Understanding Hydrogen Capture Technology Innovations
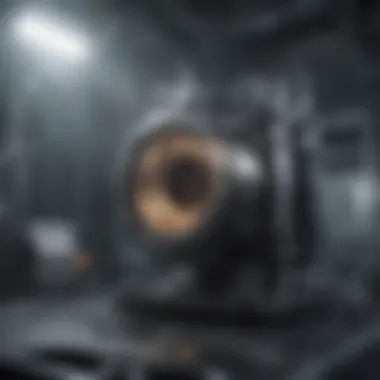
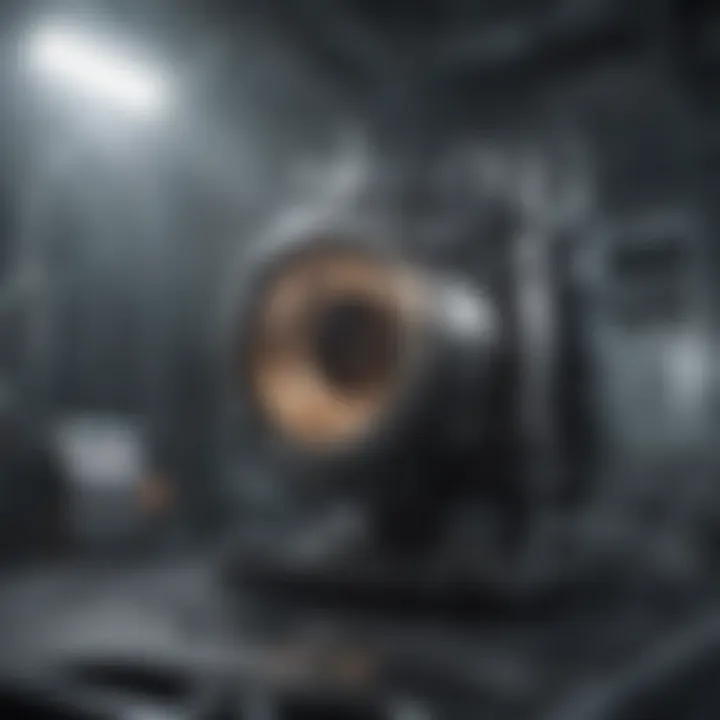
Intro
Hydrogen capture technology represents a pivotal shift in the energy landscape. As the world moves towards cleaner and more sustainable energy sources, capturing hydrogen emerges as a key solution. This technology is not just about capturing hydrogen from various processes, but it also focuses on how this element can be harnessed efficiently and responsibly. With the increasing urgency to address climate change, understanding the role of hydrogen capture is essential for stakeholders across sectors.
The article unfolds the complexities involved in capturing hydrogen. It highlights innovations that are pushing the boundaries of current technology while outlining the associated implications for environmental sustainability. The discussions shall provide a critical examination of the methodologies employed in the field, the challenges faced in deployment, and the potential for future developments.
Research Overview
The exploration of hydrogen capture is vital as it bridges gaps between traditional energy practices and future energy solutions. This section presents a summary of crucial findings within the realm of hydrogen capture technology.
Summary of Key Findings
Research indicates that hydrogen capture systems can significantly lower greenhouse gas emissions. Some key findings include:
- The efficiency of membrane technologies has increased markedly over the past few years, allowing for more effective separation of hydrogen from other gases.
- The development of adsorption materials, like metal-organic frameworks, has shown promising capabilities in capturing hydrogen at lower costs.
- Scalability remains a considerable challenge, but advancements are being made in adapting these technologies for industrial levels.
Methodologies Employed
In research surrounding hydrogen capture, several methodologies are employed to obtain viable data and insights. These often include:
- Experimental setups that involve pilot projects to test different technologies under real-world conditions.
- Computational modeling to predict the behavior of hydrogen capture systems and optimize designs.
- Life cycle assessments to evaluate the environmental impacts of hydrogen production and capture models.
In-Depth Analysis
This section seeks to provide a more detailed examination of hydrogen capture technologies and their implications.
Detailed Examination of Results
In recent studies, results indicate that employing innovative designs in hydrogen capture systems has yielded lower energy consumption during the process. Key results illustrate reductions in operational costs and improved energy efficiency. For example, the integration of renewable energy sources into hydrogen capture systems has been beneficial.
