Understanding High Throughput Screening Assays in Research
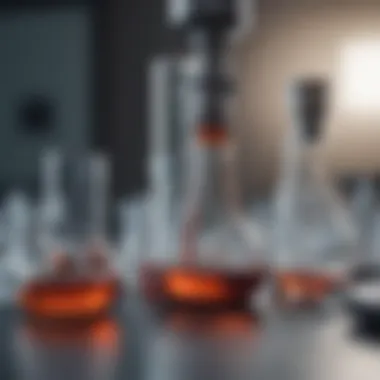
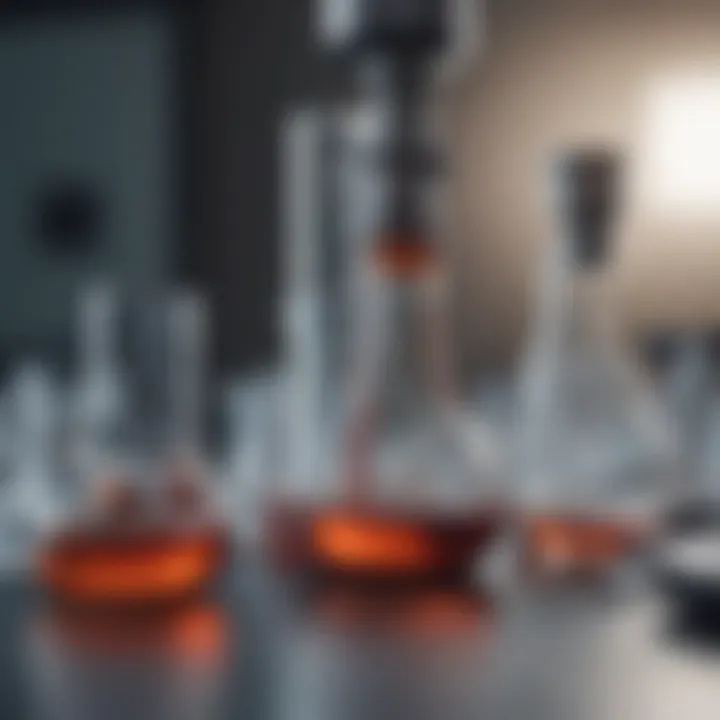
Intro
High throughput screening (HTS) assays revolutionize the landscape of drug discovery. These techniques enable the rapid testing of thousands of compounds, which accelerates the identification of potential drug candidates. Researchers utilize HTS to streamline drug development processes, optimizing efficiency and time management.
The principles underlying HTS involve meticulous planning and execution. The studies employ diverse methodologies to enhance compound screening. Understanding these setups and techniques is vital for students and professionals seeking to engage deeply with biochemistry and pharmaceutical development.
In this article, we will explore the methodologies and applications related to HTS assays. We will also discuss the key findings along with challenges and optimization strategies. Altogether, this creates a comprehensive framework for understanding HTS at various levels of complexity.
Research Overview
Summary of Key Findings
High throughput screening has fundamentally transformed the approach to drug discovery. Key findings indicate:
- HTS can evaluate thousands of compounds in a short timeframe.
- Various assay formats, such as biochemical and cellular assays, are prevalent in HTS.
- Data generated helps in identifying lead candidates for pharmaceutical development.
Methodologies Employed
Different methodologies are integral to HTS. Some prominent ones include:
- Fluorescence-based assays: Utilize fluorescent markers to measure interactions or activity.
- Luminescence assays: Detect light emitted from reactions, often signaling compound activity.
- Mass spectrometry: An analytical technique used to identify compounds based on mass-to-charge ratios.
Each methodology has strengths and weaknesses, impacting compound screening outcomes. It is essential to select an appropriate method according to research objectives.
In-Depth Analysis
Detailed Examination of Results
Results derived from HTS provide detailed insights into compound efficacy and safety. By analyzing data patterns, researchers can identify promising candidates. Assay results reveal how compounds interact with biological targets, leading to the development of effective drug therapies.
Furthermore, identifying false positives and negatives in data is critical, as these can lead to misinterpretation of compound behavior. Scientists often use advanced statistics and bioinformatics tools to enhance result validity.
