broader implications of RNases in molecular biology.
Definition and Overview
RNases are specialized enzymes that catalyze the degradation of RNA molecules. By hydrolyzing the phosphodiester bonds within RNA strands, they contribute to various cellular functions. There are different types of RNases, categorized primarily based on their structure, mechanism of action, and substrate specificity. The two main categories are endoribonucleases, which cleave RNA at internal sites, and exoribonucleases, which remove nucleotides from the ends of RNA strands.
These enzymes are not only crucial for RNA turnover but also play a key role in RNA quality control, helping to regulate the levels of various RNA species in a cell. Consequently, their activity is integral to proper cellular function and homeostasis.
Historical Context
The exploration of RNases dates back to the early 20th century. The first comprehensive isolation of ribonuclease occurred in the 1930s, when researchers began to investigate the enzyme's chemical properties and potential functions. Advances in biochemical techniques paved the way for a deeper understanding of RNA itself, leading to the realization of the multifaceted roles that RNases hold in the cell.
As research progressed, scientists recognized not only the enzymatic activity of RNases but also their regulatory functions. This historical evolution has culminated in a robust understanding of RNases as integral components in RNA metabolism and cellular responses.
"The significance of RNases extends beyond mere RNA degradation; they are critical regulators of RNA life cycles and cellular homeostasis."
Overall, the study of RNases has become a cornerstone of molecular biology, affording insights into disease mechanisms, genetic regulation, and prospects for biotechnological applications.
Classification of RNases
The classification of RNases is critical in understanding their diverse roles in molecular biology. Recognizing the different types of RNases helps clarify their functions in RNA metabolism, as each class exhibits distinct biochemical characteristics and mechanisms of action. This classification not only aids in research and application but also in the development of therapeutic strategies targeting specific RNase activities. Each category of RNase performs unique tasks that are pivotal in processes such as RNA processing, quality control, and cellular responses. Careful delineation allows scientists to explore RNases with precision and adapt methodologies accordingly.
Endoribonucleases
Endoribonucleases are enzymes that cleave RNA molecules internally. They exhibit specificity for certain RNA substrates based on sequence or structural features, leading to important functional implications. For instance, these enzymes play a crucial role in the maturation of rRNA and tRNA. Their ability to generate smaller RNA fragments allows for more efficient processing and functioning of the ribonucleic acids. This subgroup is essential in various cellular processes, including the regulation of gene expression and the initiation of RNA degradation.
Exoribonucleases
Exoribonucleases operate by removing nucleotides from the ends of RNA molecules. They can function in both 5' to 3' and 3' to 5' directions, which influences RNA stability and degradation. This activity is essential for maintaining RNA homeostasis within the cell. Exoribonucleases are necessary for the trimming of aberrant RNA molecules, which underscores their role in quality control. Their activity also enables the recycling of RNA components, contributing to cellular economy.
Specific RNase Families
RNase A Family
The RNase A family is one of the most studied groups of ribonucleases. It is renowned for its ability to cleave RNA at specific sites, producing 2',3'-cyclic phosphates. This characteristic is particularly beneficial for research related to RNA structure and function. The widespread availability and accessibility of RNase A make it a popular choice in laboratories. However, a notable challenge is that it can lead to non-specific degradation under certain conditions, which researchers must consider for experimental design.
