Understanding the Genetic Code: Origins and Impact
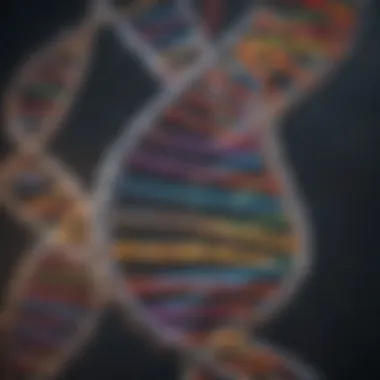
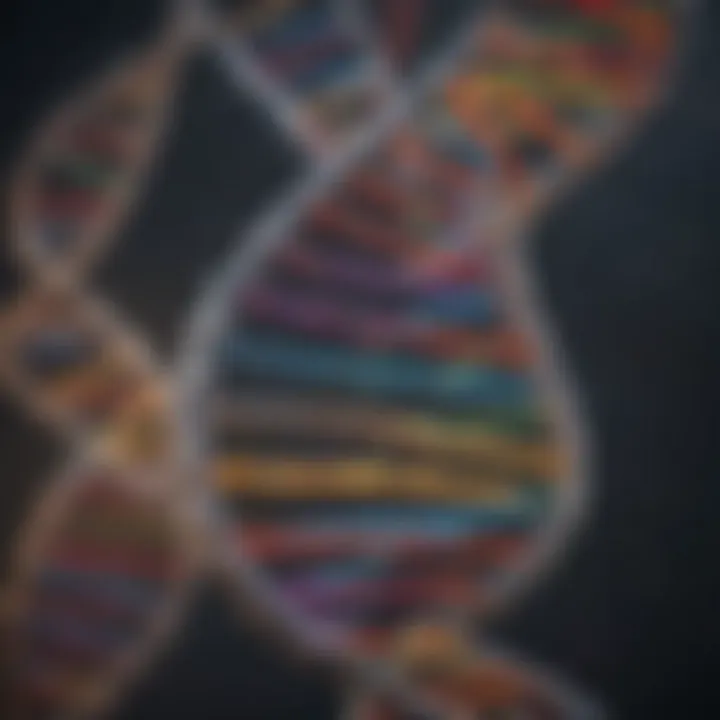
Intro
The genetic code stands as the cornerstone of biological science, a complex language that translates the sequences of nucleotides found in DNA into the proteins that form the very essence of life. These proteins are not just building blocks; they perform a myriad of functions necessary for the survival and reproduction of living organisms. Understanding this intricate system isnโt just an academic exercise; it's a pathway to unraveling the mysteries of evolution, tackling diseases, and advancing biotechnological innovations.
Familiarizing oneself with the genetic code involves peeling back layers of discovery from the historic early studies of DNA structure, to the ethical considerations of modern genetic engineering. This exploration reveals not just the mechanics of how life is encoded, but also illuminates the far-reaching implications of manipulating the genetic fabric through techniques like CRISPR technology. As we dive into this fascinating subject, we will examine key findings, methodologies, and provide a thoughtful analysis that stretches across different facets of the genetic code, providing context and clarity for students, educators, and professionals alike.
Preface to the Genetic Code
The genetic code stands as the backbone of biological sciences, serving as an essential framework for life itself. It dictates how proteins are synthesized, which are the building blocks of cellular structures and functions. Any comprehensive exploration into the realm of genetics must, therefore, begin with a solid understanding of this code. This section emphasizes the significance of delving into the genetic code, highlighting its underlying contributions to grasping complex biological processes and its implications across various disciplines.
By comprehending the genetic code, individuals can decipher much more than mere sequences of nucleotides. It opens the door to understanding evolutionary mechanisms, disease pathology, and even the burgeoning field of biotechnology. For students and professionals alike, recognizing the importance of this topic is crucial for navigating modern biological research and its real-world applications. The road ahead is rife with discoveries that could reshape not only science but also ethical norms surrounding genetic manipulation.
Definition of the Genetic Code
The genetic code is a set of rules that governs how the sequence of nucleotidesโadenine (A), thymine (T), cytosine (C), and guanine (G)โin DNA is translated into amino acids, the building blocks of proteins. In essence, it lays down a blueprint that every organism follows to create functional molecules necessary for life.
This code operates through codons, which are triplet sequences of nucleotides. Each codon corresponds to a specific amino acid or serves as a start or stop signal for protein synthesis. The universality of this code across nearly all organisms highlights its fundamental role in biology, something we will explore in detail in later sections.
