The Function of Hemoglobin: A Comprehensive Exploration
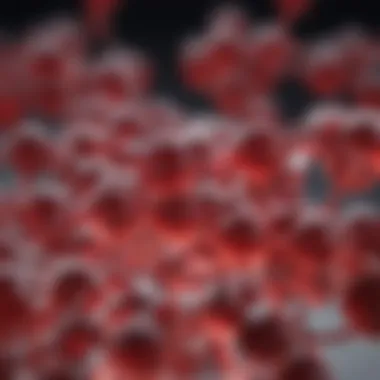
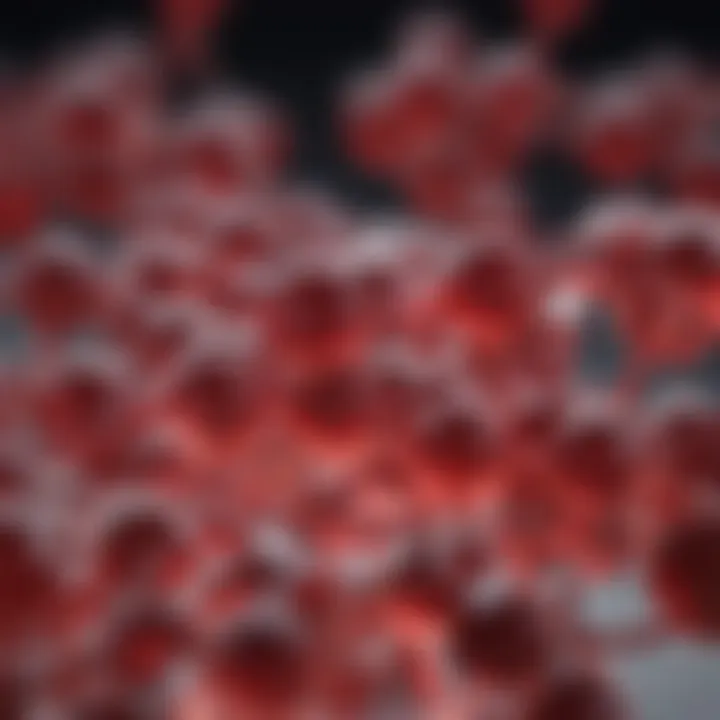
Intro
Hemoglobin is a protein that garners attention in both the biological sciences and clinical medicine due to its critical role in human physiology. It’s the primary component of red blood cells and serves as the highway for oxygen transport throughout the body. But there’s more than meets the eye when you delve deeper into the world of hemoglobin. There are layers of complexity in its structure and various functions that go beyond just ferrying oxygen.
In this exploration, we'll unpack how hemoglobin operates, differentiate among its varied forms, and discuss its variable interactions with both oxygen and carbon dioxide. By doing so, we aim to provide a holistic view that merges architectural biochemistry with real-world implications for health and disease.
Research Overview
The investigation into hemoglobin's multifaceted roles has spurred a plethora of studies. This section summarizes some of the most pressing findings and methodologies that have been employed to reveal the intricacies of this protein.
Summary of Key Findings
Recent research provides fascinating insights into hemoglobin's roles, such as:
- Oxygen Binding and Release: Hemoglobin exhibits cooperative binding behavior, meaning that the binding of one oxygen molecule increases the likelihood of more oxygen binding to other sites.
- CO2 Transport: It’s not solely about oxygen; hemoglobin also carries carbon dioxide from the tissues back to the lungs, facilitating respiratory function.
- Genetic Variability: Differences in hemoglobin structure, such as those found in sickle cell anemia, highlight its diversity across populations and species.
“Understanding hemoglobin isn't just about biology; it's about bridging that gap to clinical realities, such as managing hemoglobinopathies.”
Methodologies Employed
The studies have employed various techniques that illuminate the details of hemoglobin's function:
- Spectrophotometry: Used to assess how hemoglobin absorbs different wavelengths of light, aiding in understanding its oxygen-bound states.
- X-ray Crystallography: This method reveals the atomic structure of hemoglobin, explaining how its tertiary structure influences its function.
- Computational Modeling: Advanced simulations have been employed to predict behavior under various physiological conditions.
In-Depth Analysis
In examining the roles of hemoglobin, it becomes essential to analyze findings in detail and assess them against previous studies to unearth evolving insights.
Detailed Examination of Results
Understanding hemoglobin’s functional adaptability is crucial. Some studies have shown how variations can lead to altered oxygen affinity, which greatly affects athletic performance and endurance—key for athletes and individuals in high-altitude environments. Here, hemoglobin's ability to adapt its oxygen binding is influenced by factors like pH and carbon dioxide concentration, governed by the Bohr effect.