RNase H Family
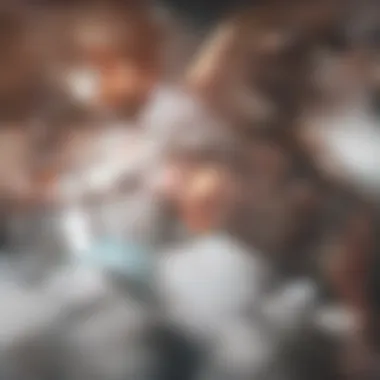
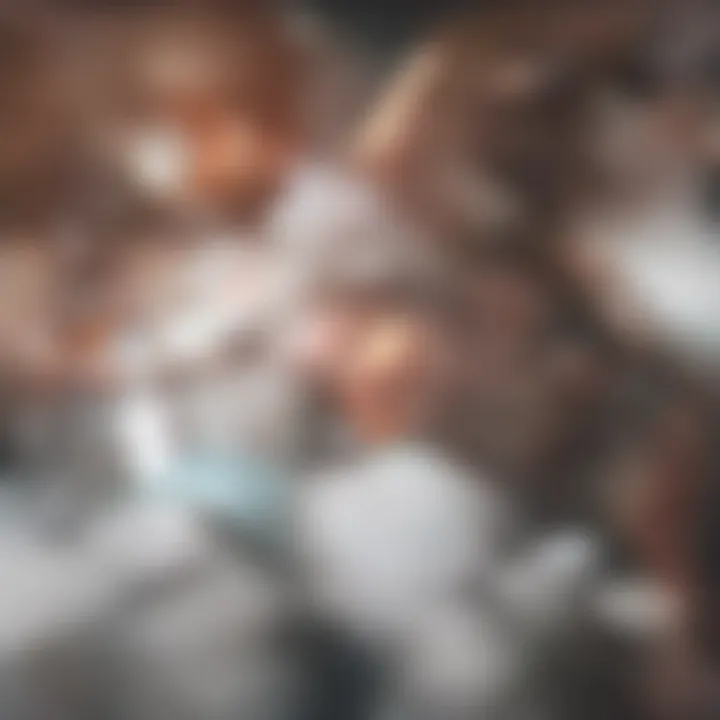
RNase H family members are distinguished by their ability to degrade RNA in RNA-DNA hybrids. This unique feature plays a pivotal role in various cellular processes, including DNA replication and repair. The capacity to recognize hybrid nucleic acids allows these RNases to be incredibly useful in biotechnology applications, such as in the development of tools for detecting specific nucleic acids. Nevertheless, the activity of RNase H can vary, making it crucial to carefully select the appropriate conditions during experiments to achieve desired outcomes.
RNase Family
The RNase III family is recognized for its involvement in the processing of double-stranded RNA. It facilitates the production of small interfering RNAs, which are fundamental in gene regulation. The specificity of RNase III for double-stranded RNA is a key advantage in research, particularly in studies involving RNA interference. However, its strong preference for these substrates can limit its application in scenarios involving single-stranded RNA, which researchers must account for in experimental protocols.
Biochemical Properties of RNases
The biochemical properties of ribonucleases (RNases) are fundamental in understanding their roles in molecular biology. These properties define how these enzymes interact with RNA molecules, dictate their functionality, and influence various cellular processes. An in-depth examination of these attributes provides insight into how RNases are essential in maintaining RNA homeostasis and how they contribute to cellular health.
Mechanism of Action
The mechanism of action of RNases is pivotal in grasping their functionality. RNases largely operate by hydrolyzing RNA strands at specific sites, effectively breaking the RNA molecule into smaller fragments. This action can occur through different pathways depending on the classification of the RNase. For example, endoribonucleases cleave within the RNA strand, leading to the production of smaller oligonucleotides, while exoribonucleases trim the RNA from its ends. Understanding these mechanisms is crucial for determining how RNases regulate RNA levels in the cell.
In the context of enzymatic activity, the catalytic mechanism involves essential components like metal ions and specific amino acid residues. These elements participate in stabilizing the transition state of the RNA during hydrolysis. Moreover, the recognition of substrate specificity varies among different RNase families, influenced by the structural conformation of the enzyme and environmental conditions.
Enzymatic Activity and Regulation
The enzymatic activity of RNases is tightly regulated to maintain cellular function. Various factors influence this regulation, including environmental signals, cellular stressors, and interaction with other proteins. Regulation can occur at multiple levels β from transcriptional control of RNase expression to post-translational modifications that alter the enzyme's activity or stability.
Several mechanisms contribute to the activity of RNases:
- Environmental Factors: Temperature and pH can significantly impact RNase activity. Each RNase has an optimal pH and temperature range where it functions most effectively.
- Substrate Availability: The concentration of RNA substrates influences RNase activity; a higher concentration can enhance enzymatic action.