Historical Context
Understanding the genetic code isnโt merely a modern endeavor; it is steeped in a rich history of discovery.
Early Discoveries
In the mid-20th century, scientists began to unravel the mysteries of heredity and genetic material. One of the pivotal moments was the elucidation of the structure of DNA by James Watson and Francis Crick in 1953. Their model provided a visual framework for how genetic information is stored and replicated, ultimately leading to the realization that DNA is not just a passive molecule but an active participant in cellular processes.
The key characteristic of these early discoveries lies in their transformative power. They laid the groundwork for molecular biology as a discipline. The collaborative efforts of many scientists during this period turned out to be highly beneficial for subsequent research on the genetic code. It marked a shift away from classical genetics into a molecular understanding of life.
However, those discoveries do come with a unique feature that deserves recognitionโthe rapid pace of research, which sometimes overshadowed ethical considerations, laying bare the need for a nuanced approach toward genetic manipulation.
Key Figures in Genetics
The world of genetics has been shaped by remarkable individuals who advanced our understanding of heredity and genetic function. Gregor Mendel, often regarded as the father of genetics, set the stage with his study of pea plants, uncovering the basic principles of inheritance even before DNA was known.
Following Mendel, figures like Barbara McClintock made significant contributions with her discovery of transposable elements, demonstrating that genetic material could move around within the genome. This insight was revolutionary, showcasing the complexity of genetic information beyond simple linear sequences.
These key figures emphasize the interconnectedness of genetics and the collaborative spirit of scientific inquiry. Their work illustrates that progress in understanding the genetic code does not belong to a single person but is built upon the shoulders of many.
Consequently, understanding who these contributors are offers substantial insights into how the field has evolved over time. Each breakthrough adds depth to our collective knowledge and highlights the importance of a multifaceted approach to genetics, making it a rich topic for exploration in this article.
"To understand the genetic code is to understand the very language in which life is written."
Structure of the Genetic Code
The structure of the genetic code is fundamental to understanding how life operates at a molecular level. It provides insight into the way biological information is stored, transferred, and translated into the functional proteins that sustain life. This section delves into two main components: the building blocks of DNA and the coding sequences that dictate protein synthesis. The examination of these elements is crucial for grasping the implications of genetic studies, ranging from biodiversity to biomedicine.
Components of DNA
Nucleotides
Nucleotides, the building blocks of DNA, are vital components that its structural integrity hinges upon. Each nucleotide consists of three parts: a phosphate group, a sugar molecule, and one of four nitrogenous basesโadenine, thymine, cytosine, or guanine. One key characteristic of nucleotides is their ability to form long chains through phosphodiester bonds, allowing for the creation of the double helix structure prominent in DNA. This characteristic makes nucleotides a highly beneficial choice in the context of this article as they not only support genetic coding but also facilitate replication and repair of DNA.
A unique feature of nucleotides is their capacity for base pairing, where adenine pairs with thymine, and cytosine pairs with guanine. This pairing enables the preservation of genetic information across generations. However, a disadvantage might arise when mutations occur, leading to potential malfunctions or diseases, complicating our understanding of genetics. Their role in the genetic code can't be overstated; nucleotides collectively determine an organism's traits and characteristics.
Base Pairing
Base pairing is an essential aspect of the genetic code, playing a critical role in maintaining the accuracy of genetic information. The complementary nature of the base pairing ensures that during DNA replication, the precise genetic sequences are copied. This key characteristic underscores the importance of base pairing in genetic stability, making it a popular choice for explorative studies discussed in this article. The fidelity of replication is essential for the propagation of life.
A noteworthy aspect of base pairing is the formation of hydrogen bonds between complementary bases, which adds a level of specificity and reliability to genetic information transfer. While there are advantages, such as creating a stable structure for the double helix, disadvantages may include the possibility of mismatches during replication, which could lead to genetic disorders. Understanding base pairing provides a foundational layer in unraveling the complexities of the genetic code and its implications for life.
Codons Explained
Triplet Code
The triplet code is a crucial feature of how the genetic code conveys information necessary for protein synthesis. Each triplet (or codon) consists of three nucleotides, which correspond to a specific amino acid or signal within the protein synthesis process. A primary advantage of this triplet system is its ability to encode 64 possible combinations, thereby allowing for a diverse array of proteins to be made, showcasing the richness of genetic information.