Comparison with Previous Studies
While previous findings established hemoglobin primarily as an oxygen carrier, newer research highlights additional layers, such as its role in nitric oxide signaling. This dual function illustrates how hemoglobin contributes not just to basic respiratory needs but also to vascular health. Earlier studies may not have fully encapsulated this aspect, focusing primarily on transport and affinity, thereby leaving a gap in understanding hemoglobin's broader physiological significance.
In summary, hemoglobin is a protein beacon guiding various vital processes in our bodies. Through this exploration, we aim to follow its journey—from molecular structure to clinical ramifications—bridging the theoretical and practical realms.
Prologue to Hemoglobin
Understanding hemoglobin is essential for grasping the intricacies of human physiology. Hemoglobin is not just a simple protein found in red blood cells but a dynamic player in our body's oxygen transport system. It interacts intricately with various gases, influencing both cellular functions and overall metabolic processes. The importance of hemoglobin extends into numerous clinical realms as well, allowing for the exploration of conditions such as anemia and other hemoglobinopathies. Thus, laying the groundwork in this section prepares the reader to appreciate how these elements all converge on the broader subject of oxygen transport and gas exchange.
Historical Perspective
The story of hemoglobin begins well back in the 19th century when scientists like Max von Pettenkofer and Richard Altmann significantly contributed to identifying its composition. However, it was not until the 1950s that the structure of hemoglobin was pieced together thanks to the pioneering work of researchers like John Kendrew and Max Perutz. Their efforts unveiled the three-dimensional structure of hemoglobin, allowing for a detailed understanding of how it functions at a molecular level. This historical context underscores the evolution of thought surrounding hemoglobin and highlights the strides made in biochemistry.
Basic Structure of Hemoglobin
To understand hemoglobin's function fully, one must delve into its structural components. The characteristic features of hemoglobin not only define its capabilities but also its interactions with various gases in the body.
Globin Chains
Globin chains are fundamental to hemoglobin, consisting of four polypeptide chains—two alpha and two beta chains. Each chain showcases a distinctive configuration that enables hemoglobin to perform its duties effectively. A key characteristic of these chains is their ability to change shapes during oxygen binding and release, known as conformational change. This feature allows hemoglobin to pick up oxygen in the lungs and release it in tissues where it is needed most. Importantly, variations in globin chains can lead to differing types of hemoglobin, such as fetal hemoglobin, which has a higher affinity for oxygen than adult hemoglobin, making it vital for fetal development. While generally advantageous, these variations can sometimes lead to disorders impacting oxygen transport.
Hem Group
At the heart of hemoglobin's functionality lies the heme group. Each of the four globin chains is paired with a heme molecule that contains an iron atom. This iron is crucial because it allows hemoglobin to bind to oxygen molecules. One might say that the heme group is the star player in the oxygen transport game, enabling hemoglobin to pick oxygen in the lungs and carry it throughout the body. Its unique feature is this ability to allow for reversible binding, so that release can occur smoothly at the required sites. However, the susceptibility of iron to oxidation poses concerns, as it can affect affinity for oxygen and lead to potential disorders—underscoring the balance needed in maintaining hemoglobin functionality.
Quaternary Structure
The quaternary structure of hemoglobin is another critical aspect, referring to the arrangement of the globin chains and heme groups into a functional unit. This four-subunit configuration is vital for cooperative binding—a method where the binding of one oxygen molecule enhances the likelihood of subsequent oxygen molecules binding to the remaining sites. This dynamic interplay offers hemoglobin its unique trait of adjusting its affinity based on the oxygen concentration in the blood. The major advantage of this feature is that it allows for efficient oxygen delivery, a key requirement for sustaining life. However, the complexity of this structure can sometimes be challenging, as alterations can lead to forms of hemoglobin that are not as effective, highlighting both the robustness and fragility of this protein.
