Understanding NGS: Principles, Applications, and Future
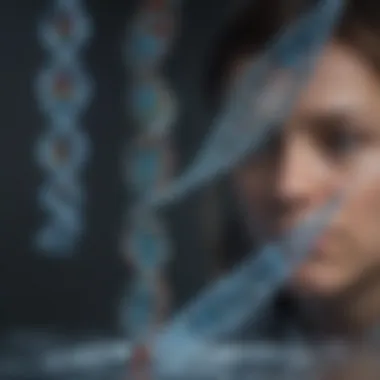
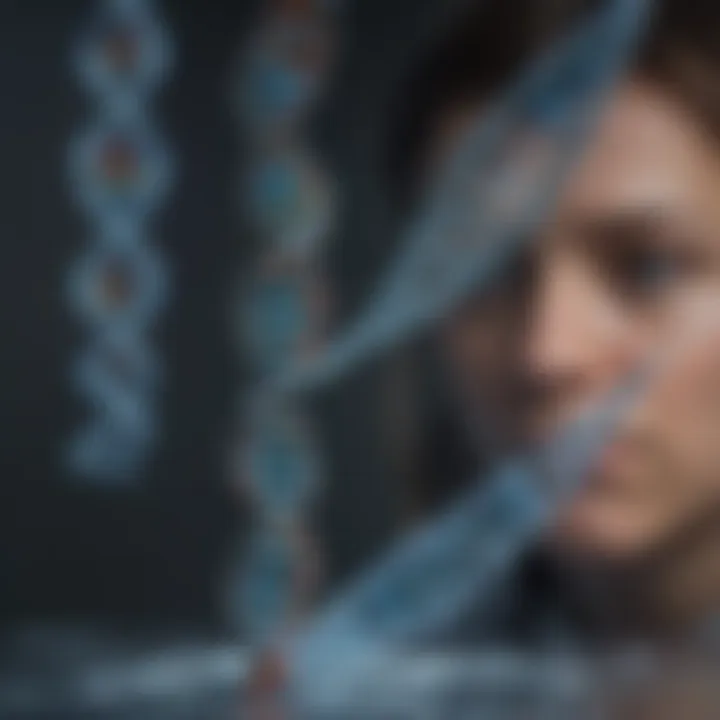
Intro
Next generation sequencing, abbreviated as NGS, has emerged as a crucial technology in genomics. It reshapes our understanding of biological systems by allowing fast and cost-effective sequencing of DNA and RNA. This section will set the groundwork for appreciating the significance of NGS in various fields, including medicine, agriculture, and environmental science. The intent is to outline the transformative nature of this technology, the driving principles behind it, and the broad spectrum of applications that it enables.
NGS is capable of generating vast amounts of genetic data, which can lead to breakthroughs in fields ranging from personalized medicine to crop improvement. It provides insights that were once out of reach, providing researchers unprecedented access to genomic data. This capability fosters significant advancements in understanding complex biological questions, enhancing diagnosis, and developing therapeutic solutions.
We will move forward to examine specific research findings that highlight NGS's contributions to current scientific endeavors.
Foreword to Next Generation Sequencing
Next Generation Sequencing (NGS) has emerged as a groundbreaking technology in the field of genomics, fundamentally altering how researchers approach the analysis of genetic material. As we delve into this article, it is essential to comprehend the significance of NGS. The technique allows for the rapid sequencing of DNA and RNA, leading to vast datasets that were once unimaginable. This efficiency not only accelerates scientific discovery but also promotes cost-effectiveness in genomic studies.
The key elements of NGS include its capacity to generate millions of sequences simultaneously, known as massively parallel sequencing. This is in stark contrast with traditional methods that could only sequence one DNA fragment at a time. The high throughput of NGS permits researchers to analyze complex genomes, discover variants, and understand genetic relationships more thoroughly.