- Inhibitors and Activators: Other molecules can inhibit or activate RNases, adjusting their activity in response to cellular needs. For instance, proteins that bind RNases may prevent them from interacting with their RNA substrates.
Understanding these regulatory mechanisms aids in unraveling the complex network of RNA metabolism. As researchers explore these aspects further, insights into how RNases contribute to disease pathways or therapeutic applications can be anticipated.
"The regulation of RNases is crucial not only for RNA maintenance but also for ensuring that cellular processes remain finely tuned."
Role of RNases in RNA Metabolism
The role of RNases in RNA metabolism is crucial, contributing significantly to the post-transcriptional control of gene expression. RNases are involved in various processes that ensure the proper management and regulation of RNA within the cell. Understanding these roles provides insight into both fundamental cellular mechanisms and their implications in health and disease. The activities of RNases help define the cellular RNA pool, maintaining balance and integrity in RNA structures.
RNA Processing
RNA processing is the modification of nascent RNA transcripts to produce the mature RNA forms necessary for translation or other functions. RNases play a central role here by catalyzing specific cleavage reactions. For example, endoribonucleases operate within the RNA strand, facilitating the removal of introns and generating correct exons. This step is vital for generating messenger RNA (mRNA) that can be translated into proteins.
Moreover, RNases like RNase III engage in the processing of precursor microRNAs (pre-miRNAs) into functional miRNA. This mechanism is fundamental as miRNAs are key regulators of gene expression through RNA interference pathways, impacting on numerous biological processes such as development, differentiation, and cell proliferation.
RNA Degradation
RNA degradation is an essential process that protects cells from the accumulation of defective or outdated RNA molecules. RNases are responsible for the targeted degradation of RNA, enabling the cell to recycle nucleotides and prevent erroneous protein synthesis. Exoribonucleases are particularly notable in this task, removing nucleotides from the ends of RNA molecules.
The regulated degradation of specific mRNAs is equally important, allowing for timely responses to cellular signals. For example, the decay of mRNA can influence the levels of proteins synthesized in response to stress or other stimuli. This process ensures that only the required proteins are available when needed.
Quality Control Mechanisms
Quality control mechanisms facilitated by RNases ensure the surveillance of RNA integrity within cells. For instance, if a ribosomal RNA (rRNA) molecule has an aberration, specific RNases can identify and degrade this faulty RNA. This surveillance prevents faulty ribosomes from assembly, which could lead to problematic protein synthesis.
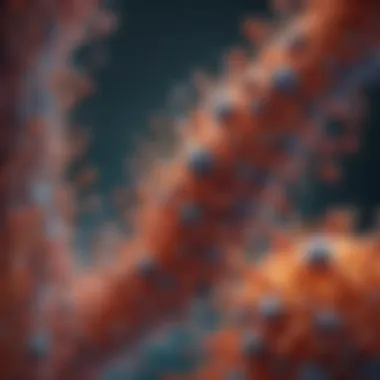
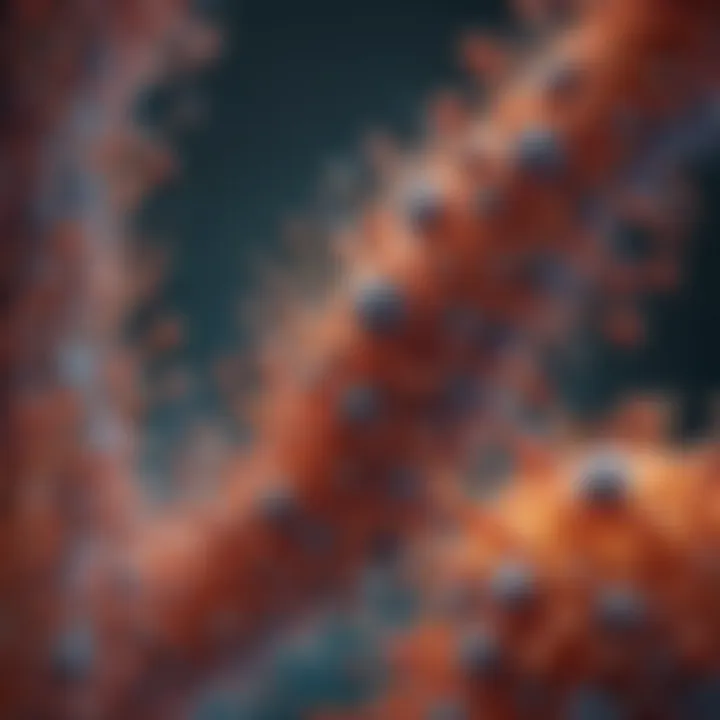
The RNA exosome complex, which includes several RNases, is vital for monitoring RNA quality. It recognizes and degrades defective RNA molecules.