Comparison with Previous Studies
When comparing current advancements with past studies, noticeable differences surface. Older models often faced limitations in terms of efficiency and cost-effectiveness. Moreover, recent studies advocate for a synergistic approach, combining various hydrogen capture methods to maximize productivity and reduce waste.
βThe future of hydrogen is not just about capturing it but understanding how to utilize it effectively within our current frameworks.β
Epilogue
The article sets out to elucidate the vast potential of hydrogen capture technology in transforming our approach toward energy. By assessing current innovations and contemplating future trajectories, this exploration aims to equip stakeholders with the necessary understanding to navigate this developing field.
Intro to Hydrogen Capture Technology
Hydrogen capture technology is gaining significant traction as the world transitions to cleaner energy sources. It addresses the urgent need to manage hydrogen, a versatile energy carrier, by capturing and utilizing it effectively. This technology plays a crucial role in mitigating the adverse environmental impacts of fossil fuel usage while supporting the advancement towards a more sustainable and efficient energy system.
Definition and Overview
Hydrogen capture technology refers to the methods and processes used to isolate hydrogen from various sources, including industrial processes and renewable energy production. In essence, this technology allows for the collection and purification of hydrogen gas, making it available for diverse applications. Through techniques such as membrane separation, adsorption, and chemical absorption, hydrogen can be captured from emissions and other sources, reducing waste and promoting its use as a clean energy carrier. Understanding this technology involves appreciating the underlying principles and mechanisms, as well as the materials and systems used for effective capture.
Importance in Energy Transition
The importance of hydrogen capture technology in the energy transition cannot be overstated. As nations strive to meet ambitious climate goals, hydrogen emerges as a key player due to its potential to reduce carbon emissions significantly. Capture technologies enable the utilization of hydrogen from both renewable and non-renewable sources without exacerbating pollution.
Moreover, hydrogen can store and transport energy efficiently, acting as a bridge between renewable energy generation and consumption. It can be used in fuel cells for transportation, in industrial processes, or as a way to balance supply and demand in electrical grids. Thus, adopting hydrogen capture not only addresses emissions but also fosters energy security and economic resilience.
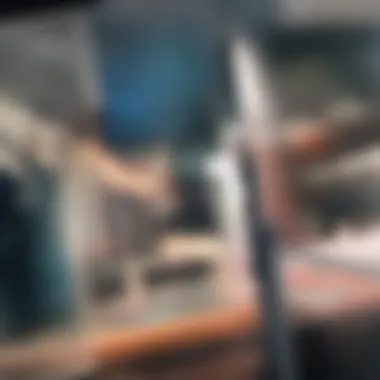
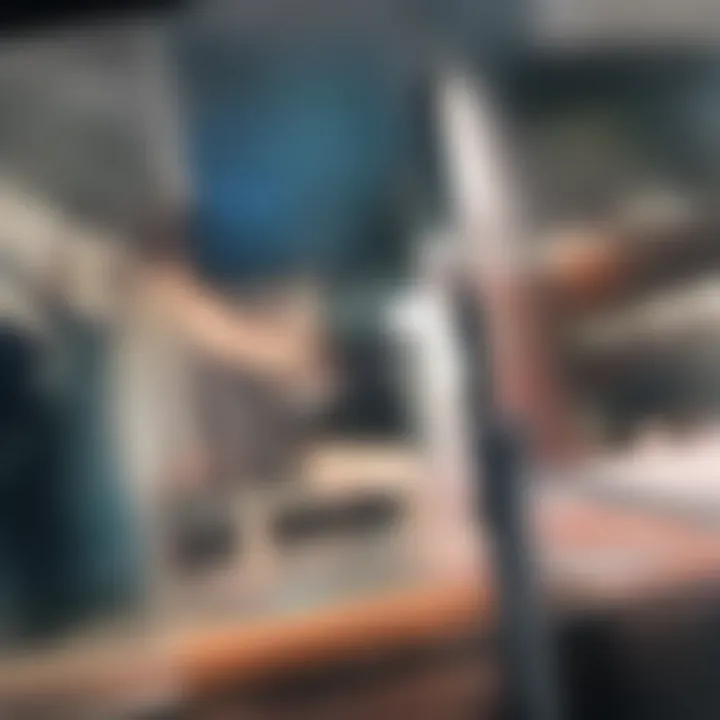
"Hydrogen capture technology represents a pivotal development in our quest for cleaner energy solutions."