Benefits of NGS extend beyond technical advantages. In the realm of clinical diagnostics, NGS plays a pivotal role in identifying genetic disorders and understanding cancer genomics. Similarly, it finds applications in agriculture, where it aids in crop improvement through genomic selection. Moreover, NGS has valuable implications in environmental monitoring, enabling the identification of microbial communities in neglected ecosystems.
However, its implementation presents several considerations. Ethical dilemmas pertain to data privacy, since NGS often involves the most personal information about individuals. There is a significant concern about genetic discrimination, raising questions about how genetic data should be managed. These factors must not be overlooked as society continues to harness the potential of NGS.
Understanding the intricacies of NGS is, therefore, crucial for students, researchers, educators, and professionals. Each of these elements intersects to create a comprehensive landscape of human knowledge and practices in genomics. As we proceed, this article will elucidate the principles, methodologies, applications, and broader implications of next-generation sequencing, providing a holistic view of its transformative power in the contemporary world.
In summary, Next Generation Sequencing represents a synthesis of innovation and practicality, reshaping our understanding of biology and medicine.
It is evident that grasping the fundamentals of NGS is not just an academic pursuit, but a necessity for contributing to the future of scientific inquiry.
Defining Next Generation Sequencing
Next Generation Sequencing (NGS) denotes a paradigm shift in genomic analysis. It encompasses a suite of technologies that allow for the rapid sequencing of DNA and RNA. In this context, understanding the nuances of NGS is critical. The importance of NGS lies in its capability to generate massive amounts of data in a fraction of the time and cost compared to traditional methodologies. This advance facilitates breakthroughs not only in research but in clinical environments as well.
Key elements of NGS:
- High-throughput capabilities: NGS can process multiple samples simultaneously, enhancing overall throughput.
- Cost-effectiveness: The overall cost of sequencing has drastically reduced, democratizing access to genomic information.
- Accuracy: NGS improves the fidelity of results compared to older approaches.
- Versatility: It can be used for various applications, ranging from whole genome sequencing to targeted resequencing and RNA sequencing.
Understanding these characteristics helps clarify the advantages of NGS. This insight allows researchers to select appropriate sequencing methods for their specific applications.
Characteristics of NGS
Next Generation Sequencing is defined by several hallmark features that distinguish it from traditional sequencing methods. First, the ability to sequence millions of fragments in parallel is a game-changer in speed and efficiency. Each run can yield gigabases of data, supporting comprehensive genomic studies.
Another essential characteristic is the various NGS platforms available. Illumina, Oxford Nanopore Technologies, and PacBio SMRT Sequencing provide options tailored to different needs, emphasizing flexibility in approach and technique. The adaptability in library preparation methods allows for customization according to project requirements.
Additional characteristics of NGS include:
- Dynamic data generation, allowing for real-time analysis.
- Scalability, supporting both small-scale experiments and large population studies.
- Integration with bioinformatics, providing detailed data interpretation and visualization tools.
These attributes make NGS indispensable for modern biological research and medical diagnostics.
Comparison with Traditional Sequencing Techniques
In contrast to NGS, traditional sequencing technologies, such as Sanger sequencing, are limited in throughput and more labor-intensive. Sanger sequencing provides data for only a single fragment at a time, which creates a bottleneck for larger projects.
Key differences between NGS and traditional sequencing include:
- Speed: NGS can deliver results in days, while Sanger sequencing may require weeks.
- Cost-Effectiveness: NGS reduces expenses significantly per base pair sequenced.
- Data Volume: NGS generates substantially larger datasets, suitable for complex analyses.
- Error Rates: While Sanger sequencing offers high accuracy for small-scale sequencing, NGS employs advanced error-correction techniques to manage accuracy across massive datasets.
In summary, the transformative capability of NGS not only enhances research efficiency but also expands the horizons of genomics as a field. Understanding these differences enables scientists and researchers to make informed choices about which sequencing methods best fit their specific needs, thereby driving innovation.