Comparison with Previous Studies
When examining HTS methodologies, contrasting current research findings with earlier studies showcases the evolution of these techniques. While early forms of screening were labor-intensive, modern HTS processes employ automation. Advances in robotics and data analysis allow for greater throughput and accuracy, making it easier to sift through compound libraries.
The transition from manual to automated processes has led to more robust data quality, thereby facilitating better drug discovery outcomes.
"The integration of automation in HTS has drastically cut down the time taken for compound evaluation, enabling faster delivery of potential therapies to the market."
In summary, understanding the methodologies and applications of high throughput screening assays provides essential knowledge for anyone involved in scientific research. This article aims to connect students and professionals alike with the information necessary for informed engagement in the field.
Prologue to High Throughput Screening Assays
High throughput screening (HTS) assays are now a prominent part of scientific investigation, especially in drug discovery. Their significance lies in the ability to test thousands of samples quickly, identifying potential drug candidates efficiently. Notably, HTS allows researchers to make data-driven decisions, which is crucial for advancing therapeutic approaches in various diseases.
HTS has transformed the landscape of biological testing. With the rapid advancement of technology, researchers can now harness automation and sophisticated detection techniques to facilitate screening processes. This rapid testing can significantly decrease the time and cost associated with finding new drug candidates, making it a invaluable approach in modern biomedicine. As a result, understanding HTS is not only important for professionals in the field but also for educators and students who aim to engage with contemporary research methodologies.
Through this article, we will explore the intricacies of HTS assays. We will discuss their definitions, historical context, key components, methodologies, applications, challenges, and future trends. By delving into these aspects, readers can gain profound insights, enriching their comprehension of high throughput screening in scientific research and its far-reaching implications.
Definition and Importance of HTS
High throughput screening refers to the methodical evaluation of numerous biological compounds for their effect on a particular target, such as a protein or a gene. This method utilizes automated techniques, allowing for rapid processing of large datasets. The importance of HTS is underscored by its capabilities: it accelerates drug discovery, enhances the identification of lead compounds, and streamlines the entire research process.
Key benefits of HTS include:
- Efficiency in testing large libraries of compounds.
- Reduction in time spent on preliminary screening, providing faster outcomes.
- Increased accuracy due to automated processes, minimizing human error.
Moreover, HTS plays a vital role in pharmacogenomics, potentially leading to improved personalized treatment approaches in healthcare. Its implications extend beyond drug discovery, also touching upon various aspects of biology, including gene function studies and biomarker identification.
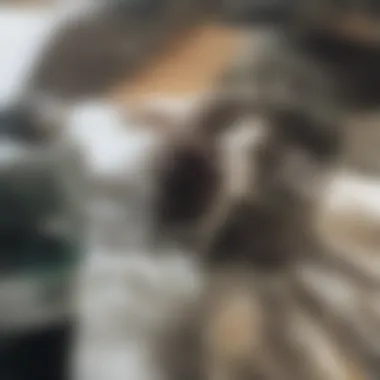
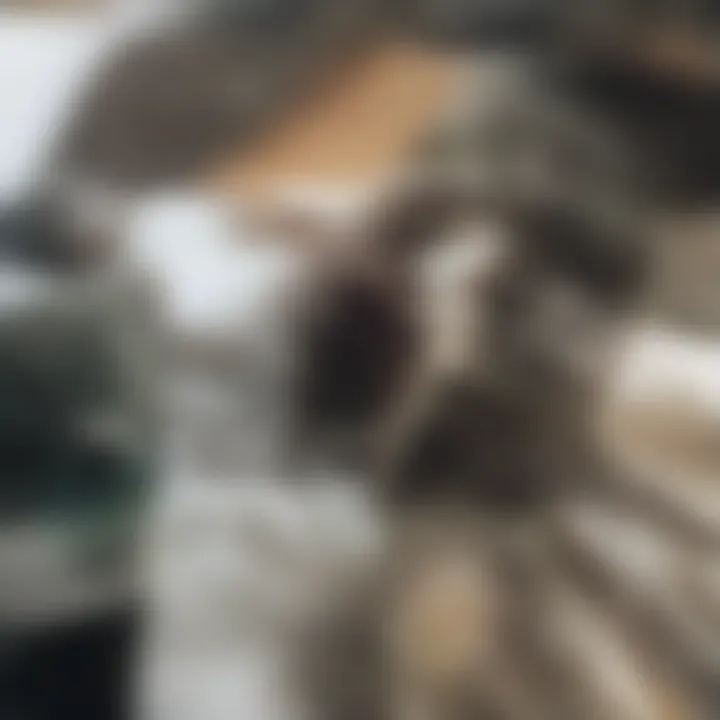
Historical Background
The roots of high throughput screening can be traced back to the late 20th century, when the demand for faster drug discovery processes became apparent. Initially, screening methods were predominantly labor-intensive and slow. The introduction of robotics and computer technologies laid the groundwork for the evolution of HTS.