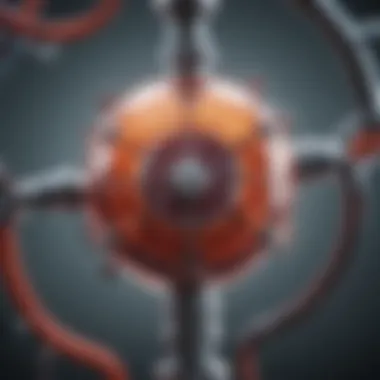
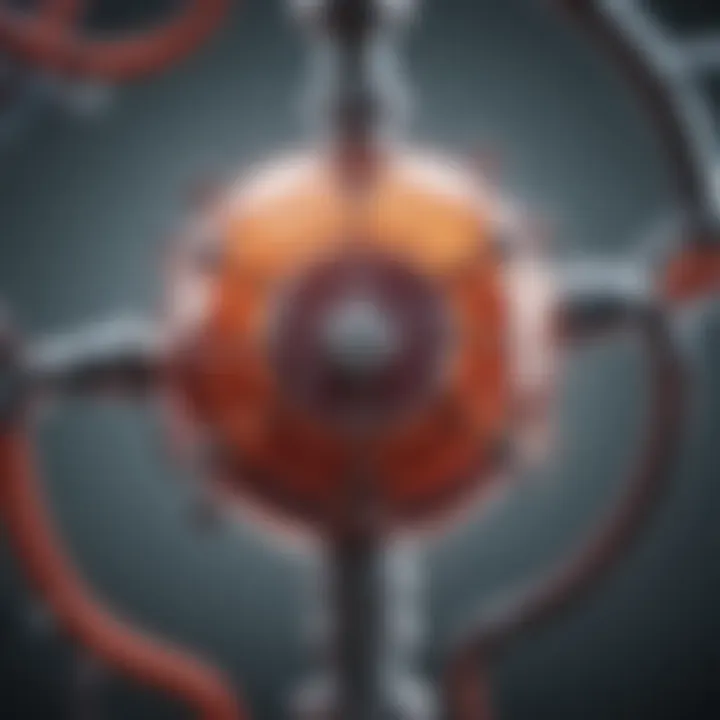
Primary Functions of Hemoglobin
The role of hemoglobin extends far beyond merely transporting oxygen; it is pivotal in several key physiological processes that underline its importance in human health and biology. Understanding the primary functions of hemoglobin provides insight into mechanisms of respiration and circulation—a cornerstone of human physiology. Its multifaceted nature allows hemoglobin to not only facilitate oxygen delivery but also to efficiently manage carbon dioxide levels in the blood, thus maintaining homeostasis. This section delves into two core areas: oxygen transport and carbon dioxide transport, both of which are essential for sustaining life.
Oxygen Transport
Oxygen transport is perhaps the most well-known function of hemoglobin. This molecule’s capacity to bind oxygen and transport it from the lungs to the tissues significantly shapes the respiratory system's efficiency.
Oxygen Binding Affinity
The oxygen binding affinity of hemoglobin is crucial in determining how effectively oxygen is absorbed and released. This characteristic refers to the degree to which hemoglobin can attract and hold onto oxygen molecules. A high binding affinity ensures that hemoglobin can pick up oxygen in the lungs where the concentration is elevated. This attribute is particularly beneficial in varying environmental conditions—like high altitude or during intense exercise—where oxygen levels fluctuate considerably.
One unique aspect of this process is the cooperative binding of oxygen. When one molecule of oxygen binds to hemoglobin, the structure of hemoglobin changes slightly, making it easier for subsequent oxygen molecules to bind. This notch in the chain is essential for maximizing oxygen uptake and is advantageous as it results in a significant increase in the total amount of oxygen transported with only a slight increase in pressure. However, it does come with disadvantages. For example, in certain pathological states like anemia, the binding affinity can be altered, leading to inefficient oxygen delivery to tissues.
Oxygen Release Mechanism
Equally important is the mechanism of oxygen release. Known as the allosteric effect, it allows hemoglobin to release oxygen where it's needed most—specifically in metabolizing tissues that produce carbon dioxide and create an acidic environment. As tissues utilize oxygen during cellular respiration, the corresponding rise in carbon dioxide concentration lowers the pH, prompting hemoglobin to release oxygen.
The key characteristic of this oxygen release mechanism is its efficiency and responsiveness to the metabolic needs of the body. This feature is advantageous because it ensures that when the demand for oxygen increases, so does its release. However, the flip side is that in instances of systemic acidosis, hemoglobin may release too much oxygen too quickly, which could aggravate local hypoxia in tissues.
Carbon Dioxide Transport
Moving from oxygen to carbon dioxide transport, hemoglobin demonstrates its versatility further. Not only does it carry oxygen effectively, but it also plays a pivotal role in removing carbon dioxide from the body, which is equally critical for maintaining a stable internal environment.
Carbon Dioxide as Bicarbonate
Carbon dioxide transportation primarily takes place in the form of bicarbonate ions. Once carbon dioxide diffuses from tissues into the blood, approximately 70% is converted to bicarbonate with the aid of the enzyme carbonic anhydrase. This conversion is essential for transporting CO2 efficiently back to the lungs for exhalation.