Embracing this technology offers multiple benefits, including:
- Reduction of greenhouse gas emissions
- Increased utilization of renewable energy sources
- Enhanced energy storage solutions
- Economic opportunities in emerging hydrogen markets
Fundamental Principles of Hydrogen Capture
Understanding the fundamental principles of hydrogen capture is critical to grasping how this technology performs effectively. Detailed insights into these principles reveal the underlying mechanics necessary for optimizing the capture process. This section will address two primary principles: chemical thermodynamics and physical principles. Understanding these elements allows researchers and professionals to identify optimal conditions for hydrogen capture systems, facilitating better designs and more efficient operations.
Chemical Thermodynamics
Chemical thermodynamics plays a vital role in hydrogen capture processes. It focuses on the energy changes and the equilibrium relationships that govern reactions involving hydrogen. One important concept in thermodynamics is the Gibbs free energy, which indicates whether a reaction can occur spontaneously. In hydrogen capture, understanding these energy dynamics helps in predicting the feasibility and efficiency of processes, such as adsorption or absorption.
The enthalpy change is significant in determining the energy required for hydrogen to react. Specific capture methods may favor certain temperatures and pressures based on their enthalpic properties. For instance, higher pressures often enhance gas capture in adsorption methods due to decreased volume, favoring interactions between gas molecules and solid surfaces. On the other hand, some chemical absorption methods are temperature-dependent and require optimal conditions for maximum fluidic transformation.
Thermodynamic models can provide valuable insights for designing systems to capture hydrogen. By using these models, engineers can simulate operational conditions and test various scenarios before implementation, greatly minimizing risks and optimizing performance.
Physical Principles
Physical principles relate to the behaviors of matter and its interactions at different states. For hydrogen capture, the physical principles include diffusion, viscosity, and surface interactions. For example, diffusion directly influences how quickly hydrogen can move through membranes or into substances during capture processes.
Different materials used in membrane separation techniques exhibit varying diffusion rates, significantly influencing their effectiveness. In designing membranes, it is essential to choose materials that allow for rapid diffusion of hydrogen while blocking other gases. This selectivity is a critical requirement in many applications, ensuring high purity in the captured hydrogen.
Viscosity also plays a role in hydrogen capture through chemical absorption methods. Liquids with lower viscosity offer quicker mobility for hydrogen molecules to interact, enhancing the absorption efficiency.
Moreover, surface interactions dictate the efficacy of adsorption methods. The surface area and porosity of solid materials determine how much hydrogen can adhere to their surfaces. Enhanced surface characteristics can lead to greater interaction strengths, improving overall capture performance.
In summary, understanding chemical thermodynamics and physical principles is crucial for developing effective hydrogen capture technologies, leading to advancements in energy management and sustainability.
Technologies for Hydrogen Capture
Hydrogen capture technology is crucial in the context of emerging energy solutions. Innovative methods are being developed to ensure efficient and effective hydrogen separation from various sources. Understanding these technologies allows us to evaluate their potential impacts on the evolution of energy systems. This section will outline the primary techniques currently being utilized for hydrogen capture. Each technology has its own benefits, limitations, and applications that contribute to advancing our energy transition goals.
Membrane Separation Techniques
Membrane separation is a versatile method for capturing hydrogen. This technique involves selective permeation of hydrogen through a membrane while retaining other gases. Different types of membranes are available, including polymeric and ceramic membranes. Their effectiveness lies in their ability to provide high purity hydrogen with reduced energy consumption.
- Benefits: These membranes are usually lightweight and compact, making them suitable for various setups, including portable applications.
- Considerations: The durability and selectivity of membranes can vary significantly. Temperature and pressure conditions can also affect their performance and lifespan.
Adsorption Methods
Adsorption is a physical process in which hydrogen molecules adhere to the surface of a material, commonly known as an adsorbent. This method can capture hydrogen at lower pressures and is effective in separating it from gas mixtures. Many materials are being studied as adsorbents, such as zeolites and activated carbon.
- Benefits: Since this method does not require changes in phase, it tends to be cost-effective compared to other techniques.
- Considerations: The effectiveness of adsorption depends greatly on the choice of adsorbent material and operational conditions, such as temperature and pressure.
Chemical Absorption
Chemical absorption involves a chemical reaction between hydrogen and a liquid absorbent. This method can effectively capture hydrogen at high purity but is generally less efficient at low concentrations. Alkanolamines and other solvents are common absorbents used in this process.