This RNA quality control pathway maintains cellular homeostasis by removing non-functional RNAs, thereby upholding the overall health of the organism.
In summary, the roles of RNases in RNA metabolism encompass essential functions such as RNA processing, degradation, and quality control mechanisms. Understanding these processes illuminates the broader implications for molecular biology, with significant relevance to research areas ranging from genetics to therapeutic development.
RNases and Cellular Processes
The role of ribonucleases, or RNases, extends beyond simple RNA degradation. They are deeply intertwined with the way cells regulate various functions. Many of these processes play significant roles in ensuring cellular health and adaptation. Understanding these processes is crucial for researchers who seek to improve our grasp on how cells operate and react under different conditions.
Gene Regulation Activities
RNases are central to the regulation of gene expression. By controlling the levels of mRNA in a cell, they influence which proteins are produced and when. This regulation is pivotal for numerous cellular functions, including growth, differentiation, and response to environmental signals. For example, when a gene is activated, its respective mRNA is synthesized. Once produced, the RNases can either stabilize it or trigger its degradation.
Factors like microRNAs also play a role in regulating gene expression through RNases. MicroRNAs can bind to specific mRNA molecules, leading to their degradation through RNase activity. This mechanism ensures fine-tuning of protein levels within the cell, which is essential for maintaining homeostasis.
"Understanding RNase functions in gene regulation provides insights into various biological processes and potential therapeutic targets in diseases."
Role in Cellular Stress Responses
RNases also have prominent roles in how cells respond to stress. Various stress conditions, such as oxidative stress or nutrient deprivation, can lead to the accumulation of unneeded or damaged RNA molecules. RNases facilitate the rapid turnover of these RNA species, effectively preventing cellular damage and maintaining the integrity of the transcriptome.
In stressful situations, the expression of certain RNases can be upregulated. This adaptive mechanism allows cells to selectively degrade mRNA that are no longer needed while preserving essential transcripts. This balance is crucial for the survival of the cell under adverse conditions.
Research has shown that manipulating RNase activities could enhance stress responses or mitigate pathological effects in various diseases, such as cancer or neurodegeneration.
Through these roles, RNases serve not just as enzymes for RNA turnover, but as key players in the intricate network of cellular processes, influencing both normal physiology and responses to environmental challenges.
This exploration of RNases and their functions is imperative for understanding cellular behavior in a dynamic environment.
Implications of RNase Functionality
The functionality of ribonucleases (RNases) is significant for various reasons. This section explores these implications, specifically in pathological considerations and therapeutic applications. Recognizing the influence of RNases extends our understanding of cellular processes and offers ways to address both disease and treatment.
RNases do not only contribute to RNA metabolism but also directly impact health and disease. For instance, aberrations in RNase activity can lead to inappropriate RNA processing, which may result in the development of various diseases, including cancers and neurodegenerative disorders. On the therapeutic side, targeting RNase activity can provide novel approaches for treatments, creating opportunities for interventions in previously difficult-to-treat conditions. The study of RNases is not merely academic; it has practical consequences that can benefit medicine and biotechnology.
"Understanding RNase functionality opens pathways for innovative therapies and precise diagnostic tools in molecular biology."
In summary, the implications of RNase functionality are profound and span biological, medical, and technological domains. The effects on physiological and pathological processes highlight the necessity of further exploration in diverse contexts.