The unique feature of carbon dioxide as bicarbonate is its ability to regulate pH levels in the blood. Bicarbonate acts as a buffer, helping to neutralize acids produced from metabolic processes. This process is beneficial as it prevents harmful fluctuations in pH that could impair cellular function. Yet, it also comes with disadvantages: if bicarbonate levels are not adequately regulated, it may lead to respiratory acidosis or alkalosis, compromising the organism's homeostatic balance.
Role in Acid-Base Balance
The role of hemoglobin in acid-base balance is another critical facet of its functionality. As carbon dioxide is produced in tissues, its presence not only signals the need for oxygen but also affects the pH level of the blood. Hemoglobin's ability to bind to some of the hydrogen ions produced during CO2 conversion stabilizes the pH, illustrating a delicate balance that is crucial for physiological functions.
The key characteristic of this role is its robustness and responsiveness—it ensures that hemoglobin does not just ferry oxygen but also helps maintain the acid-base equilibrium in the body. However, as with any system, disadvantage exists; disruptions in this balance, often due to respiratory disorders, can lead to significant health issues, including confusion or cardiovascular distress.
Overall, the primary functions of hemoglobin encompass a complex interplay between oxygen and carbon dioxide management, ensuring efficient respiration while maintaining homeostasis in the body. Understanding these functions not only enhances our grasp of basic biology but is also crucial in clinical contexts, where disturbances in these processes can have profound implications for health.
Hemoglobin Variants Across Species
Hemoglobin, the protein responsible for transporting oxygen, exhibits remarkable variations across different species. Understanding these hemoglobin variants provides crucial insights into evolutionary biology, physiology, and clinical implications. This section will explore how diverse adaptations contribute to various environmental requirements, survival mechanisms, and how these variabilities translate into functional advantages.
Evolutionary Adaptations
Hemoglobin in Aquatic vs. Terrestrial Animals
The adaptation of hemoglobin in aquatic and terrestrial animals is a fascinating subject in evolutionary biology. Aquatic creatures, like fish, rely on hemoglobin that is efficiently tuned for extracting oxygen from water, which is typically less oxygen-rich than air. A significant aspect of fish hemoglobin is its higher affinity for oxygen in lower concentrations, allowing these animals to thrive underwater.
This higher affinity for oxygen is a beneficial trait as it permits aquatic animals to sustain themselves in environments where air-breathing would be untenable.
"Nature has its way of fashioning solutions that enable survival under varied circumstances."
For instance, the hemoglobin of some fish can undergo conformational changes to optimize function depending on environmental conditions.
In contrast, terrestrial animals have a different set of demands. They need hemoglobin that can operate effectively within a atmosphere with much higher oxygen concentrations. This leads to a slightly lower oxygen affinity compared to aquatic types, allowing for easier release of oxygen to tissues during physical activity.
One unique feature of terrestrial hemoglobin types is the ability to undergo extensive allosteric modulation, meaning that their oxygen-binding properties can adapt based on physiological demands. These adaptations showcase a prime example of how evolutionary pathways sculpt the hemoglobin molecule to meet specific environmental challenges.
High-Altitude Adaptations in Hemoglobin Structure
Another remarkable adaptation is seen in high-altitude species. Animals that reside in lofty areas, such as the Andean condor and Tibetan yak, possess specialized hemoglobins. One key characteristic of these high-altitude variants is their increased oxygen affinity, allowing the animals to effectively utilize the scarce oxygen available in their environments.
These adaptations might also involve alterations in hemoglobin structure, including modifications to the globin chains, enabling them to function optimally under low-oxygen situations. A notable feature of high-altitude hemoglobins is a greater cooperative binding effect, meaning that once oxygen begins to bind, the remaining sites become more receptive as well. This facilitates an effective oxygen uptake even in less-than-ideal scenarios.