- Benefits: The primary advantage is the capability to capture hydrogen at high concentrations with a high degree of purification.
- Considerations: This method often requires significant energy input during the regeneration phase, where the absorbed hydrogen is released.
Cryogenic Distillation
Cryogenic distillation is a more complex method that involves cooling gas mixtures until the hydrogen liquefies while other gases remain in the vapor phase. Itβs particularly useful for large-scale operations where high purity and recovery rates are required.
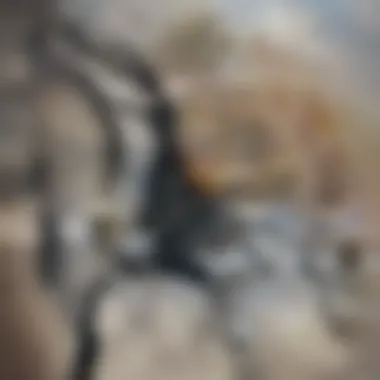
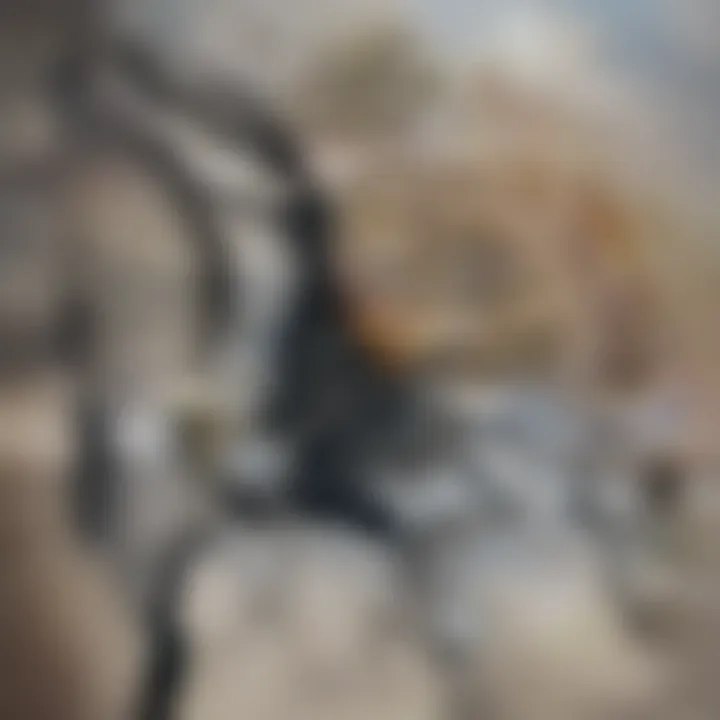
- Benefits: This technique can produce hydrogen with very high purity, suitable for demanding applications.
- Considerations: The energy-intensive nature of cryogenic processes can result in higher operational costs. Therefore, it is typically reserved for large-scale hydrogen production scenarios.
"Emerging hydrogen technologies exemplify innovative thinking in addressing energy challenges, offering practical solutions for cleaner energy production."
Current State of Hydrogen Capture Technology
The current state of hydrogen capture technology reflects significant advancements in processes and applications. The importance of understanding this state cannot be overstated, as it directly influences future energy solutions, sustainability efforts, and economic viability. As the world increasingly seeks alternative energy sources, hydrogen capture emerges as a pivotal component in facilitating the transition away from fossil fuels.
Global Market Landscape
The global market for hydrogen capture technology is evolving rapidly. Economies are focusing on reducing carbon emissions. According to recent reports, the hydrogen market is projected to expand significantly in the coming years.
Regions such as Europe, Asia-Pacific, and North America are leading this shift, supported by government policies and industrial interest. Investments are flowing into various components of hydrogen technology, including production, storage, and transportation. Key players include companies like Air Products and Chemicals, Inc. and Shell Hydrogen, which are innovating practical methods for large-scale hydrogen capture.
- The U.S. Department of Energy's funding has notably accelerated research.
- European companies are collaborating on projects for hydrogen production from renewable sources.
- In Asia-Pacific, there is significant interest in integrating hydrogen solutions into existing infrastructure.
These developments indicate a promising trajectory for hydrogen capture technologies, as solutions become more economically viable and scalable.
