Focused Ion Beam: Principles and Future Trends
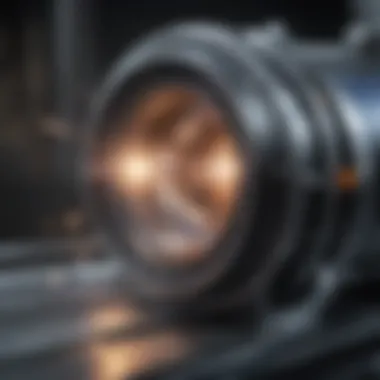
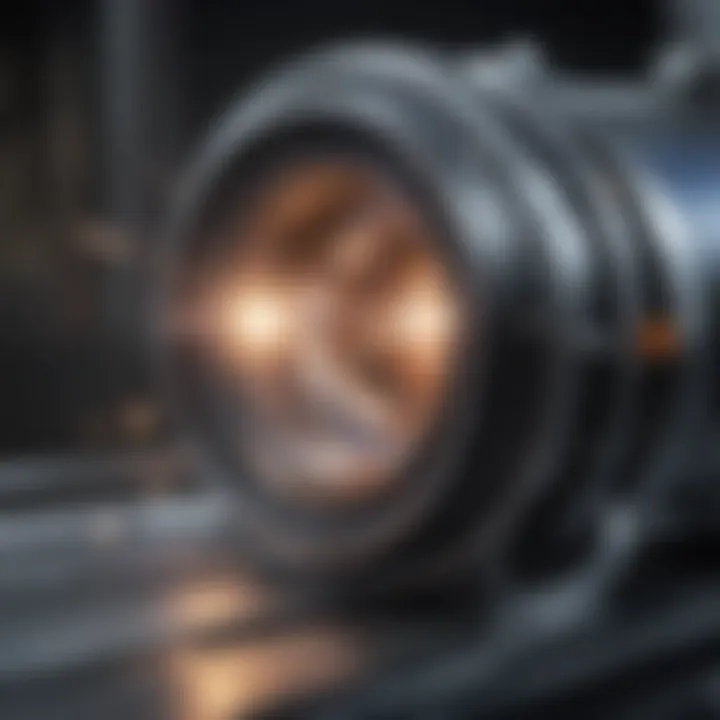
Intro
Focused Ion Beam (FIB) technology has become indispensable in various fields, particularly in materials science and semiconductor fabrication. At its core, FIB enables the precise manipulation and analysis of materials at the nanoscale. This technology leverages the interaction of ions with matter to facilitate imaging, milling, and deposition processes. Understanding FIB involves not just the principles that govern ion behavior but also the sophisticated equipment and methodologies employed in its application.
As industries push towards greater miniaturization and the need for enhanced functionality, the relevance of FIB technology grows. Researchers and professionals alike are tasked with mastering these nuances to stay at the forefront of scientific and industrial advancements.
In this article, we aim to dissect the principles underpinning FIB technology, explore its diverse applications, and speculate on its future directions.
Research Overview
Summary of Key Findings
FIB technology plays a crucial role in enabling high-resolution imaging and the customization of materials. Key findings from recent studies indicate that FIB can achieve resolutions down to the atomic level, making it a vital tool for nanotechnology. Additionally, advancements in FIB systems allow for real-time imaging and analysis, which significantly enhances research and application processes.
Methodologies Employed
The methodologies surrounding FIB technology typically involve:
- Ion beam generation and focusing
- Sample preparation techniques
- Analysis using various imaging methods
- Data interpretation and simulation
These methodologies not only allow for precision but also facilitate a deeper understanding of material properties and behaviors.
In-Depth Analysis
Detailed Examination of Results
The results from utilizing FIB technology reveal the profound capabilities in tailoring materials. For instance, in semiconductor fabrication, FIB assists in creating intricate patterns that are essential for microchips. The technology has also proven effective in materials science, where it aids in the development of new composites for various applications.
Comparison with Previous Studies
When compared to previous studies, the improvements in FIB technology underscore a noticeable evolution in methodology. Early studies primarily focused on basic imaging capabilities. However, recent findings emphasize advanced applications such as vaccine development and biological imaging. This shift illustrates the growing value of FIB in interdisciplinary research.
"FIB technology remains at the forefront of innovation, driving advancements that span multiple scientific domains."
Prolusion to Focused Ion Beam Technology
The introduction of focused ion beam (FIB) technology marks a significant advancement in material analysis and modification. FIB systems are pivotal in a wide range of applications, from semiconductor manufacturing to nanotechnology. Understanding FIB technology is essential for researchers and professionals engaged in advanced materials science and engineering fields. This section lays the foundation for comprehending how FIB operates and its extensive importance across various domains.
Definition and Overview
Focused ion beam (FIB) technology refers to a highly specialized technique that utilizes accelerated ions to scan and irradiate a targeted area on a substrate. It operates similarly to scanning electron microscopy (SEM), yet it uses ions instead of electrons. This difference allows FIB to manipulate materials at the atomic level, enabling precise structuring and modification.