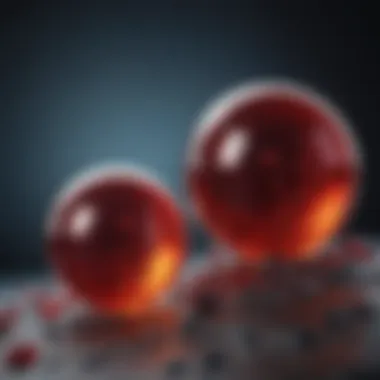
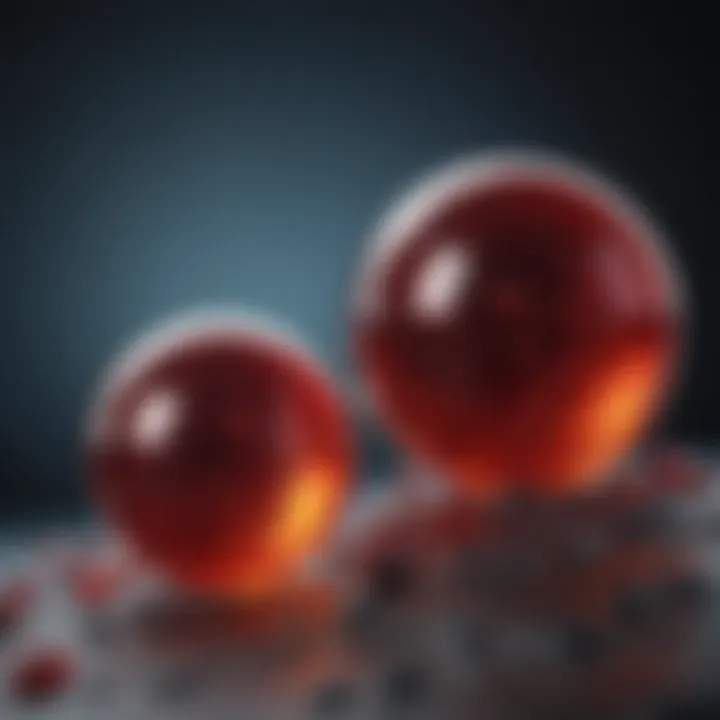
However, while these adaptations provide advantages for survival, they may also come with disadvantages. For instance, higher oxygen affinities can hinder the release of oxygen during intense activities, leading to potential physiological stress in some cases. When looking at the broader picture, these unique adaptations illustrate the intricate balance nature strikes to ensure survival across vastly different ecosystems.
Comparison of Hemoglobin Types
The comparison between adult and fetal hemoglobin is pivotal for understanding oxygen transport mechanisms. The adult form, hemoglobin A, typically possesses distinct properties compared to fetal hemoglobin F that allow the developing fetus to optimize oxygen from maternal circulation. The differences in structure facilitate a higher oxygen affinity in fetal hemoglobin, essential for survival in a low-oxygen environment typical of the womb.
Adult vs. Fetal Hemoglobin
One of the key characteristics that distinguish adult hemoglobin from fetal hemoglobin is the presence of different globin chains. Adult hemoglobin is composed of two alpha and two beta chains, while fetal hemoglobin contains two alpha and two gamma chains. This switch from gamma to beta chains at birth is crucial since fetal hemoglobin can more effectively capture oxygen from the mother’s bloodstream.
A unique feature of fetal hemoglobin is its reduced ability to bind to 2,3-Bisphosphoglycerate, a molecule present in red blood cells that generally decreases hemoglobin's oxygen affinity. This disparity allows fetuses to extract more oxygen from maternal blood. However, after birth, the transition to adult hemoglobin is necessary due to its adaptability to postnatal life, where oxygen levels normalize.
Variations in Avian Hemoglobin
Birds exhibit notable variations in hemoglobin types, which allows them to meet the demands of flight and high metabolic rates. A distinguished aspect of avian hemoglobin is its unique structure that supports a higher oxygen-carrying capacity, a pivotal feature for creatures that require explosive bursts of energy.
One salient point about avian hemoglobin is the presence of adaptations that enhance oxygen release in muscular tissues during flight. Like the high-altitude adaptations seen in certain mammals, avian hemoglobin can also switch between different forms, ensuring optimal function regardless of the circumstances. The structural differences contribute to the efficiency of oxygen delivery, making birds adept at soaring through various environments, from tropical forests to arid deserts.
These hemoglobin variations not only reveal evolutionary paths but also underscore the significance of physiological adaptations in diverse environments, emphasizing hemoglobin's role beyond mere oxygen transport.
Physiological Regulation of Hemoglobin
The physiological regulation of hemoglobin is fundamental in ensuring that oxygen delivery is optimized in response to the body’s varying needs. Hemoglobin’s efficiency is not a static quality; rather, it is adeptly modulated by a number of factors at both molecular and systemic levels. This adaptability is paramount because it allows the body to maintain homeostasis in the face of fluctuating oxygen requirements, such as during physical activity or in varying environmental conditions.
Allosteric Effects
Role of pH and CO2 Concentration
The role of pH and carbon dioxide (CO2) concentration is crucial in shaping hemoglobin's behavior in the bloodstream. This phenomenon is known as the Bohr effect, where an increase in CO2 or a decrease in pH results in hemoglobin releasing bound oxygen more readily. Mechanistically, as CO2 levels rise, it generates carbonic acid, leading to a drop in pH. Hemoglobin’s structure becomes altered, thus favoring oxygen release in tissues that are metabolically active.