Leading Innovations
Innovation in hydrogen capture is crucial to addressing environmental demands and facilitating energy transitions. Some remarkable progress has been made in various areas of this technology.
- Membrane technologies: Advancements in membrane separation continue to enhance efficiency and selectivity in capturing hydrogen.
- Adsorption processes: The development of new adsorbent materials allows for greater capture rates even at lower pressures.
- Chemical absorption: New solvents and processes can capture hydrogen more effectively, thus contributing to lower operational costs.
- Cryogenic separation: Innovations here have improved energy efficiency in large-scale applications.
A significant aspect of these innovations is the interdisciplinary approach taken between researchers and industry. Collaborations between universities and technology companies are producing the next generation of solutions. Developing these technologies involves examination of not only technical viability but also economic considerations regarding implementation and operation.
"The advancements in hydrogen capture technology are critical for achieving a sustainable future, where energy systems are efficient and emissions are minimized."
Applications of Hydrogen Capture Technology
Hydrogen capture technology plays a vital role in various sectors, influencing energy production, environmental sustainability, and urban development. Understanding its applications is essential, as it underscores the technologyβs overall significance and the multifaceted benefits it offers. There are several key areas where hydrogen capture can have a profound impact, namely industrial utilization, integration in renewable energy systems, and potential applications in urban environments.
Industrial Utilization
In industrial settings, hydrogen serves as a critical feedstock for processes such as ammonia production and petroleum refining. The ability to capture hydrogen effectively can enhance efficiency and reduce greenhouse gas emissions significantly. For instance, the Haber process, essential in ammonia synthesis, generates large amounts of emissions. By capturing hydrogen, industries can minimize their environmental footprint while maintaining productivity.
Key considerations for industrial utilization include the cost-effectiveness of the capture process and conversion technology. As industries become more aware of environmental regulations, hydrogen capture technology offers a solution to comply with stricter guidelines. Moreover, companies investing in hydrogen capture can improve their public image, which can lead to increased consumer trust and corporate responsibility.
Integration in Renewable Energy Systems
The integration of hydrogen capture technology within renewable energy systems is another prominent application. Hydrogen production from renewable sources such as wind and solar energy can serve as an energy storage solution, balancing supply and demand. Capturing excess hydrogen produced during peak production times ensures energy stability and maximizes resource utilization.
The potential to harness hydrogen as a clean fuel source elevates the prospect of a sustainable energy future. Using captured hydrogen for fuel cells in transportation or electricity generation diminishes reliance on fossil fuels. Investments in research and development aim to enhance the efficiency of hydrogen production methods and the dynamics of integration across various energy systems.
Potential in Urban Environments
In urban settings, hydrogen capture technology shows promise not just for energy production but also as a strategy for improving air quality and reducing vehicular emissions. Hydrogen fuel cells can replace traditional internal combustion engines, leading to cleaner city environments. Capturing hydrogen from waste treatment processes or industrial activities within cities can foster a circular economy, where by-products are transformed into useful resources.
Urban planners and policymakers must consider the infrastructure needed for implementing hydrogen capture systems effectively. The interaction of hydrogen technology with existing urban ecosystems can enhance energy efficiency and promote sustainability initiatives. Leading cities are considering frameworks to integrate hydrogen into public transportation, thereby fostering sustainable urban mobility.
"The application of hydrogen capture technology in urban settings represents a transformative step toward cleaner, more sustainable cities. Beyond simply emission reductions, it offers innovative pathways to energy efficiency and resource management."
Overall, the applications of hydrogen capture technology extend across various sectors and environments, reinforcing its relevance in the ongoing transition toward sustainable energy solutions. The exploration and advancement in this field come with the promise of economic, environmental, and social benefits, enriching our understanding and fostering improvements in energy management.
Challenges in Hydrogen Capture
The development and implementation of hydrogen capture technology face several challenges that can impede its advancement. Understanding these challenges is essential for stakeholders in the science and technology sectors. It not only highlights the obstacles involved but also informs about the required innovations and strategies needed to navigate these complexities. Each identified challenge can potentially impact the efficacy and viability of hydrogen capture solutions, shaping the future direction of research and policy.