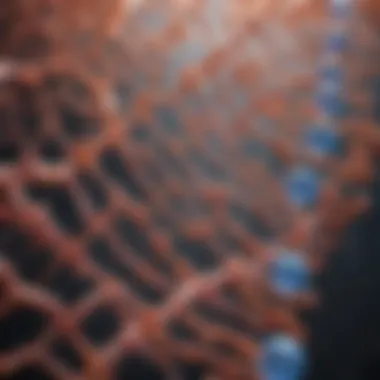
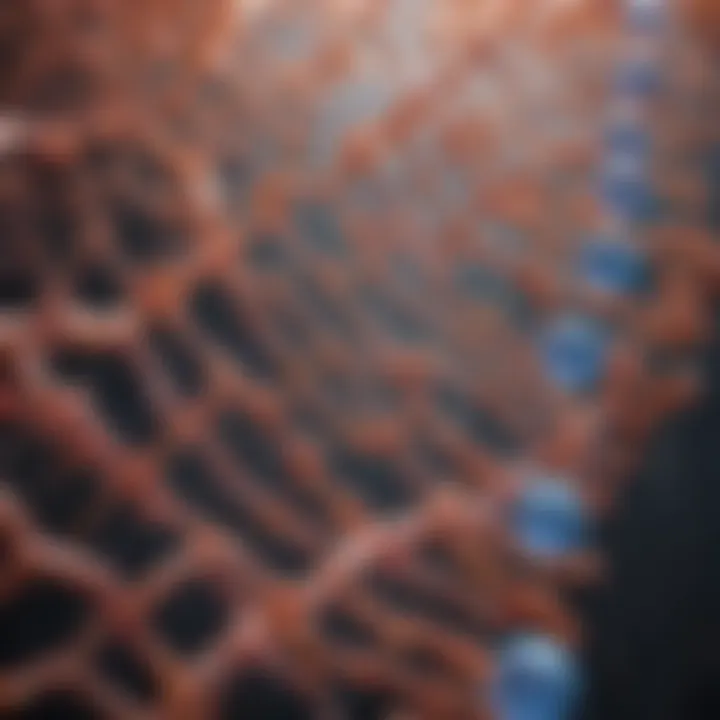
One unique quality of the triplet code is its redundancy; for example, multiple codons can code for the same amino acid. This redundancy acts as a safety net against mutations, ensuring that a certain degree of genetic integrity is maintained. Nonetheless, this complexity can present challenges for researchers attempting to decode genetic sequences efficiently.
Start and Stop Codons
Start and stop codons are pivotal in making sense of the genetic code during translation. The start codonโusually AUGโsignals the initiation of protein synthesis, while stop codons indicate termination. This system is paramount for ensuring that proteins are synthesized accurately and efficiently. The presence of these codons is a beneficial aspect because, without them, the process of translation could yield proteins that are either incomplete or non-functional.
A distinct advantage of start and stop codons is their consistency across species, providing a universal language for the translation process. However, a disadvantage is the susceptibility to translation errors which could lead to malformed proteins, subsequently impacting cellular function. Recognizing these codons helps illuminate the regulatory mechanisms that govern gene expression, tying back into the broader theme of genetic implications discussed in this article.
Structurally, the genetic code consists of elements that work together harmoniously to ensure life functions as intended. Understanding these foundations is key to unlocking the complexities of genetics.
Function of the Genetic Code
The genetic code plays a fundamental role in living organisms by acting as the blueprint that governs protein synthesis and, by extension, cellular activities. Understanding this intricate framework is crucial because it sheds light on how genes direct the formation of proteins, which are essential for various biological functions and processes. From cell growth and repair to defense against diseases, the function of the genetic code is paramount.
The significance of the genetic code is underscored by several key elements:
- Protein Synthesis: Every cell relies on the genetic code to produce proteins, which are vital for sustaining life. This process of protein creation involves both transcription and translation, each contributing unique characteristics that enhance our understanding of biology.
- Gene Regulation: A pivotal aspect of genetic function involves the ability of cells to regulate gene expression based on environmental cues or developmental signals. This regulation can have profound implications for how organisms adapt to their surroundings.
- Cellular Responses: The genetic code influences how organisms react to external stimuli, ensuring that cells can communicate and respond effectively.
Protein Synthesis Process
Transcription
Transcription marks the initial step in the protein synthesis pathway, where a segment of DNA is copied into messenger RNA (mRNA). This process is essential, as it transforms the genetic information housed in DNA into a format that can be manipulated to synthesize proteins. One key characteristic of transcription is its specificity; only the necessary genes are transcribed at any given time, reflecting the cellโs requirements. This adaptability makes transcription an integral part of cellular function.