This has a key characteristic: it ensures that oxygen is delivered precisely where it is most required, usually in tissues undergoing high metabolic demand. Such a property makes it a beneficial aspect to study regarding hemoglobin because it highlights not only its carrying capacity but also its responsiveness to systemic changes. The unique feature lies in the balance it achieves between oxygen transport and release, with notable advantages in maintaining effective perfusion to tissues under duress.
On the downside, in pathological conditions where pH is significantly disrupted, such as in cases of severe acidosis, hemoglobin's performance can be compromised, affecting oxygen transport efficiency and overall cellular function.
Impact of ,3-Bisphosphoglycerate
The impact of 2,3-bisphosphoglycerate (2,3-BPG) on hemoglobin regulation is another critical layer of understanding. 2,3-BPG is a glycolytic metabolite that binds to hemoglobin, decreasing its oxygen affinity. This means that higher levels of 2,3-BPG lead to more oxygen being released into tissues, particularly when oxygen levels are low or during conditions such as anemia.
This is a key characteristic because it showcases how metabolic needs can influence hemoglobin’s functionality directly. It is also a significant point of interest in this article as it illustrates another layer of regulation that complements the pH and CO2 adjustments. The unique feature of 2,3-BPG is its ability to modulate oxygen delivery dynamically, reflecting changes in metabolic activity across different physiological scenarios.
However, elevated levels of 2,3-BPG, while beneficial for oxygen release, can also become detrimental if not balanced correctly. Too much could lead to a state where oxygen affinity is so low that not enough oxygen is transported to vital organs, which is a delicate balancing act within the bloodstream.
Hormonal Influence
Effect of Erythropoietin
Erythropoietin (EPO) is a hormone produced primarily by the kidneys and is critical in the regulation of red blood cell production. The effect of erythropoietin on hemoglobin function lies significantly in its ability to stimulate erythropoiesis. Higher levels of EPO lead to increased red blood cell mass, consequently elevating hemoglobin concentrations that are crucial for oxygen transport.
This characteristic is beneficial for those needing to enhance their endurance or improve oxygen delivery, notably athletes or patients recovering from specific medical conditions. The unique feature of EPO is its targeted approach to promoting hemoglobin synthesis, aligning well with the physiological demand for oxygen. However, an overproduction of EPO, sometimes seen in chronic hypoxia or certain cancers, can lead to elevated hemoglobin levels which can increase risks for complications like thrombosis.
Glucocorticoids and Hemoglobin Levels
Glucocorticoids are another set of hormones that influence hemoglobin levels, primarily through their effects on metabolic processes and stress response. These steroid hormones can modulate erythropoiesis as well, albeit in more complex ways than EPO. Glucocorticoids can increase hemoglobin synthesis indirectly by promoting erythroid progenitor cell proliferation in the bone marrow.
One notable characteristic is their ability to mediate stress responses, allowing for adjustments in hemoglobin levels during prolonged illness or stress. This consideration makes glucocorticoids a critical focus in understanding hemoglobin regulation within the context of various health conditions, especially those characterized by inflammation or immune response.
However, excessive glucocorticoid levels, especially in conditions like Cushing's syndrome, can lead to anomalies, such as elevated hemoglobin levels, which pose additional health risks.
It’s important to recognize the iterative interplay between these hormonal factors and hemoglobin, as this chain of influence underscores the body's nuanced physiological responses to internal and external stimuli.
In summary, the physiological regulation of hemoglobin via allosteric mechanisms and hormonal influences highlights its complexity and adaptability. Understanding these dynamics helps elucidate not only how the body optimizes oxygen transport but also the potential clinical implications of dysregulation in various disorders.