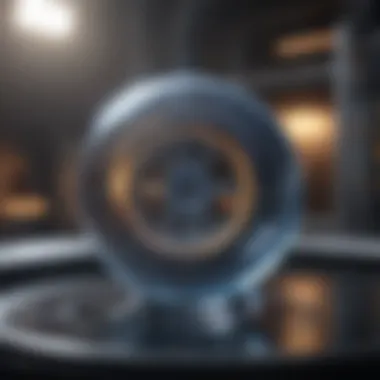
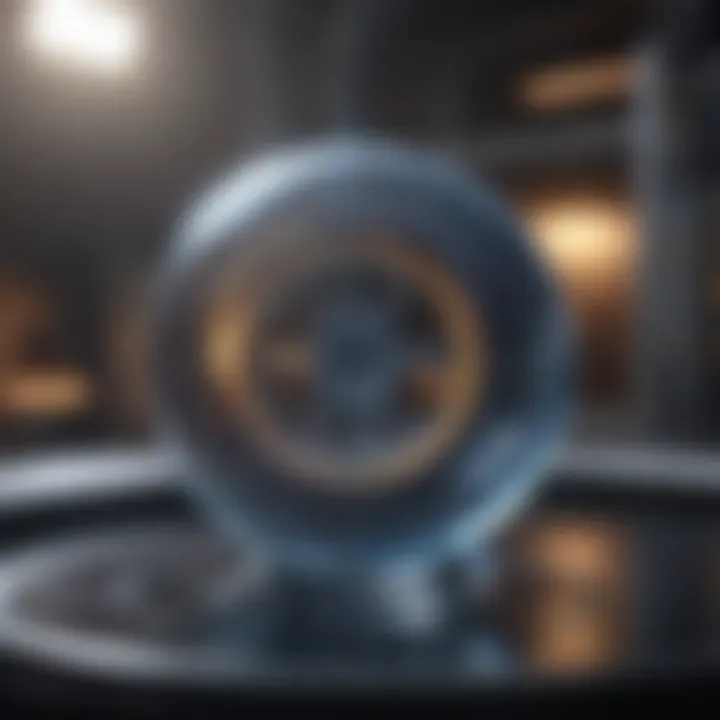
Economic Viability
The economic viability of hydrogen capture technology is a primary concern. Investment costs can be substantial, and operational expenditures may also add significant burden. The initial outlay necessary for developing hydrogen capture systems can discourage potential investors. Furthermore, market conditions and energy prices directly influence the economic landscape. If hydrogen production and capture are not economically attractive compared to traditional fossil fuels, adoption becomes limited.
- Cost of technology: High initial capital investment required for chemical absorption and membrane technologies.
- Competition with traditional energy sources: Fossil fuels remain cheaper in the short term, making it difficult for hydrogen solutions.
- Government policies and incentives: These play a crucial role; without supportive policies, it can be difficult for hydrogen initiatives to gain ground.
Technical Constraints
Technical constraints refer to the limitations in existing technology that hinder the effectiveness of hydrogen capture. These include operational challenges, efficiency issues, and the need for advancements in material science. Many technologies used for hydrogen capture exhibit difficulties that affect their long-term sustainability.
- Efficiency levels: Current technologies may not achieve the required efficiency in capture rates, making them less attractive for broad application.
- Durability and lifespan of materials: Many materials used in capture processes can degrade, impacting long-term functionality.
- Integration with existing systems: The ability to dovetail hydrogen capture technology with current industrial processes can be complex and costly.
"Technological advancement is crucial to mitigating the barriers faced in hydrogen capture. Future research should focus on enhancing efficiency and reducing costs."
Environmental Considerations
Finally, environmental considerations must be taken into account with hydrogen capture technology. Although the objective is to reduce greenhouse gas emissions, some hydrogen capture processes may have adverse environmental impacts.
- Energy consumption: Hydrogen capture processes can be energy-intensive, leading to greater resource use than expected.
- Waste management: The byproducts of certain hydrogen capture methods might pose disposal challenges that can affect local ecosystems.
- Lifecycle assessment: It is essential to evaluate the entire lifecycle, from production to end-use, to ensure that hydrogen technology remains beneficial for the environment.
Awareness and addressing these challenges will help define effective pathways forward in the hydrogen capture field. Continuous dialogue and research are needed to innovate solutions that respond to financial, technical, and environmental issues.
Future Directions in Hydrogen Capture Research
Research in hydrogen capture technology is at a pivotal juncture. As the world increasingly pivots toward sustainable energy solutions, exploring future directions in this field becomes vital. Innovations in this arena not only promise enhanced efficiency but also align closely with the global urgency for cleaner energy sources. This section will cover emerging technologies and interdisciplinary approaches that could significantly influence the landscape of hydrogen capture, emphasizing their potential benefits and the considerations they bring.