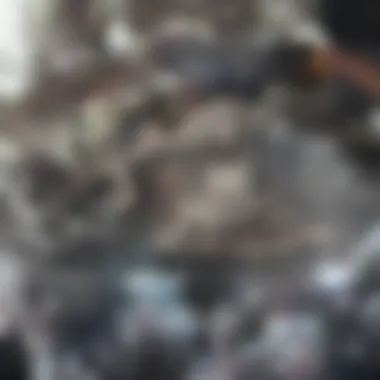
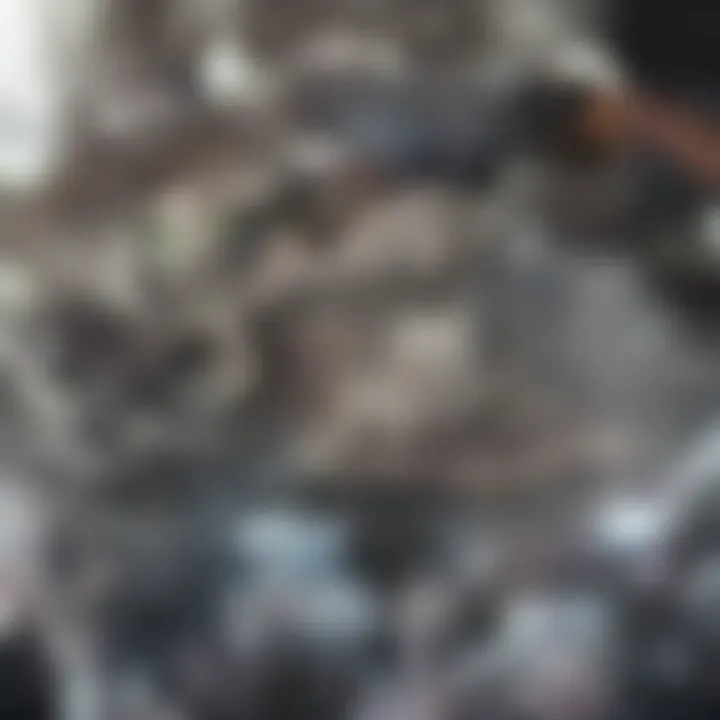
Principles of NGS Technology
The principles of Next Generation Sequencing (NGS) form the foundation of this transformative genomic technology. Understanding these principles is fundamental for grasping how NGS has revolutionized the field of genomics. NGS technologies enable the rapid and cost-effective sequencing of massive amounts of DNA and RNA, transforming not just research but also clinical practices.
One fundamental aspect of NGS is its high throughput capacity. This allows sequencing of millions of fragments simultaneously, which significantly accelerates the process compared to traditional methods. As a result, researchers can perform whole genome sequencing, targeted sequencing, or RNA sequencing efficiently. The benefits of NGS extend beyond speed. It generates vast amounts of data that facilitate comprehensive analysis, a point that is critical in fields like personalized medicine, where genetic information plays a pivotal role in treatment decisions.
Basic Mechanisms of Sequencing
The basic mechanisms of NGS encapsulate several techniques that enable the determination of nucleotide sequences. When a sample is prepared for sequencing, it undergoes processes like library preparation and sequencing itself. Library preparation involves fragmenting the DNA and adding specific adapters. The actual sequencing can utilize various technologies, each with unique methodologies, but they all aim to ascertain the order of nucleotides in the sample.
Library Preparation Techniques
Library preparation is an essential step that directly affects the quality and outcome of the sequencing. It consists of three main techniques: fragmentation, adapter ligation, and amplification.
Fragmentation
Fragmentation is a crucial technique in NGS library preparation. The DNA is broken into smaller pieces, which can then be individually sequenced. This process ensures that the data generated cover the entire genomic region of interest. A key characteristic of fragmentation is its ability to produce uniformly sized fragments. This is beneficial as it provides consistent data quality across different sequencing reads. Fragmentation can be achieved through various methods, including physical means, enzymatic methods, or chemical processes. While effective, one disadvantage is that over-fragmentation can lead to loss of important genetic information.