Clinical Implications of Hemoglobin Function
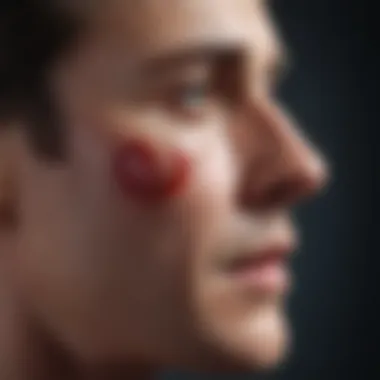
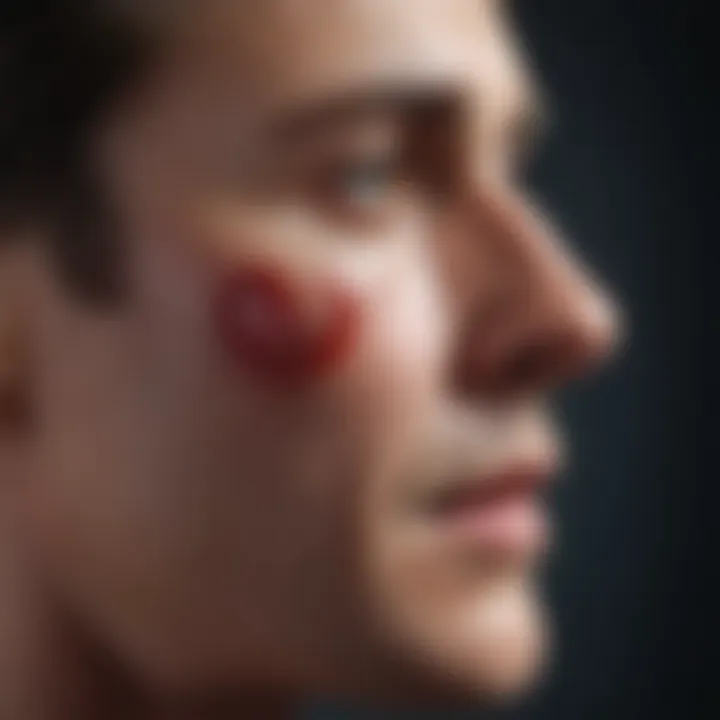
Hemoglobin, a crucial protein in red blood cells, doesn’t merely carry oxygen; its clinical implications stretch far and wide. Understanding hemoglobin's functions is key to diagnosing, managing, and treating various conditions, particularly hemoglobinopathies like sickle cell disease and thalassemia—two disorders that can have profound effects on individuals and healthcare systems. Additionally, monitoring hemoglobin levels is integral for assessing patient health across a multitude of scenarios, making this topic essential in both clinical and research settings.
Hemoglobinopathies
Sickle Cell Disease
Sickle cell disease, a genetic disorder caused by a single mutation in the beta-globin gene, serves as a prime example of how hemoglobin function can be altered. One of the most striking aspects of sickle cell disease is its impact on red blood cell shape. Under low oxygen conditions, these cells morph from their typical disc shape to a rigid, crescent-like structure. This transformation not only distorts the blood flow but also makes the cells prone to rupture, leading to chronic pain, organ damage, and increased risk of stroke.
Why is sickle cell disease a compelling choice for this article? Well, it illustrates the direct consequences of hemoglobin misfunction on human health. The unique feature here is the polymerization of deoxygenated hemoglobin, which drives the sickling process. This characteristic presents both advantages and disadvantages; while it sheds light on the importance of genetic factors in health outcomes, it also points to the complexity of managing such a multifaceted condition.
Thalassemia
Thalassemia, another hemoglobin disorder, arises from the underproduction of one of the globin chains. This leads to an imbalance in globin production, causing free heme that can be toxic to the body. There are two main types: alpha and beta thalassemia, each with distinct genetic origins and patient implications. Its key characteristic is the altered hemoglobin synthesis, disrupting normal physiological roles and presenting significant challenges for affected individuals.
Highlighting thalassemia in this article is important because of its varied clinical presentations, ranging from mild to severe anemia. A unique aspect of thalassemia is its treatment landscape, which may involve regular blood transfusions and iron chelation therapy, adding layers of complexity to patient management. This underscores the necessity of tailored healthcare approaches based on hemoglobin function.
Monitoring Hemoglobin Levels
Diagnostic Techniques
Monitoring hemoglobin levels is a cornerstone in clinical settings. Various diagnostic techniques, such as complete blood count (CBC), high-performance liquid chromatography (HPLC), and electrophoresis, are employed to determine hemoglobin concentration and identify different types. These methods are crucial for diagnosing conditions like anemia or polycythemia.
The key characteristic of these techniques is their ability to provide rapid and reliable results. Their importance is manifold: they inform treatment decisions, monitor disease progression, and evaluate response to therapies. The drawback, however, lies in accessibility. Not every healthcare facility possesses advanced equipment for all diagnostic methods, which can lead to disparities in care.
Clinical Indicators of Dysfunction
Clinical indicators of hemoglobin dysfunction are critical for patient management. One specific aspect includes symptoms like fatigue, pallor, or shortness of breath, which signal impaired oxygen delivery due to low hemoglobin levels. These indicators are vital, particularly in emergency settings where rapid assessment can dictate the course of treatment.
The highlight here is their ability to prompt immediate medical attention. Their unique feature is the constellation of symptoms that can reflect not just hemoglobin levels but also broader systemic issues, such as nutrition deficiencies or underlying chronic diseases. By recognizing these indicators, healthcare professionals can initiate timely interventions, improving overall patient outcomes. Their disadvantage, however, is that they can sometimes overlap with other conditions, complicating accurate diagnosis and requiring further investigation to pinpoint the underlying cause.