Emerging Technologies
Several emerging technologies are on the horizon, aiming to advance hydrogen capture mechanisms. These technologies promise to refine and elevate existing processes, making them more efficient and economically viable. Among the most notable innovations include:
- Solid-State Hydrogen Storage: This technology offers a compact and safer means of storing hydrogen. By utilizing advanced materials, it can maintain high energy density while minimizing leakage risks.
- Metal-Organic Frameworks (MOFs): MOFs represent a breakthrough in gas adsorption materials. Their high porosity allows for effective hydrogen capture under mild conditions, promoting operational cost reductions.
- Advanced Catalysts: Catalysts continue to play a crucial role in hydrogen capture. Exploring novel materials to enhance catalytic efficiency could lead to faster reaction rates and lower thermal energies.
- Bio-inspired Methods: Leveraging biological systems can yield innovative capture techniques. For instance, enzymes that naturally facilitate hydrogen production can inspire synthetic pathways for efficient capture.
Technological innovations in hydrogen capture can not only improve efficiency but also reduce costs. Enhanced capture efficiency translates into a more sustainable hydrogen economy, aligning with global goals for energy transition.
Interdisciplinary Approaches
Interdisciplinary approaches highlight the need for collaboration across multiple fields to fully realize the potential of hydrogen capture technology. Engaging professionals from chemistry, engineering, environmental science, and policy-making can yield transformative insights.
- Collaborative Research Initiatives: Partnering academic institutions with private industry can drive innovation. Collaborative research can address both technical and economic challenges, sharing resources and expertise.
- Policy and Regulatory Frameworks: Engaging policy-makers in research conversations can ensure that developments in hydrogen capture align with regulatory standards. Understanding policy implications can streamline the adoption process for new technologies.
- Public Awareness and Education: Increasing public understanding of hydrogen technologies can aid acceptance and encourage investment. Educational programs that outline hydrogen benefits can foster a more informed audience, supporting market growth.
- Sustainability and Environmental Impact Studies: Interdisciplinary research can provide comprehensive assessments of hydrogen capture technologies. Understanding their environmental impact is crucial to promoting their adoption.
Collectively, these interdisciplinary efforts foster an environment ripe for innovation. As various fields converge, the potential for breakthroughs in hydrogen capture expands.
"Interdisciplinary cooperation is key to unlocking the full potential of hydrogen capture technology."
The future of hydrogen capture technology is bright, driven by emerging innovations and strengthened by collaborative efforts across disciplines. Each stride forward in this field will contribute to a more sustainable energy future.
The End
In this article, the conclusion serves as a crucial integration of all previously discussed elements, honing in on the essence of hydrogen capture technology. This technology represents a key approach to addressing energy transition challenges, driving towards lower carbon emissions and fostering sustainability. Reinforcing the importance of this topic allows stakeholders to understand where current innovations stand and how they can be leveraged in various applications.
Summary of Key Points
- Hydrogen Capture Technology: A vital mechanism for capturing hydrogen generated from various sources, enhancing energy efficiency and minimizing waste.
- Technological Innovations: Significant advancements such as membrane separation and cryogenic distillation present unique benefits and challenges.
- Economic and Environmental Implications: The economic viability of hydrogen capture technologies must be critically evaluated alongside their environmental impact, ensuring a holistic approach to sustainability.
- Future Research Directions: There is a pressing need for ongoing research to address technical constraints and explore new, interdisciplinary methods that can elevate hydrogen capture techniques to new heights.
Call for Continued Research
The future of hydrogen capture technology relies heavily on sustained research efforts. As technology advances, the demand for more efficient, cost-effective solutions becomes evident. Key areas to focus on include:
- Interdisciplinary Collaboration: Engaging experts from various fields such as chemistry, engineering, and environmental science to foster innovative solutions.
- Investing in Emerging Technologies: Allocating resources to study and develop next-generation methods for hydrogen capture.
- Real-world Applications: Understanding how hydrogen capture can be integrated into existing infrastructures and energy systems to achieve maximum effectiveness.
Continued exploration in this domain is paramount. By inviting further research, we can refine hydrogen capture technologies, ensuring they meet future energy needs while considering economic and environmental sustainability.