In the 1990s, significant advances were made, with institutions like Eli Lilly and Amgen leading the charge. The adoption of miniaturized assay formats and microplate technology facilitated the testing of thousands of compounds with minimal reagent costs. As scholars and industries recognized the potential, HTS methodologies gained momentum, becoming an industry standard.
Today, HTS continues to evolve at a remarkable pace. Ongoing developments between technology and biological understanding enhance the ability and accessibility of these assays, establishing them as a cornerstone of modern scientific research in drug development and beyond.
Key Components of HTS Assays
High throughput screening (HTS) assays rely on several crucial components that collectively enhance their efficiency and effectiveness. Understanding these components is essential for maximizing the potential of HTS in various research applications, particularly in drug discovery. The three main elements include reagents and compound libraries, automation and robotics, and detection methods. Each plays a significant role in shaping the outcomes of HTS experiments and overcoming the challenges associated with large-scale biological testing.
Reagents and Compound Libraries
Reagents and compound libraries are fundamental to any HTS process. The selection of appropriate reagents can significantly influence the specificity and sensitivity of the assay. Compound libraries, which consist of a vast array of small molecules, are screened to identify potential drug candidates. These libraries should be diverse enough to cover a wide range of chemical space and biological activity.
The significance of high-quality reagents cannot be overstated. They should demonstrate stability and reproducibility in their performance. Factors such as purity and concentration must also be considered. Furthermore, the availability of the compound libraries for specific targets is critical. This ensures that researchers can test a broad spectrum of candidates against the desired biological target, thus increasing the likelihood of finding effective compounds.
Automation and Robotics in HTS
Automation and robotics have transformed HTS, enabling laboratories to perform numerous assays quickly and accurately. Automation minimizes human error and increases throughput, allowing scientists to focus on analyzing results instead of executing repetitive tasks. Robotic platforms perform liquid handling, plate washing, and other essential functions with remarkable precision.
The integration of automation in HTS also allows for a higher degree of standardization. This consistency is vital for reproducibility, a key aspect of experimental validation in research. Moreover, robotic systems can manage a variety of tasks simultaneously, which can significantly reduce the time required for assay completion. Such efficiency is invaluable in the competitive field of drug discovery.
Detection Methods
Detection methods are critical for interpreting the results of HTS assays. They determine how signals generated during screening are quantified and analyzed. Generally, three primary detection methods are heavily employed: fluorescence, luminescence, and colorimetric assays.
Fluorescence
Fluorescence detection is widely used in HTS because of its sensitivity and ability to measure low concentrations of analytes. This method relies on detecting emitted light from fluorescent molecules. One key characteristic of fluorescence is its high signal-to-noise ratio, making it suitable for various applications. Additionally, many fluorescent assays can be multiplexed, allowing simultaneous detection of multiple targets in a single experiment.
However, fluorescence assays may face challenges such as photobleaching, which can diminish signal strength over time. To mitigate this, careful experimental design and appropriate selection of fluorescent probes are necessary.
Luminescence
Luminescence assays involve detecting light produced by a chemical reaction, which makes them highly sensitive and suitable for HTS applications. A distinct advantage of luminescent methods is that they do not require external light sources, which eliminates background noise and enhances signal detection.
Despite these advantages, one drawback of luminescence detection can be the transient nature of the signal. This requires careful timing of data acquisition to capture peak luminescence. Moreover, luminescent assays often require specific reagents that may complicate the assay setup.
Colorimetric
Colorimetric detection determines the concentration of an analyte by measuring changes in color intensity during a reaction. This method is simple, cost-effective, and straightforward, making it accessible for many laboratory settings. A key characteristic of colorimetric assays is their ease of use, as they often do not require advanced equipment for analysis.