Adapter Ligation
Adapter ligation follows fragmentation. During this process, short pieces of DNA, known as adapters, are ligated to the ends of the fragmented DNA. These adapters are critical as they contain sequences necessary for the amplification and binding during sequencing. A key characteristic of adapter ligation is its specificity; the adapters are designed to fit only the fragmented DNA, ensuring accuracy. One unique feature of this technique is that it can include indices that allow for multiplexing, enabling multiple samples to be sequenced in a single run. The downside of adapter ligation can be the risk of low-efficiency ligations, which may lead to incomplete libraries.
Amplification
Amplification is the final step in library preparation. This process makes copies of the fragmented DNA with adapters, increasing the amount of DNA available for sequencing. Its key characteristic is the ability to generate sufficient quantities of DNA, which is often necessary for accurate sequencing. One unique advantage of amplification is that it can enhance detection of low abundance sequences, which is essential in applications like rare variant detection. However, one must exercise caution as over-amplification can introduce biases, affecting the final data quality.
Sequencing Platforms
Sequencing platforms offer distinct methodologies that play a vital role in how NGS data is generated. Several technological approaches are currently leading the market: Illumina, Oxford Nanopore Technologies, and PacBio SMRT sequencing, each with their unique advantages and considerations.
Illumina Technology
Illumina technology is perhaps the most widely used NGS method today. Its approach integrates sequencing by synthesis, where fluorescently labeled nucleotides are incorporated into strands of DNA. A key characteristic of Illumina technology is its high throughput capacity, enabling the sequencing of more than a billion sequences in a single run. The advantage of this platform lies in its accuracy and cost-effectiveness, making it a popular choice in both research and clinical laboratories. However, a limitation is its short read lengths, which may complicate assembly of complex genomes.
Oxford Nanopore Technologies
Oxford Nanopore Technologies employ a different technique, based on the measurement of electrical signals as DNA strands pass through nanopores. This method offers significant advantages, most notably the ability to generate long reads. A key characteristic is its portability, allowing for field-based sequencing. The unique feature is that it can simultaneously sequence and analyze the DNA in real-time. However, the disadvantage is the higher error rates compared to other methods, which may require additional validation steps.
PacBio SMRT Sequencing
PacBio SMRT (Single Molecule Real-Time) sequencing also stands out due to its long-read capabilities. This technology provides high accuracy in detecting longer stretches of DNA. The key characteristic is its ability to sequence complex regions of the genome more reliably. A unique advantage is that it measures the duration of each nucleotide incorporation event, which can yield insights into the structure of the DNA. However, the cost of PacBio sequencing can be greater than other platforms, which might limit its broader application in some contexts.
Steps Involved in NGS Workflows
The steps involved in NGS workflows are critical to the successful application of next generation sequencing technologies. Understanding these steps is crucial for researchers and professionals seeking to leverage NGS for various applications, ranging from clinical diagnostics to research innovations. Each segment of the workflow plays a unique role in ensuring that high-quality, reliable data is generated from the sequencing process.
Sample Preparation
Sample preparation serves as the foundation of the NGS workflow. In this phase, biological samples—such as blood, tissue, or plant material—are meticulously prepared for sequencing. The preparation includes the extraction of nucleic acids, which are often degraded or contaminated. Thus, the purity and integrity of the samples significantly impact the subsequent steps. Techniques like enzymatic digestion or mechanical disruption help in isolating DNA or RNA. The efficiency of sample preparation directly correlates to the quality of data produced, making it a vital consideration in any NGS project.
Data Generation
Data generation entails the process of transforming prepared samples into sequence reads. This is where the sequencing itself occurs, utilizing specific platforms such as Illumina or PacBio. Each platform uses distinct methods, relying on optical detection of nucleotide incorporation during synthesis. This phase is often the most resource-intensive and technologically demanding. It generates immense quantities of raw sequencing data, which must then go through initial processing for quality assessment. Understanding how data is generated helps in troubleshooting and optimizing protocols, which is crucial for successful sequencing outcomes.
Data Analysis and Interpretation
Data analysis and interpretation are essential for deriving meaningful insights from the generated sequence data. This step involves several sub-processes, each playing a significant role in ensuring the accuracy and relevance of the findings.