Some key characteristics of FIB technology include:
- High Precision: FIB systems can create structures with nanometer scale accuracy.
- Versatility: It can be utilized for tasks such as milling, deposition, and imaging.
- Material Analysis: FIB facilitates in-depth analysis of material properties and compositions.
The ions generated in a FIB system are typically Gallium, though other elements can be employed depending on the desired application. Following acceleration, ions are focused into a beam, which is then directed at the sample. The ion beam interacts with the material, leading to various physical and chemical effects.
Historical Development
The evolution of focused ion beam technology can be traced back to the 1970s. Initially, the notion of using ions for imaging surfaced, but early systems were mostly experimental. The first commercial FIB systems were developed in the 1980s, which marked a turning point in both semiconductor fabrication and materials analysis.
Over the years, advancements in ion optics, fabrication techniques, and ion source technologies have significantly enhanced the capabilities of FIB systems. Today, FIB technology is integrated with other analytical methods, such as scanning electron microscopy (SEM), providing a more comprehensive toolkit for scientists. This interdisciplinary approach reflects an ongoing trend towards hybrid technologies in materials research and fabrication.
Fundamental Principles of Focused Ion Beams
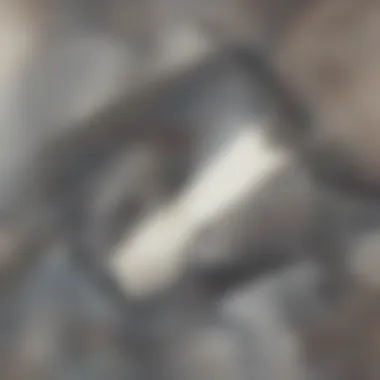
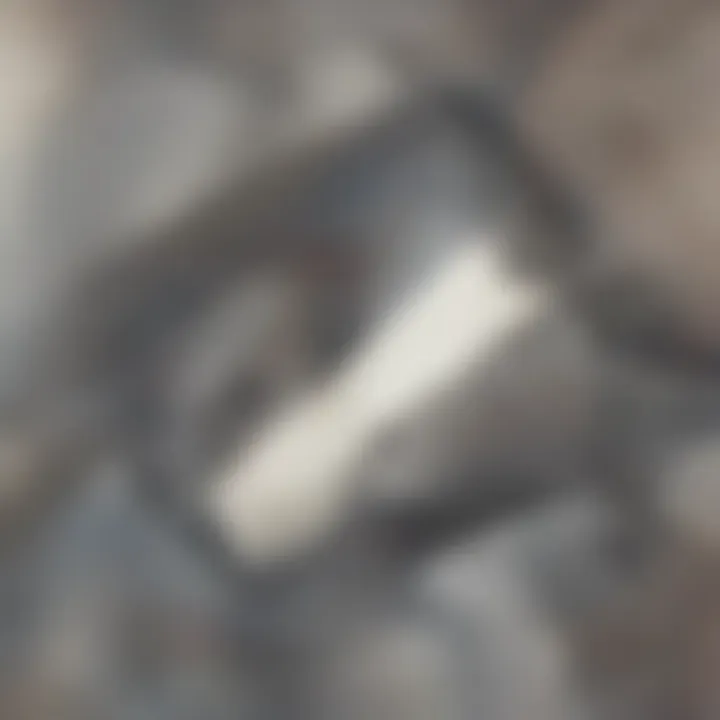
Understanding the fundamental principles of focused ion beams (FIB) is critical for grasping their capabilities and applications in diverse scientific fields. The principles involved dictate not only how ion beams interact with materials but also how they are manipulated for precision tasks. These principles are rooted in the physics governing ion beam technology, governing their effective use in various applications, from the nano-scale to larger materials science initiatives.
Ion Beam Physics
Ion beam physics emphasizes the behavior and properties of charged particles when they are accelerated and focused. An ion is an atom that has lost or gained one or more electrons, acquiring a net charge. Basics of ion beam physics include understanding the characteristics such as mass, charge, and energy of ions. When ions are directed towards a target, their kinetic energy plays an essential role in determining the outcome of the interaction.
The mass-to-charge ratio is particularly significant, as it influences how ions behave in electric and magnetic fields. Lighter ions, for example, can be accelerated at lower voltages compared to heavier ions to achieve similar energy levels. This forms the basis for different applications; selecting an appropriate ion species is crucial for achieving desired effects in material modification or removal.
In FIB systems, ions such as gallium or indium are frequently used. Their high mass-to-charge ratios facilitate better penetration and interaction with various materials. This characteristic allows for detailed analysis at the nano-scale.
Ion beam physics is not just theoretical; it lays the groundwork for innovations in manufacturing, diagnostics, and research-based projects.