One unique feature of transcription is that it allows for alternative splicing, where different arrangements of exons can result in multiple protein variants being produced from a single gene. It serves as a double-edged sword; while it provides flexibility and diversity, it also adds layers of complexity that can complicate the mechanism of gene expression management.
Translation
Following transcription, translation occurs in the ribosomes, where the mRNA is decoded to synthesize proteins. This phase is foundational in converting the genetic code into functional molecules. Translation is characterized by its efficiency; several ribosomes can translate a single mRNA strand simultaneously, thereby speeding up protein production.
An interesting aspect of translation is the role of transfer RNA (tRNA), which delivers the appropriate amino acids to the birthing protein chain in the correct sequence. This precision ensures that proteins are built accurately to fulfill their designated roles in cellular processes. However, the complexity of translation can also lead to errors, highlighting the delicate balance that organisms maintain in this vital step of genetic expression.
Role in Cellular Functions
Gene Regulation
Gene regulation operates as the control system for how and when genes are expressed. This regulation is critical for maintaining homeostasis and allows organisms to adapt to changing environments. A pivotal characteristic of gene regulation is its layered complexity; multiple signals can converge to influence gene expression, making it a fascinating area of study.
One distinctive element of gene regulation is the presence of enhancers and silencersโregulatory sequences that can enhance or inhibit transcription from a distance. The advantages of such dynamic regulation allow for survival in various conditions, but the drawback lies in its potential to malfunction, leading to diseases such as cancer.
Cellular Response to Environments
Cells constantly interact with their environment, and their genetic code informs how they respond. This responsiveness is critical for survival and adaptation. The core aspect of this function involves environmental sensing, which can trigger cellular pathways designed to initiate appropriate responses, such as stress management or immune reactions.
A unique feature of how cells respond to their environment is epigenetic modifications, where external factors can influence gene expression without altering the underlying DNA sequence. While this responsiveness grants cells a remarkable level of adaptability, it can lead to unintended consequencesโlike the potential for maladaptive responses that might affect long-term survival.
"Genetic regulation isnโt just about genes; itโs how those genes interact with the environment. Much like a well-oiled machine, every piece must be in sync for the system to function smoothly."
All these components highlight the fundamental role the genetic code plays in shaping not just individual organisms but also the complexity of life itself. With every twist and turn in this narrative, we begin to appreciate the elegance and intricacy embedded within the foundation of biology.
Evolution of the Genetic Code
Understanding the evolution of the genetic code unveils a fascinating interplay between biology and environmental conditions. At its core, the genetic code is not static; it has transformed over eons, gradually shaping the diversity of life we see today. Analyzing its evolution provides critical insights into adaptation mechanisms, speciation events, and even the overall health of ecosystems. This section will delve into two primary influences on the genetic code's evolution: abiotic factors and biological evolution.
Origins of the Genetic Code
Abiotic Factors
Abiotic factors refer to the non-living components of the environment that can influence biological processes. These determinants, such as temperature, pH, and environmental pressure, played a crucial role in the origins and evolution of the genetic code. Notably, how these factors shaped molecular interactions can seriously affect genetic variations among organisms.
One key characteristic of abiotic factors is their universality; they oscillate with time and influence entire ecosystems. In this article, highlighting them as significant contributors provides a robust framework for understanding how basic chemical principles can lead to complex biological systems.
A unique feature of studying these factors is appreciating how they create selective pressures. For example, extreme temperatures can lead to the emergence of heat-resistant proteins encoded by specific codons. Understanding abiotic influences on the genetic code thus not only enhances knowledge of molecular biology but also informs research in evolutionary genetics and ecology. However, one disadvantage is that the link between abiotic conditions and genetic outcomes often remains obscured, necessitating complex modeling to see clear connections.