However, colorimetric methods are generally less sensitive than fluorescence and luminescence. This can limit their applicability in scenarios demanding high sensitivity. Additionally, they may be affected by the presence of interfering substances that alter color readings, which is a consideration during experimental design.
In summary, the components of HTS assays are interconnected, each contributing to the overall functionality and success of the screening process. By optimizing reagents, embracing automation, and selecting appropriate detection methods, researchers can enhance the effectiveness of HTS in drug discovery and related fields.
HTS Methodologies
High throughput screening methodologies play a crucial role in the realm of drug discovery and biochemical analysis. These methodologies provide a framework for systematically testing thousands of samples quickly and efficiently. By adopting such approaches, researchers can identify promising candidates for further development. They can also optimize processes to improve overall efficacy and accuracy in data collection.
Cell-based Assays
Cell-based assays are fundamental in high throughput screening as they reflect the biological context of drug action. These assays utilize live cells to evaluate the effects of compounds in a biologically relevant environment. This allows researchers to assess not only the potency of the compounds but also their selectivity and potential toxicity.
A common type of cell-based assay is the reporter gene assay, which measures gene expression following compound administration. Another example is the cell viability assay, often used to determine the cytotoxic effects of drugs. The benefits of using cell-based assays include:
- Relevance: Providing insights into cellular responses that in vitro biochemical assays may not capture.
- Complexity: Allowing researchers to study multifactorial biological responses to compounds.
- Quantitative data: Enabling high-resolution data via automated imaging and analysis tools.
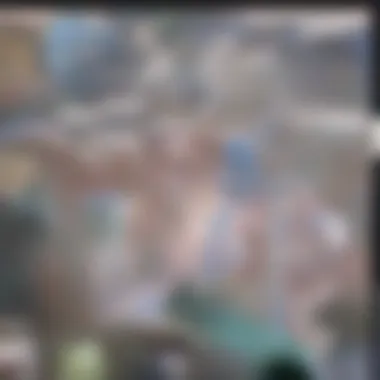
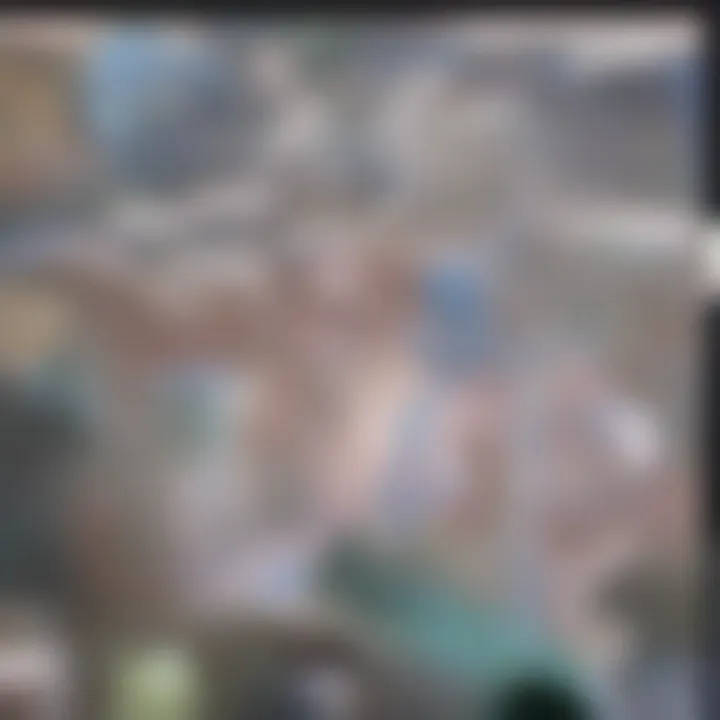
Despite their advantages, challenges persist. Cell culture conditions must be optimized to ensure reproducibility. Factors such as cell line selection and growth conditions can significantly impact the results.
Biochemical Assays
Biochemical assays are another pillar of high throughput screening. These assays focus on biochemical interactions rather than living cells. For example, they measure enzyme activity, ligand-receptor interactions, or binding affinities of small molecules to target proteins.
One of the most common biochemical assays is the enzyme-linked immunosorbent assay (ELISA), used extensively for detecting and quantifying proteins. Another well-established method is the Western blotting, which allows for protein identification and quantification. The advantages of biochemical assays include:
- Simplicity: Generally easier to control and replicate compared to cell-based assays.