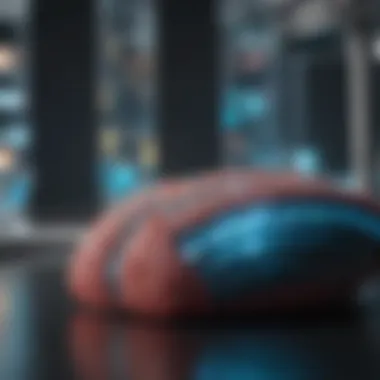
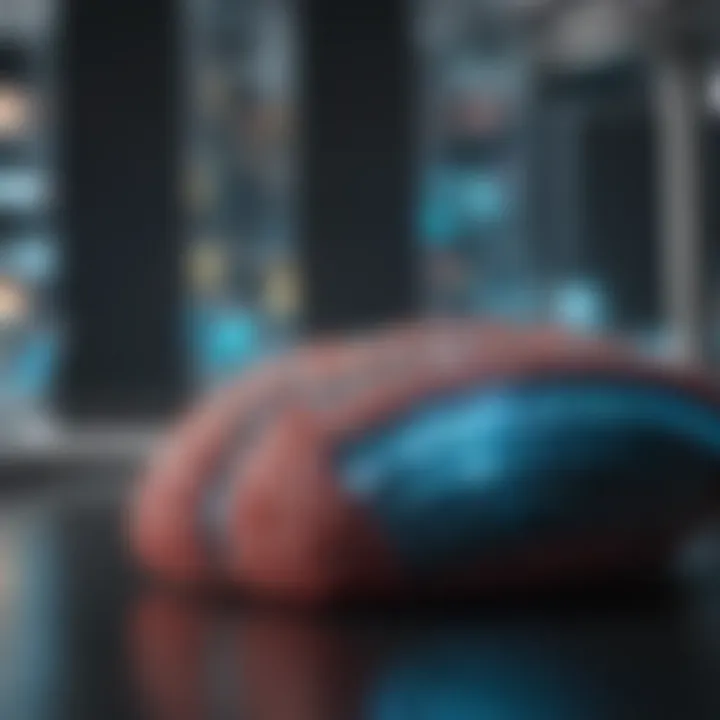
Quality Control
Quality control (QC) is crucial to assess the reliability of the raw data acquired from the sequencing runs. It involves evaluating the sequence quality scores, detecting adapter contamination, and identifying any biases caused during preparation. Tools such as FastQC are commonly used to generate reports on base quality distributions, GC content, and other key metrics. The significance of quality control lies in its ability to filter out low-quality reads that could lead to erroneous conclusions. The unique feature of effective quality control is its proactive nature; it ensures that only high-quality data is utilized for downstream analysis, enhancing the overall integrity of the research.
Alignment
Alignment involves mapping the obtained sequence reads to a reference genome. This process is pivotal for determining the exact location of sequences within the genome. Tools and algorithms such as Bowtie and BWA are typically employed to facilitate this step. Notably, alignment ensures that sequence variations are accurately identified. However, challenges may arise from repetitive regions of the genome which can complicate the process. Nevertheless, successful alignment is a hallmark of a well-structured NGS workflow and is vital for any genomic studies or variant analyses.
Variant Calling
Variant calling is the process of identifying variants, such as single nucleotide polymorphisms (SNPs) or insertions/deletions, present in the sequenced data compared to the reference genome. Tools like GATK or Samtools are often used to carry out this analysis. The importance of variant calling lies in its direct implications for understanding genetic diversity, disease associations, and evolutionary studies. It presents a unique feature in its potential to reveal alterations that occur within the genome, demonstrating how NGS can contribute to personalized medicine and other significant fields. Despite its advantages, variant calling can yield false positives, necessitating careful validation through additional experimental approaches.
In summary, the steps involved in NGS workflows—from sample preparation to data analysis—are interdependent. Each phase is crucial for generating reliable, high-quality genomic data necessary for various applications.