Pathological Considerations
Pathological implications of RNase functionality primarily relate to the association of various RNases with diseases. Disturbances in the expression or activity of specific RNases can lead to inadequate RNA processing and accumulation of inappropriate RNA fragments. This is especially true in conditions such as:
- Cancers: Certain RNases, when overexpressed or mutated, are implicated in tumor growth. For instance, RNase L has been linked to the regulation of apoptosis and its dysregulation can contribute to cellular proliferation often seen in cancers.
- Neurodegenerative Diseases: RNase-related dysfunctions can disrupt the balance of RNA degradation and synthesis, which is critical in maintaining neuronal health. Disorders such as Alzheimer's disease have shown associations with RNase involvement, where misregulated RNA turnover contributes to pathological features.
- Infectious Diseases: RNases also play roles in the immune response to viral infections. Alterations in RNase levels can influence the ability to combat viral RNA, affecting disease outcome.
Targeting these pathological pathways can yield potential strategies for intervention. Research continues to explore how manipulating RNase activity can reverse or prevent disease progression.
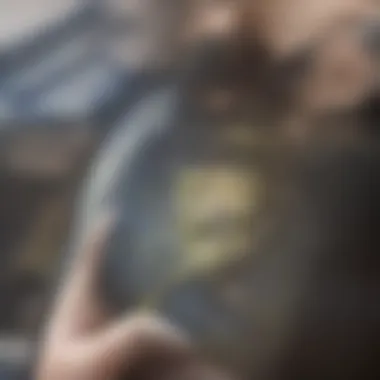
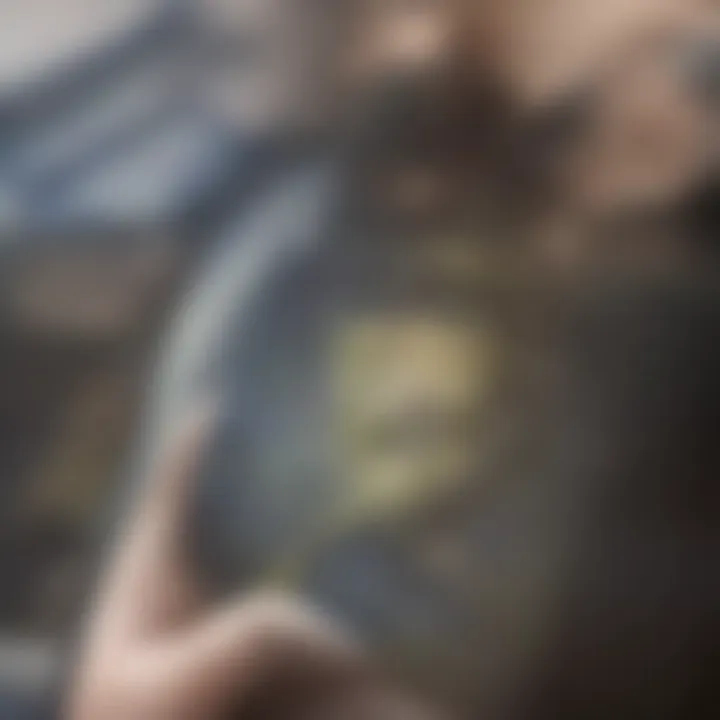
Therapeutic Applications
The therapeutic potential of RNases is gaining traction in molecular biology. By understanding how RNases function, researchers aim to harness this knowledge for medical applications. Some of the significant applications include:
- Targeted Cancer Therapies: The engineering of RNases, like the ribonuclease cytotoxic proteins, shows promise in selectively targeting tumor cells. These RNases can cause cell death in cancerous cells while sparing normal cells, enabling a more focused treatment approach.
- Antiviral Strategies: RNases are being explored as viral inhibitors. By degrading viral RNA, these enzymes can limit virus replication, offering a novel antiviral strategy to combat infections.
- Diagnostic Tools: The measuring of RNase levels can serve as a biomarker for certain diseases. For example, increased RNase levels can indicate inflammatory responses or malignancies, thus aiding in early detection.
Overall, the therapeutic applications of RNases provide a horizon full of possibilities. Their versatile role in both pathology and treatment underscores the importance of continued research and innovation in this field.