Biological Evolution
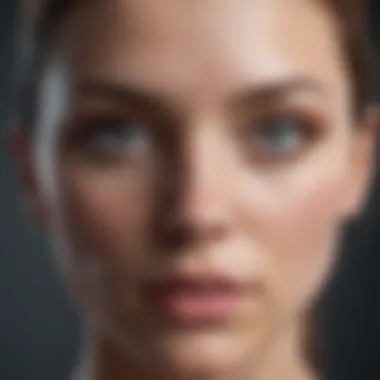
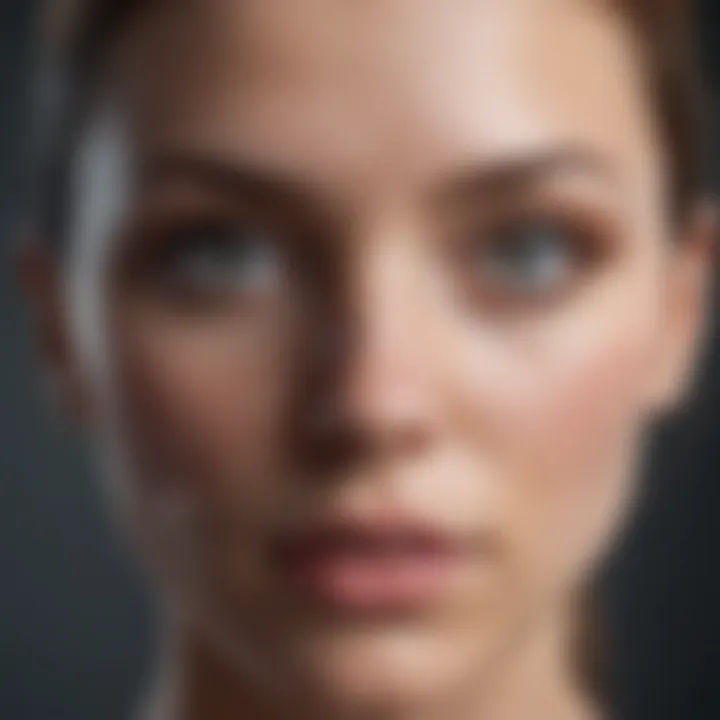
Biological evolution encapsulates the change in the inherited characteristics of biological populations over successive generations. Itโs the bedrock of biodiversity and intricately linked with the development of the genetic code itself. This relationship underscores significant transitions in the characteristics of organisms based on their survival and reproduction.
Focusing on biological evolution as a key aspect allows for a rich exploration of how genetic codes can adapt through mutation, natural selection, and genetic drift. This characteristic is beneficial because it invites discussions on the mechanisms that foster diversity within species and across ecosystems.
A unique aspect of biological evolution is its gradualism. While some changes occur swiftly via environmental pressures, most are often slow and subtle, preserving the genetic code's functionality through ages. The disadvantage, however, is that tracing back evolutionary pathways can be complex and sometimes speculative, requiring extensive fossil records and molecular data to substantiate claims. Still, this convoluted journey highlights the intricacies of life and how interconnected every organism is within the web of biological heritage.
Codon Usage Bias
Codon usage bias refers to the non-random usage of synonymous codons, which can impact protein synthesis efficiency and accuracy across different species. Understanding this phenomenon showcases how the nuances of genetic codes can vary even among closely related organisms.
Species Variability
Species variability in codon usage is a fascinating element leading to diverse adaptations. Different organisms display distinct preferences for specific codons influenced by various factors including mutational pressure, natural selection, and even temperature adaptations. This variability is a key element in understanding evolutionary relationships and gene expression patterns.
Highlighting species variability allows for a profound understanding of how closely related organisms can exhibit substantial differences in genetic language, which is beneficial for fields like phylogenetics. A unique feature is that this variability can lead to optimized protein production tailored for specific environmental conditions, enhancing survival rates. However, the major drawback is that it could complicate the development of universal genetic engineering tools, as not all codon substitutions guarantee successful protein expression.
Implications for Adaptation
The implications of codon usage bias towards adaptation are multi-layered. Variations in codon usage can significantly affect an organism's ability to thrive in changing environments. For instance, certain organisms have evolved preferences for codons that correspond to more robust protein structures under stress conditions, which is essential for survival.
One key characteristic is that an organismโs capacity to adapt generically hinges upon these subtle but impactful variations in their genetic code. This significant aspect makes it easier to explore how organisms may respond to environmental changes over time. The unique advantage of recognizing these implications lies in its application in conservation biology, helping predict how various species might cope with a changing climate.
The downside is that understanding adaptation through codon bias requires an in-depth analysis of rapid environmental shifts, which can be challenging to capture through traditional evolutionary studies. Still, grasping these implications is vitally important in modern genetics and helps outline frameworks for future research in evolutionary biology.
Genetic Code and Biotechnology
The interplay between genetic code and biotechnology is a profound field of study that reverberates through multiple scientific realms. By harnessing the understanding of DNA sequences, researchers have opened doors to innovations that not only enhance the quality of life but also raise questions about ethics and governance. This section aims to illuminate the specific elements and benefits related to the genetic code as it intertwines with biotechnology, while also considering pertinent concerns that come along for the ride.