- Rapid results: Can provide quicker results in a controlled environment.
- Specificity: High specificity for target interaction, minimizing potential interference from cellular components.
However, biochemical assays can sometimes overlook the dynamics seen in live cells, leading to discrepancies in translational relevance in drug discovery.
Label-free Technologies
Label-free technologies represent a more modern approach to high throughput screening. These methods do not require the use of fluorescent or radioactive labels, which can alter the biological interactions being studied. Label-free techniques utilize various physical phenomena, including mass change, optical interference, or impedance, to assess molecular interactions in real time.
One popular label-free method is surface plasmon resonance (SPR), which allows for the measurement of binding kinetics without labeling molecules. Other methodologies include acoustic wave biosensors and optical waveguides. The benefits of label-free technologies include:
- Maintenance of natural interactions: Preserving the true biological state of interactions.
- Real-time data: Allowing continuous monitoring of interactions, leading to a better understanding of dynamics.
- Broad application: Suitable for a variety of assays involving proteins, peptides, and small molecules.
Nonetheless, these methods can be technically complex and may require a significant investment in instrumentation and training.
High throughput screening methodologies, from cell-based assays to biochemical assays, each offer unique insights and benefits that contribute significantly to the drug discovery process.
Applications of HTS
High throughput screening (HTS) plays a pivotal role in research, especially in the fields of drug discovery and biochemical analysis. The applications of HTS are diverse, touching upon various critical components of scientific advancement. Understanding these applications is essential for grasping the significance of HTS in modern laboratories.
Drug Discovery and Development
The foremost application of HTS lies in drug discovery and development. In this context, HTS facilitates the rapid evaluation of large libraries of compounds against specific biological targets. This accelerates the identification of promising drug candidates significantly. Traditional methods of screening drugs were time-consuming and labor-intensive. HTS, however, allows researchers to run thousands of tests simultaneously, which enhances efficiency.
The benefit of HTS in drug discovery is profound. For instance, pharmaceutical companies can identify compounds that show bioactivity at an early stage of research. This reduces the overall time and cost associated with bringing new drugs to market. An example is the use of HTS in antiviral drug development, where it has been essential in identifying inhibitors that target viral replication mechanisms.
Furthermore, the integration of sophisticated data analysis tools in HTS enables the identification of structure-activity relationships. It allows researchers to understand how the chemical structure of a compound influences its biological activity, paving the way for rational drug design.
Target Identification and Validation
Another significant application of HTS is in target identification and validation. Identifying new biological targets is crucial in developing therapeutic interventions. Using HTS, researchers can screen for potential targets by observing the interactions between compounds and various proteins or genes.
High throughput technologies allow for the exploration of vast biological landscapes. In studies related to cancer, for example, HTS is used to identify novel targets that can be modulated to alter disease progression. Validating these targets is equally essential, and HTS assists in confirming the role of these targets in biological processes. This step is critical for ensuring that future therapeutic agents will have the desired effects in clinical settings.
Through HTS, the relationship between a target and its modulators can be established. Understanding these relationships is key for both drug discovery and therapeutic applications, resulting in a more focused approach to developing interventions.
Predictive Toxicology
With rising concerns about the safety of drugs, predictive toxicology has emerged as another vital application of HTS. HTS can be used to assess the potential toxicity of compounds early in the discovery process. By screening compounds using various HTS assays designed to detect toxicological effects, researchers can identify unfavorable candidates before entering costly clinical trials.
The implementation of predictive toxicology in HTS enhances drug safety significantly. It can predict how a drug may behave in the body or its potential side effects, thus improving the overall drug development process. Common endpoint evaluations involve cellular viability assays and cytotoxicity assays, which assess the harmful effects of different compounds.
In summary, the applications of HTS extend beyond mere drug discovery. From identifying and validating new biological targets to enhancing predictive toxicology, HTS presents a multi-faceted approach that can transform modern scientific research. The potential benefits of integrating HTS methodologies into research pipelines are vast, ultimately leading to safer and more effective therapeutic solutions.
Challenges in HTS Assays
High throughput screening (HTS) assays have transformed drug discovery and biomedical research. However, several challenges can hinder their efficiency and accuracy. Understanding these challenges is crucial for optimizing the technology and ensuring reliable outcomes in scientific studies. This section will explore various challenges, focusing on three primary areas: data management and analysis, false positives and negatives, and sample handling and reproducibility. Each of these elements plays a significant role in the overall effectiveness of HTS.