Applications of Next Generation Sequencing
Next Generation Sequencing (NGS) has a wide range of applications that significantly impact various fields, especially in clinical and research settings. The technology allows for comprehensive genomic analysis, providing insights into genetic variations. This capability not only enhances our understanding of diseases but also enables the development of personalized medicine. The versatility of NGS opens doors to numerous applications, each demonstrating unique characteristics and benefits.
Clinical Diagnostics
Genetic Disorders
Genetic disorders are among the most important areas where NGS is applied. NGS technologies facilitate the identification of mutations and genetic variations responsible for disorders. For patients with unexplained symptoms, genetic testing via NGS can lead to accurate diagnoses. This speed and accuracy make NGS a valuable tool in clinical settings.
A key characteristic of genetic disorders is their complexity. Many conditions can arise from multiple gene mutations, which can be challenging for traditional sequencing methods to detect. NGS addresses this complexity well, enabling healthcare professionals to explore a broad range of genes in a single test. Thus, it helps in identifying such disorders more effectively.
However, while the advantages of NGS in genetic disorder diagnosis are significant, challenges remain in interpretation of results and the need for skilled professionals to analyze the data.
Oncology
In oncology, NGS plays a crucial role in cancer diagnosis and treatment. Cancer is generally caused by genetic mutations, and identifying these mutations can help tailor treatments to individual patients. The technology assists in detecting actionable mutations that can guide targeted therapies, making it a critical tool in precision medicine.
The ability to assess tumor genomic profiles in detail highlights the importance of NGS in oncology. This specificity can lead to more effective treatment plans and better patient outcomes. However, NGS is not without its drawbacks. The costs involved and the need for high-quality tumor samples can be barriers to its widespread adoption in clinical practice.
Biomedical Research
Transcriptomics
Transcriptomics examines RNA transcripts within a cell, providing insights into gene expression levels. NGS has revolutionized this field by permitting the simultaneous analysis of thousands of genes. This capability allows researchers to explore the complexities of gene regulation and response to various stimuli.
The significance of transcriptomics cannot be understated. Understanding gene expression patterns is fundamental in various biological processes such as development, disease progression, and response to treatments. The unique feature of transcriptomics using NGS is its high-throughput capability, allowing for large-scale studies that were previously not feasible. However, challenges exist in analyzing the vast amounts of data generated, requiring advanced bioinformatics tools.
Metagenomics
Metagenomics focuses on studying genetic material recovered directly from environmental samples. NGS enables researchers to analyze the complex microbial communities present in diverse ecosystems without the need for culturing organisms. This aspect makes metagenomics particularly appealing. It opens avenues for understanding species diversity and function in various environments.
A key characteristic of metagenomics is its potential to uncover previously unknown organisms and their relationships within ecosystems. Moreover, understanding environmental microbes has implications in health, agriculture, and conservation efforts. On the downside, metagenomic studies can be data-intensive and require careful consideration of sample collection and processing methods.
Agricultural Genomics
Agricultural genomics utilizes NGS to enhance crop breeding and livestock management. By identifying beneficial traits at the genomic level, breeders can make informed decisions on which plants or animals to select for breeding. This leads to improved yield, disease resistance, and adaptability to climate change.
The integration of NGS in agriculture symbolizes a shift towards more precise and efficient breeding strategies. However, the accessibility of these technologies in developing regions remains a concern, hindering the full potential of agricultural genomics.
Environmental Monitoring and Conservation
NGS is pivotal in environmental monitoring and conservation efforts. It aids in understanding biodiversity and the impact of human activity on ecosystems. By generating genetic data from wildlife populations, NGS can reveal population structures, migration patterns, and adaptation to changing environments. This knowledge is critical for effective conservation strategies.