CRISPR and Gene Editing
CRISPR, short for Clustered Regularly Interspaced Short Palindromic Repeats, has revolutionized the landscape of genetic engineering. This technology allows precise alterations to the genetic code, functioning almost like a word processor for DNA. The implications are staggering: scientists can knock out unwanted genes, correct genetic defects, and even engineer resistance to diseases in crops and livestock.
Notably, the accuracy of CRISPR means that the risk of unintended genetic changes is minimized, making it a robust tool for both research and therapeutic applications. However, with power comes responsibility. Ethical debates surrounding CRISPR focus on the potential for germline editing and the repercussions of creating โdesigner babies.โ As captivating as this technology is, discussions around its moral boundaries are vital in guiding its application.
Synthetic Biology Applications
Synthetic biology expands the boundaries of traditional biotechnology. By combining knowledge from various disciplinesโincluding genetics, engineering, and computer scienceโthis area facilitates the designing of novel organisms and enhances existing biological processes for innovative applications.
Designing Novel Organisms
Designing novel organisms is not just about creating life; itโs about tailoring organisms for specific tasks that can address pressing global challenges. For instance, scientists can engineer bacteria to produce biofuels from waste materials, enhancing sustainability. The key characteristic of this process is the ability to manipulate genetic code in a way that allows organisms to perform functions that nature did not originally design them for.
However, one must weigh the benefits against the risks; while such designs can contribute to environmental sustainability, there can be unintended ecological impacts. Itโs a delicate balance, and thus, it is crucial to proceed with caution, ensuring thorough risk assessments are conducted before releasing these organisms into the environment.
Biopharmaceutical Development
The realm of biopharmaceutical development often finds itself intertwined with synthetic biology. This aspect involves the use of living cells to carry out complex biochemical processes, yielding therapeutic products. A standout feature of this development is its ability to produce complex proteins and antibodies, which are vital for treating various diseases.
The efficiency of biopharmaceuticals makes this route particularly appealing, as it can lead to more effective therapies at a lower cost compared to traditional methods. However, the process is not without its challenges; regulatory hurdles and public perception can affect the successful implementation of these innovative treatments. Understanding the genetic code plays a central role in ensuring the manufacturing processes are optimized and safe for consumption.
"The advances in biotechnology, especially within genetic code understanding, hold the potential to transform medicine and environmental practices, but we must tread carefully to ensure ethical compliance and safety."
Ethical Considerations
Understanding the ethical considerations surrounding the genetic code is paramount in today's rapidly evolving scientific landscape. As researchers delve deeper into the secrets held within our DNA, the ramifications of their discoveries ripple through society. These factors are not merely academic; they affect the way we perceive life, health, and what it means to be human. Thus, it is vital to dissect the moral implications and societal impacts tied to gene editing technologies.
Gene Editing Ethics
Moral Implications
One cannot ignore the moral implications of gene editing technologies. These technologies, particularly CRISPR, provide unprecedented opportunities to modify organisms at their very core. While the power to potentially eliminate genetic disorders and enhance agricultural yields is tempting, we must tread carefully. The key characteristic of this discussion hinges on the notion of playing God. Some argue that altering genetic makeup alters the essence of life itself. This raises questions about the right to modify not just individual genes, but entire ecosystems. In this article, the examination of moral implications serves as a beneficial lens through which to view the potential pitfalls of unchecked technological advancement.
The unique feature here is how moral frameworks vary across cultures and societies. What may be deemed acceptable in one place could be condemned in another. Thus, the advantages of discussing moral implications include fostering a more nuanced dialogue around ethics, whereas the disadvantages involve potential stifling of innovation due to fear of backlash.