Data Management and Analysis
Efficient data management is essential for any HTS assay. The sheer volume of data generated during screenings can be overwhelming. Advanced computational tools are required to store, process, and analyze this data effectively. Without proper data management strategies in place, researchers may encounter difficulties in extracting meaningful insights.
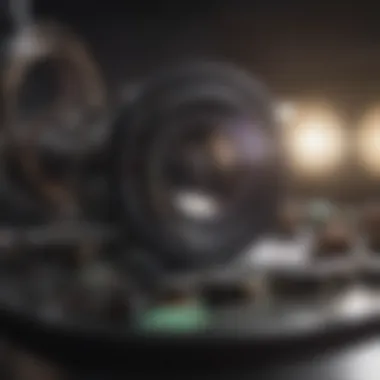
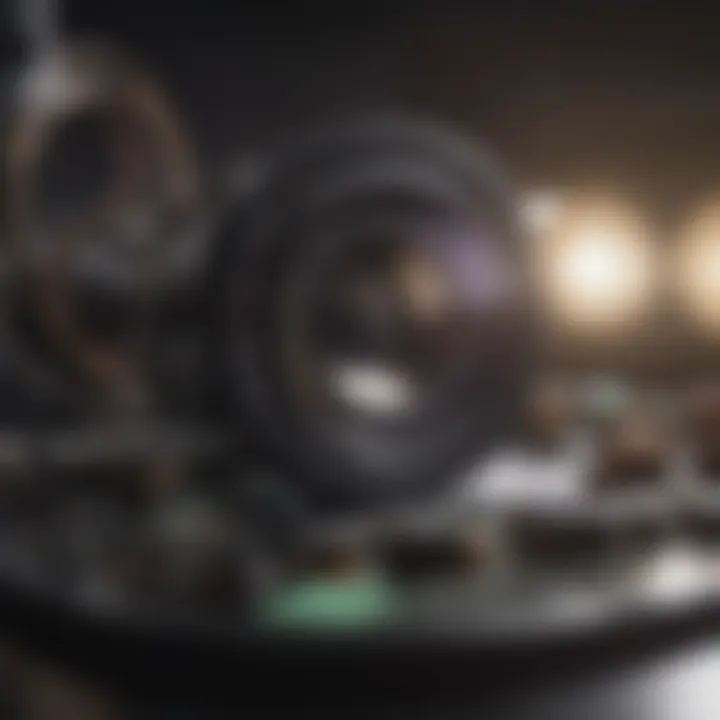
Data from HTS can be complex and multifaceted. Thus, robust analysis techniques are necessary to decipher the information. Researchers need to employ statistical methods that ensure accuracy and reliability in their results. Moreover, leveraging software tools can facilitate data normalization and correction for systematic errors, which is critical for comparing results across different assays.
Automating data management helps to mitigate human error and improve the speed of analysis. Yet, it also requires significant investment in technology and training. Therefore, organizations must weigh the benefits of automation against these costs.
False Positives and Negatives
An inherent challenge in HTS assay results is the occurrence of false positives and false negatives. False positives can lead researchers to incorrectly identify compounds as viable drug candidates when they are not. This can waste valuable time and resources on further studies that may not yield any real results. On the other hand, false negatives can cause researchers to overlook potentially promising compounds, stifling innovation in drug discovery.
To reduce these errors, researchers employ various strategies. Running confirmatory assays is one approach. Conducting secondary assays and using orthogonal methods can help validate initial findings. Additionally, implementing stringent hit criteria can also minimize the occurrence of such inaccuracies.
Sample Handling and Reproducibility
Sample handling is a critical aspect of HTS assays that directly impacts reproducibility. Proper sample preparation, storage, and handling are vital to ensure that results are consistent across different experiments. Variations in temperature, pH, and timing can alter the outcomes of assays. It is essential to establish standard operating procedures for sample handling to maintain the integrity of results.
Moreover, reproducibility is a cornerstone of scientific research. Lack of reproducibility can lead to skepticism around findings and discourage further research. To enhance reproducibility, researchers must document all procedures meticulously. Collaboration between laboratories can be beneficial in validating results and ensuring consistency across studies.