Emerging Research and Future Directions
The examination of emerging research areas regarding hemoglobin highlights its expanding role beyond merely transporting oxygen and carbon dioxide. These fresh directions of inquiry seek to unearth the intricate mechanisms by which hemoglobin participates in other physiological processes, potentially redefining its significance in health and disease.
Hemoglobin and Cellular Signaling
Role in Nitric Oxide Regulation
Nitric oxide (NO) has drawn considerable attention in recent years for its role in various biological systems, particularly in vascular health. Hemoglobin contributes to the regulation of nitric oxide within the circulatory system. It can bind and release NO, potentially acting as a reservoir that influences vascular tone. When hemoglobin binds oxygen, the process can lower its affinity for NO, allowing the gas to regulate blood flow effectively.
A key characteristic of this interaction is the dual functionality of hemoglobin—carrying oxygen while modulating NO levels. This characteristic is especially advantageous as maintaining an intricate balance between oxygen delivery and vascular relaxation is essential for optimizing tissue perfusion. Moreover, nitric oxide's ability to promote vasodilation demonstrates how hemoglobin may play a larger role in cardiovascular functions, far from its primary duties, suggesting a promising area for future studies.
Implications for Cardiovascular Health
The implications of hemoglobin's role in nitric oxide regulation extend to broader cardiovascular health concerns. For instance, dysregulation in NO availability could contribute to conditions like hypertension or atherosclerosis. Understanding the dynamics of hemoglobin in this context could lead to novel therapeutic approaches aimed at enhancing vascular health.
A notable unique feature of this research is the potential to manipulate hemoglobin's affinity for NO, thus modulating vascular responses as needed. This opens avenues for therapeutic interventions that could prevent or mitigate cardiovascular diseases associated with poor nitric oxide signaling. However, given the complexity of these interactions, further investigations are necessary to clarify the clinical applicability of findings pertaining to hemoglobin and NO.
Gene Therapy and Hemoglobin Disorders
The advancement of gene therapy technologies presents significant hope for treating hemoglobin disorders, such as sickle cell disease. Researchers are now exploring options to correct genetic mutations affecting hemoglobin proteins, with the aim of restoring normal function and alleviating symptoms. The ability to edit genes through CRISPR-Cas9 technology, for example, raises exciting possibilities for developing targeted treatments.
By focusing on the genetic underpinnings of hemoglobinopathies, this line of inquiry not only addresses the issues associated with these disorders but also allows for comprehensive insights into hemoglobin's functional repertoire. It fosters a more profound understanding of how specific mutations can alter hemoglobin’s efficiency, opening doors to customized medical strategies that are more effective than traditional methods.
In summary, the ongoing research into hemoglobin's multifaceted roles, especially in cellular signaling and gene therapy, marks a vital frontier in understanding human physiology and potential treatments for hemoglobin-related diseases.
Closure
Recapitulation of Key Points
- Structural Integrity: Hemoglobin’s unique structure, characterized by its globin chains and heme groups, is fundamental to its function. It allows for efficient oxygen binding and release, influencing how oxygen is distributed throughout the body.
- Transport Mechanisms: The dual function of hemoglobin in transporting both oxygen and carbon dioxide underlines its vital role. Through mechanisms like oxygen binding affinity and carbon dioxide conversion to bicarbonate, hemoglobin adapts to the body's metabolic demands.
- Clinical Relevance: Recognizing hemoglobinopathies such as sickle cell disease and thalassemia can be instrumental in clinical settings. The significance of monitoring hemoglobin levels furthers the understanding of various health conditions.
- Emerging Research: Current studies into hemoglobin's roles in cellular signaling and gene therapy present exciting potential avenues for innovative treatments and a deeper understanding of its functions beyond just gas transport.
The Future of Hemoglobin Research
Looking ahead, the field of hemoglobin research holds promising prospects. Investigators are keen on elucidating how hemoglobin might play a role in additional physiological and pathological processes, including its part in regulating vascular tone through nitric oxide. Potential breakthroughs in gene therapy could pave the way for treatments that rectify disorders directly affecting hemoglobin, hence improving patient outcomes.
Research focusing on the interaction between hemoglobin and various environmental factors, such as altitude and pollution, offers insights into evolutionary biology as well as future health implications for populations in changing climates. Moreover, advancements in diagnostic methods can provide sharper tools for monitoring hemoglobin function, leading to quicker and more effective clinical responses.
In sum, the future of hemoglobin research not just opens doors for innovations but also enhances our understanding of a fundamental component of human health, bridging biochemistry with applied medicine. This ongoing journey reflects the dynamism inherent in biological research, urging the next generation of scholars to delve deeper into this crucial molecule.