Societal Impact
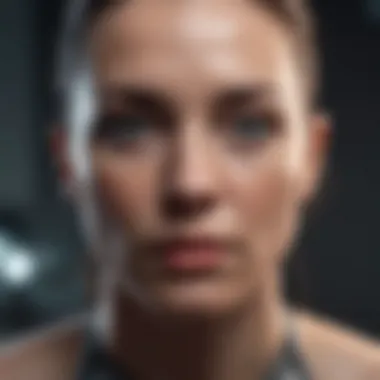
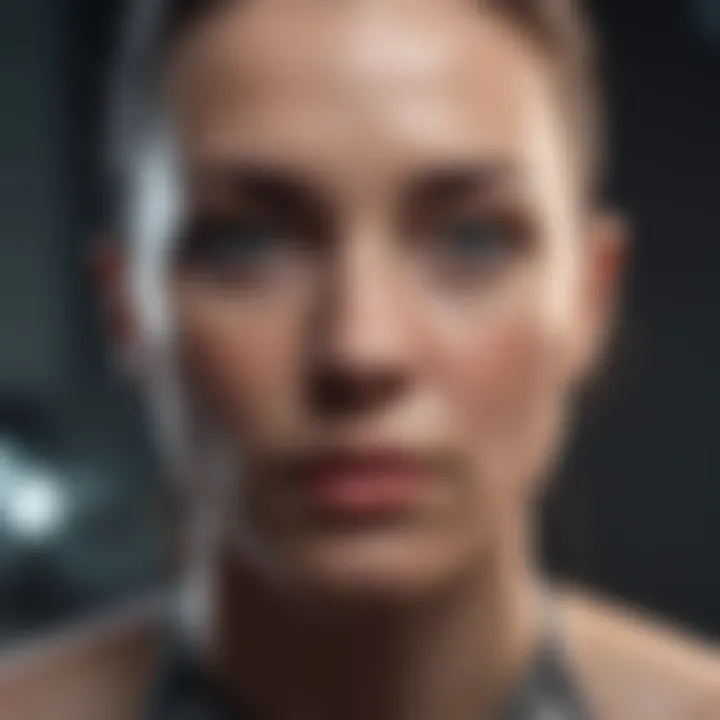
The societal impact of genetic editing technologies is profound and multi-dimensional. People's beliefs about the integrity of nature versus the benefits of technological progress are at the forefront. The key characteristic of this aspect lies in public perception, which can greatly influence research directions and funding. In this article, the exploration of societal impact sheds light on how communities react to gene editing news, whether with enthusiasm or skepticism.
Interestingly, the unique feature of societal impact is its potential to cultivate a more informed populace. Educating people about genetic code can foster trust in scientific endeavors. Yet, there are disadvantages as well; misinformation and sensational media reports can lead to misguided opinions and fears. A well-informed public can propel scientific advancements, but a misinformed one can lead to panic and distrust.
Regulation and Policy
As genetic research propels forward, so too must the frameworks that govern it. Regulation and policy stand as guiding principles ensuring that ethical considerations are met and societal impacts are accounted for.
International Guidelines
The establishment of international guidelines is crucial in promoting a unified approach toward gene editing across borders. These guidelines help in safeguarding public interest while also encouraging innovation. The key characteristic of these regulations is their emphasis on collaboration among nations, creating a framework that respects cultural values while promoting global scientific endeavors. In this article, discussing international guidelines becomes essential, as differing regulations can lead to ethical dilemmas particularly in transnational research initiatives.
One advantage of having international guidelines is that they can streamline processes, reducing confusion about what is permissible. However, potential disadvantages arise when national interests conflict with international norms, leading to discrepancies in implementation.
Public Opinion
Last but not least, public opinion plays a critical role in shaping the future of gene editing technologies. Monitoring public sentiment can guide policymakers in creating regulations that align with societal values. The key characteristic of this topic lies in its dynamism; public opinion can shift rapidly, especially in response to new scientific discoveries or ethical controversies. This article explores how trends in public opinion reflect broader cultural attitudes towards gene editing.
The unique feature of public opinion is that it provides a real-time perspective on societal acceptance or rejection of advancements in genetic technologies. Engaging the public has its advantages, promoting meaningful dialogue between scientists and citizens; yet it also has notable disadvantages if dominant voices overshadow minority viewpoints, creating a skewed narrative surrounding the implications of genetic manipulation.
The intersection of ethics and genetics is not merely theoretical; it shapes the decisions we make today regarding our future.
Through this analysis, readers can gain a nuanced understanding of the myriad ethical considerations embedded within the frameworks of gene editing and the intricate dance between science, society, and morality.
Future Directions in Genetic Research
The landscape of genetic research is undergoing rapid transformation as scientists chase the proverbial carrot of better understanding and manipulating life at its molecular roots. By looking ahead, we can identify critical avenues shaping advancements in genetics, which can influence various fields from medicine to agriculture. These trajectories not only promise growth but they also raise questions about the ethical implications and societal responsibilities that come along for the ride. In doing so, we venture into two major topics: emerging technologies and the potential impacts on medicine.
Emerging Technologies
Next-generation Sequencing
Next-generation sequencing represents a pivotal leap forward in genomic analysis, far surpassing traditional methods in terms of speed and capability. Its prime attribute is the ability to decode millions of DNA strands concurrently, facilitating comprehensive insights into genetic variations across populations. This method is particularly appealing since it reduces costs significantly compared to its predecessors, thus enabling a broader demographic engagement in genomic studies.