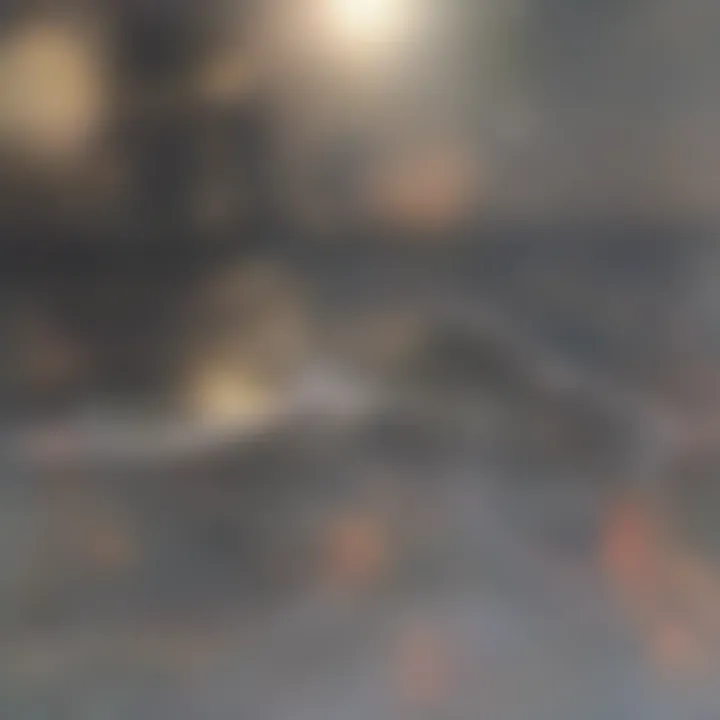
Overall, the applications of NGS are multifaceted and continue to evolve. Each field benefits from the unique capabilities that NGS offers, though challenges remain. As technology advances, it will be crucial to address these challenges to fully harness the potential of NGS.
Ethical and Social Implications of NGS
The rapid development of next generation sequencing (NGS) technologies has brought about significant advancements in various fields, including medicine and environmental science. However, these innovations also raise pressing ethical and social concerns that warrant serious consideration. Understanding these implications is essential for stakeholders, including researchers, clinicians, and policymakers, as they navigate the benefits and challenges presented by this powerful technology.
Privacy Concerns
As the accessibility of genetic information increases through NGS, privacy concerns become more pronounced. Genetic data can reveal not only an individual's predisposition to certain diseases but also information about their relatives. The potential for misuse of this information poses a significant risk.
Data breaches can expose sensitive genetic information, leading to unauthorized use. There is a need for stringent data protection regulations to prevent indiscriminate access to genetic data. Further, individuals often lack clear insights into where their data goes after sequencing. Thus, establishing robust frameworks to protect individual privacy remains a crucial challenge in NGS applications.
Genetic Discrimination
Genetic discrimination refers to the unfair treatment of individuals based on their genetic information. Such discrimination may occur in employment or insurance, where genetic predispositions can unjustly influence decisions.
For instance, if a genetic test shows an increased risk of a particular illness, an employer may be less inclined to hire that individual. Similarly, insurance companies could impose higher premiums based on genetic information. Legal protections against genetic discrimination, such as the Genetic Information Nondiscrimination Act (GINA) in the United States, are necessary, but they are not fully comprehensive or foolproof. The potential for discrimination highlights the importance of ethical guidelines and public policies that safeguard against bias associated with genetic data.
Informed Consent
Informed consent is another critical aspect of NGS that demands attention. Patients and research subjects should fully understand the extent and implications of genetic testing before undergoing such procedures. This concept extends beyond merely signing a consent form.
Individuals must be educated about the risks and benefits, how their samples will be used, and the possible outcomes of testing. Additionally, there must be clarity on how their data will be stored, shared, and protected. Failures in obtaining true informed consent can lead to ethical violations and mistrust in scientific research. Therefore, clear communication and a patient-centered approach are essential to achieving valid and ethical consent in NGS.
"As next generation sequencing continues to evolve, it becomes increasingly necessary to address the ethical and social implications that accompany its use. Without careful management of these concerns, the benefits of NGS may be overshadowed by unintended consequences."