"Reproducibility is not just a requirement; it is a pillar upon which the validity of scientific work stands."
Future Trends in HTS
The evolving landscape of high throughput screening (HTS) is significantly shaped by emerging technologies and methodologies. Keeping abreast of these future trends is essential for researchers and professionals alike. They reflect not only on how HTS is utilized today but also on its potential to enhance productivity and effectiveness in scientific research and drug development.
Integration of Artificial Intelligence
Artificial intelligence (AI) is becoming a pivotal player in managing and analyzing vast data generated from HTS assays. By utilizing machine learning algorithms, researchers can identify patterns and make predictions with much greater accuracy than traditional statistical methods. This means that the analysis of results will be much quicker and more relevant to target identification.
- Improved Data Interpretation: AI can sift through complex datasets faster, reducing the time to identify promising compounds.
- Predictive Modeling: Machine learning can predict how compounds will behave in biological systems, thus streamlining the screening process.
This will lead to fewer experiments being necessary, freeing up resources for more innovative research.
However, several considerations arise from integrating AI. Data integrity, model validation, and transparency are vital to maintain research credibility.
Personalized Medicine Applications
Personalized medicine is at the forefront of modern healthcare. Integrating HTS in this field allows for the identification of specific biological targets that vary among individuals. As we move toward more tailored treatment plans, HTS plays a crucial role in this transition.
- Targeted Drug Development: By screening compounds against patient-derived cells, researchers can discover which treatments might be most effective for individual patients.
- Biomarker Discovery: HTS can assist in identifying biomarkers, enhancing the understanding of disease mechanisms essential for developing personalized therapies.
The convergence of HTS and personalized medicine presents the opportunity to develop drugs that align more closely with individual genetic profiles, ultimately increasing their effectiveness and minimizing adverse effects.
Miniaturization of Assays
Miniaturization represents another significant trend in HTS. As assay volumes and materials decrease, researchers can conduct more experiments using less reagent. This fosters scalability and cost efficiency.
- High-density Plates: The use of microtiter plates with smaller wells allows for testing a larger number of compounds simultaneously.
- Reduced Costs: Less reagent usage not only cuts costs but also minimizes environmental impact.
Nevertheless, the miniaturization process comes with challenges. Maintaining assay sensitivity and reproducibility can be difficult as volumes decrease. Therefore, optimizing these aspects is critical for the success of miniaturized platforms.
Closure
High throughput screening (HTS) assays play a critical role in scientific research and drug development. Their importance lies in an accelerated process that enables the rapid testing of thousands of compounds to identify potential drug candidates. Here, we look at several key points concerning the conclusion of our discussion on HTS:
- The significance of HTS cannot be overstated. It transforms the drug discovery process, making it more efficient and cost-effective. The ability to screen numerous compounds in a limited timeframe allows researchers to focus on the most promising candidates sooner than traditional methods would allow.
- Integration of advanced technologies such as automation and data analytics enhances HTS capabilities. These innovations allow researchers to not just collect data but analyze it intelligently, yielding insights that might otherwise be overlooked.
- Challenges remain in HTS assays, particularly concerning data management and the potential for false positives. Addressing these issues is vital for ensuring the reliability of results obtained from HTS processes. Continuous improvement in methodologies and technologies is essential to mitigate these challenges.
"HTS assays facilitate the identification of viable drug candidates, fundamentally altering the research landscape in biomedical sciences."
The Role of HTS in Future Research
As we look forward, high throughput screening will become even more integral to future research efforts. The advancements in technology will pave the way for more sophisticated assays that provide deeper insights into biological mechanisms. HTS allows scientists to explore drug interactions and effects at unprecedented scales, ultimately leading to more targeted therapies.
Moreover, as we personalize medicine, HTS will help researchers tailor treatments to specific genetic profiles or disease states. The flexibility of HTS methodologies makes them suitable for various applications beyond drug discovery, such as exploring disease pathways and environmental interactions.
Final Thoughts
In summation, high throughput screening stands out as a pivotal innovation within the scientific community. It not only streamlines the drug discovery phase but also opens new avenues for research. The discussion outlined in this article serves to emphasize how essential HTS is for aspiring researchers and established professionals alike. With continuous advancements, the future of HTS looks promising, heralding a new era in biomedicine and pharmacology.