A unique feature of next-generation sequencing is its high-throughput capability, which allows researchers to gather vast amounts of data quickly. This aspect is especially beneficial in the aforementioned article because it accelerates our understanding of genetic codesโbe it for identifying mutations that may lead to diseases, or for exploring evolutionary relationships among species. However, the sheer volume of data brings its challenges, notably in processing and interpreting this information accurately.
Machine Learning in Genomics
Machine learning is carving out its niche in genomics, pushing the boundaries of how we analyze genetic data. By employing algorithms that can learn patterns without explicit programming, machine learning proves invaluable in sifting through complex genomic datasets to spotlight potential biomarkers for diseases or therapeutic targets. The attractiveness of this choice lies in its adaptability, allowing it to refine itself as additional data becomes available.
This technology's standout feature is its predictive capability. By assessing vast datasets, machine learning can often predict outcomes that a human analyst might miss. In respect to the overarching topic of this article, machine learning offers a promising pathway to decipher the intricacies hidden within the genetic code, thus propelling the frontier of personalized medicine forward. Yet, there are considerations such as the potential for algorithmic bias, prompting the need for critical scrutiny in how these models are trained and validated.
Potential Impacts on Medicine
Personalized Medicine
Personalized medicine is the concept of tailoring medical treatment to the individual characteristics of each patient's genetic makeup. This area is revolutionizing healthcare, allowing for more effective treatments based on a deeper understanding of how disorders affect different people based on their genetic profiles. The crowning feature of personalized medicine is its capacity to provide targeted therapies, substantially reducing the trial-and-error nature of conventional treatment methods.
In the context of this article, the implementation of personalized medicine emphasizes that the genetic code is not merely a theoretical construct but a practical tool. Unique features such as genomic profiling can unearth predispositions to certain conditions, potentially leading to preventive measures or customized interventions. However, one must tread carefully, as the implications surrounding data privacy and genetic discrimination are critical topics of ongoing debate.
Gene Therapy
Gene therapy marks a significant stride in managing genetic disorders by introducing, removing, or altering genetic materials within a patient's cells. It holds the promise of treating disorders that were previously deemed untouchable, like cystic fibrosis or sickle cell anemia. The key characteristic that makes gene therapy appealing is its potential to address root causes of diseases, leading to long-lasting effects rather than merely alleviating symptoms.
This innovative approach's unique flair lies in its ability to engineer genetic enhancements, paving the way for exciting prospects in both treatment and possibly enhancement. While gene therapy's benefits are enticing, challenges abound, including delivery methods, the potential for immune responses, and the ethical considerations inherent in altering human genetics. Therefore, the lens through which we scrutinize gene therapy must account for wider societal implications, alongside the scientific marvels it promises.
In summary, the future directions in genetic research are laden with both potential and responsibility. As we forge ahead into this uncharted territory, the interplay between technology, medicine, and ethics will dictate the path we take.
The End
The concluding part of this article emphasizes the significance of understanding the genetic code in various scientific domains. The insights derived from the study of the genetic code are essential for multiple fields, ranging from molecular biology to personalized medicine. By grasping its intricacies, researchers and practitioners can unlock potential advancements that may redefine therapeutic approaches and disease management.
Moreover, comprehending the genetic code provides a framework for addressing fundamental questions about life itself, how it evolved, and the mechanisms that underpin various physiological processes. As advancements in technology continue to progress, the implications of manipulating this codeโwhether through CRISPR or other gene-editing toolsโraise new ethical considerations that must not be overlooked. These discussions are increasingly relevant in the face of rapid developments in biotechnology, highlighting the need for responsible practices in genetic research.
Summary of Key Points
- The genetic code is fundamental in dictating protein synthesis, a process central to all life forms.
- Understanding the historical development of the genetic code allows us to appreciate its evolutionary significance.
- The intricacies of codons, base pairing, and nucleotides play critical roles in gene expression.
- Biotechnology applications, such as CRISPR, exemplify the practical implications of genetic research in modern medicine.
- Ethical considerations surrounding gene editing emphasize the balance between innovation and moral responsibility.
Final Thoughts
Reflecting on the discussions throughout this article, it's clear that the genetic code is more than just a series of nucleotides. It serves as a blueprint for life, holding the key to both current biological understanding and future possibilities. As we navigate the complexities of genetic research, fostering an informed dialogue surrounding the ethical implications will be essential. The pursuit of knowledge in this area doesn't just advance science; it also challenges society to consider the broader impact of those advancements. The message is evident: a deep understanding of the genetic code could well shape the future of humanity.