Advancements in RNase Research
Recent years have witnessed significant progress in understanding the diverse roles and functionalities of RNases in molecular biology. This advancement comes at a time when the demand for innovative therapeutic strategies and diagnostic tools continues to grow. The exploration of RNases has led to new insights that refine existing knowledge and raise intriguing questions about their application in various scientific fields.
Innovations in Biotechnology
The biotechnology landscape has benefited from new discoveries related to RNases. One major innovation is the development of ribonuclease-based therapies. These therapies aim to selectively target and degrade aberrant RNA molecules associated with diseases, such as cancer. For instance, the use of engineered RNases tailored to recognize specific RNA sequences allows for precise interventions. This targeted approach minimizes side effects often associated with traditional treatments, such as chemotherapy.
Moreover, the role of RNases in RNA interference (RNAi) has opened avenues for designing novel gene-silencing techniques. Researchers are exploring the potential of combining RNase activities with nanoparticles for enhanced delivery and specificity. This innovative strategy could foster the creation of sophisticated treatments for genetic disorders.
"The strategic manipulation of RNases holds great promise for advancing therapeutic options in molecular medicine."
Future Directions in RNase Studies
Looking ahead, the future of RNase research is poised for exciting developments. Ongoing studies are expected to clarify the intricate regulatory networks that govern RNase activity. A deeper understanding of these mechanisms can elucidate how RNases adapt in response to cellular changes and environmental stimuli.
In addition, researchers are likely to delve into the potential of RNases as biomarkers for diseases. The levels of specific RNases can vary significantly in different pathological states, making them candidates for diagnostic applications. Understanding their expression patterns could aid in early detection and monitoring of various conditions, particularly in oncology.
The integration of computational approaches with experimental biology offers another promising direction. By employing bioinformatics and machine learning, scientists can predict RNase interactions and functions based on large datasets. This can lead to identifying new RNase family members, as well as uncovering their roles in cellular processes.
As the field progresses, collaborative efforts among researchers, clinicians, and biotechnologists will be crucial in translating these scientific advancements into practical applications. The comprehensive understanding of RNases not only enhances our grasp of RNA biology but also lays the groundwork for future innovations in medical science and therapeutic development.
End
The examination of RNases in molecular biology underscores their multifaceted roles and physiological significance. This conclusion reaffirms the central themes discussed throughout the article, emphasizing how these enzymes are not merely bystanders but essential components in numerous cellular processes. Understanding the functionality of RNases allows scientists to appreciate their contributions to RNA metabolism, regulation of gene expression, and responses to cellular stress.
Summary of Key Insights
Numerous insights emerge from the analysis of RNases:
- Classification: RNases can be broadly classified into endoribonucleases and exoribonucleases, each with distinct mechanisms of action and roles in RNA metabolism.
- Biochemical Properties: The mechanisms governing their enzymatic activity reveal the intricacies of RNA processing and degradation, impacting gene regulation.
- Cellular Processes: RNases participate in essential functions such as RNA quality control and degradation, maintaining genomic integrity and cellular homeostasis.
- Clinical Implications: The therapeutic potential of RNases in treating diseases such as cancer demonstrates their importance beyond basic biology.
These points collectively reflect the vital role RNases play in maintaining life's molecular machinery. By grasping these crucial insights, students, researchers, and practitioners can better understand the broader implications of RNase functionality in molecular biology.
The Importance of RNases in Molecular Biology
The significance of RNases cannot be overstated; they are important agents in the regulation of RNA and genetic expression. Their roles extend into the realms of cell signaling and response to environmental stressors, thereby affecting cellular outcomes. Moreover, the abnormalities in RNase activity are linked to various diseases, underscoring their potential as biomarkers or therapeutic targets.
In the context of biotechnology, advances in the manipulation and application of RNases open avenues for novel treatments and diagnostic tools.
Thus, RNases exemplify a remarkable intersection of basic research and applied science, providing insights that can lead to meaningful advancements in health and industry.
"Understanding the complex role of RNases not only enriches our comprehension of molecular biology but also fuels innovation in biomedical research."
By synthesizing knowledge about RNases within this article, we highlight their indispensable presence in the scientific landscape, promoting further exploration and study in this field.