Future Perspectives of Next Generation Sequencing
The field of genomics is rapidly evolving, and future perspectives of next generation sequencing (NGS) play a crucial role in shaping its trajectory. Understanding these perspectives provides insights into potential advancements that could enhance our capabilities in genetic analysis, diagnostics, and personalized medicine. As we consider the implications of NGS in various sectors, it is essential to focus on its significant technological advancements as well as the potential limitations that may arise.
Technological Advancements
Advancements in NGS technology continue to transform how researchers and clinicians approach genomic analysis. Some of the key areas of innovation include:
- Increased throughput: Next generation sequencing platforms are becoming increasingly efficient, capable of sequencing millions of fragments simultaneously. This means that research can generate vast amounts of genetic data quickly, facilitating faster discoveries in medicine and biology.
- Long-read sequencing: Companies like PacBio and Oxford Nanopore Technologies are pushing the boundaries of sequencing with long-read technologies, allowing greater accuracy in assembling genomes. This is particularly beneficial for complex genomes where short reads can lead to misalignment.
- Cost reduction: As technology matures, the cost of sequencing continues to decrease. This makes NGS more accessible to a broader range of institutions, enabling more widespread use for clinical and research applications.
- Integration of artificial intelligence: AI algorithms are improving data analysis by enabling quicker and more accurate interpretation of genomic data. This integration can enhance variant calling and lead to better clinical recommendations.
These advancements promise to make NGS an even more powerful tool in diverse fields, including healthcare, agriculture, and environmental sciences.
Potential Limitations
Despite the growth in NGS technology's capabilities, it is also vital to remain aware of its potential limitations. Some challenges that may impact the future of NGS include:
- Data management: The sheer volume of data generated by NGS necessitates robust data analysis and storage solutions. As data grows, so do challenges related to computational power and storage infrastructure.
- Error rates: Although advancements have reduced errors, some NGS platforms still face issues with accuracy. This can affect the interpretation of results, especially in clinical diagnostics.
- Ethical considerations: As NGS becomes integrated into more aspects of life, ethical concerns about privacy, genetic discrimination, and informed consent become more prominent. Clear guidelines and regulations will be necessary to navigate these complexities.
- Regulatory challenges: With the rapid pace of technological advancement, regulatory frameworks may struggle to keep up. This could lead to delays in the implementation of new technologies in clinical settings.
Overall, while the future of NGS is promising, it is essential to address these limitations to fully realize its potential.
As the field continues to evolve, balancing innovation with ethical and practical considerations will be crucial for the effective utilization of NGS in society. The insights drawn from understanding future perspectives can guide researchers and practitioners in making informed decisions that will shape the way genomic data is used in the years to come.
End
The conclusion serves as a vital synthesis of the insights gained throughout this article. It encapsulates the significance of next generation sequencing (NGS) in modern genomics, a discipline that has undergone remarkable transformation due to this technology. Understanding the principles and applications of NGS is crucial for students, researchers, and professionals alike.
By summarizing key elements such as the technical advancements, diverse applications, and ethical considerations, the conclusion reinforces the importance of NGS as a tool for innovation in various sectors. The benefits of NGS are manifold. For instance, it enables quicker and more accurate diagnostics, driving progress in clinical settings, while also contributing significantly to biomedical research.
However, the conclusion does not shy away from addressing potential concerns associated with NGS. Privacy and informed consent remain important discussions in the field. The advancements brought on by NGS come with responsibility. As we harness its power, we must also consider the ethical implications and societal impacts of genomic data.
As the future unfolds, NGS technology is expected to advance even further, creating new pathways in genomic research. The conclusion invites the audience to reflect on how these developments might influence their own fields of study or work.
In summary, embracing next generation sequencing is not just about understanding a technological innovation. It is about recognizing the profound implications this technology carries for our future in medicine, agriculture, environmental science, and beyond.
"Next generation sequencing is redefining the landscape of genomics, presenting both unparalleled opportunities and complex challenges."
It is essential for readers to engage with this dynamic field, ensuring they stay informed and prepared for the advancements that lie ahead.