Acceleration and Focusing Mechanisms
The process of acceleration and focusing is fundamental for optimizing ion beam performance. Once ions are generated in an ion source, they must be accelerated to the appropriate energy levels using high voltages. This step is pivotal, as insufficient acceleration results in poor depth penetration and ineffective material modification.
The focusing mechanism is implemented through electromagnetic lenses. These lenses are designed to converge the ion beam onto a precise point on the sample. The interaction of electric and magnetic fields allows for fine control over the beam's diameter and direction. Precise control of beam size is vital for ensuring high resolution, particularly in applications that require nanoscale precision.
Effective focusing minimizes beam spread, which is crucial in processes such as ion milling or etching. It allows for a greater degree of accuracy during sample analysis and modifications, leading to desired morphological changes or detailed imaging results.
Interaction of Ions with Matter
Ion interaction with matter involves complex physical phenomena that can lead to various effects, including displacement, sputtering, and implantation. When ions collide with a target material, they transfer energy to the atoms in the material, causing a cascade of atomic displacement.
Sputtering occurs when the energy transferred by the ion is sufficient to eject atoms or molecules from the material's surface. This can be beneficial in materials science where layer removal or patterning is necessary. Ion implantation, on the other hand, involves embedding ions into a substrate, which is essential for altering material properties or creating semiconductor devices.
The extent of these interactions is influenced by several factors, including ion energy, atomic mass, and the material characteristics. Understanding these interactions can help optimize processes in semiconductor fabrication or nanotechnology applications. Accurate knowledge about how ions affect various materials is essential in a range of fields from developing more efficient electronic components to innovative materials creation.
Instrumentation and Technologies for FIB
Instrumentation and technologies for Focused Ion Beam (FIB) systems are crucial to understanding the technology's capabilities. High-quality instrumentation allows researchers to manipulate materials at a microscopic level. This, in turn, opens new pathways for innovation in various fields such as materials science, microelectronics, and nanotechnology.
Components of FIB Systems
A FIB system consists of several key components that work together to achieve precision in material analysis and fabrication. These components include:
- Ion Source: The ion source generates the focused ion beam. Common sources are liquid metal ion sources, which provide excellent brightness and stability.
- Accelerating Column: This column accelerates the ions to high energies, essential for effective interaction with the sample material.
- Focusing Optics: These optics condense the ion beam down to a few nanometers, allowing for extremely fine milling and imaging capabilities.
- Sample Stage: The stage holds the sample in place. It is often equipped with motors that allow for precise movement, including tilting and rotation.
- Detection System: This component collects data on the ion-sample interactions. It can provide information on material composition, topography, and other properties.
These components are engineered to function in concert, delivering high-resolution data and enabling detailed analysis of materials.
Sample Preparation Techniques
Preparing samples for FIB analysis requires specific techniques to ensure quality results. Often, samples must be thin enough for ion penetration but also robust enough to withstand the ion beam's impact. Key techniques include:
- Cross-sectioning: This involves cutting a thin slice of the material to reveal internal structures.
- Slicing and Milling: The focused ion beam can mill away material in a controlled manner, allowing for precise shaping of samples.
- Coating: In some cases, especially for insulating materials, a thin conductive layer is applied to enhance imaging capabilities and improve charge dissipation.
Getting sample preparation right is vital, as it significantly affects the quality of FIB analysis. Proper techniques minimize sample damage and optimize data obtained from the interaction of the ion beam with the sample.
Hybrid Systems involving FIB and SEM
Hybrid systems that combine FIB with Scanning Electron Microscopy (SEM) offer unique advantages. These systems leverage the strengths of both technologies, creating a powerful tool for researchers. The benefits include:
- Complementary Imaging: SEM provides detailed topographical images while FIB allows for precise modifications. Together, they provide a comprehensive view of the sample's surface and structure.
- Simultaneous Processing: Researchers can mill and image samples sequentially, which saves time and allows for immediate verification of the sample's condition.
- Enhanced Analysis: The combination facilitates advanced imaging techniques and analysis, including 3D reconstructions of samples due to the multilayering potential of FIB.
Integrating FIB and SEM into hybrid systems enhances the capability of researchers, allowing for a deeper understanding of materials at the nanoscale. This fusion of technologies will continue to evolve, providing means for innovative studies in various scientific fields.
Key Insight: The evolution of instrumentation and technologies will dictate the future of FIB applications, enhancing capabilities and broadening its use in research and industry.
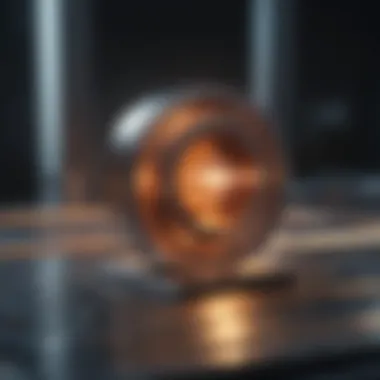
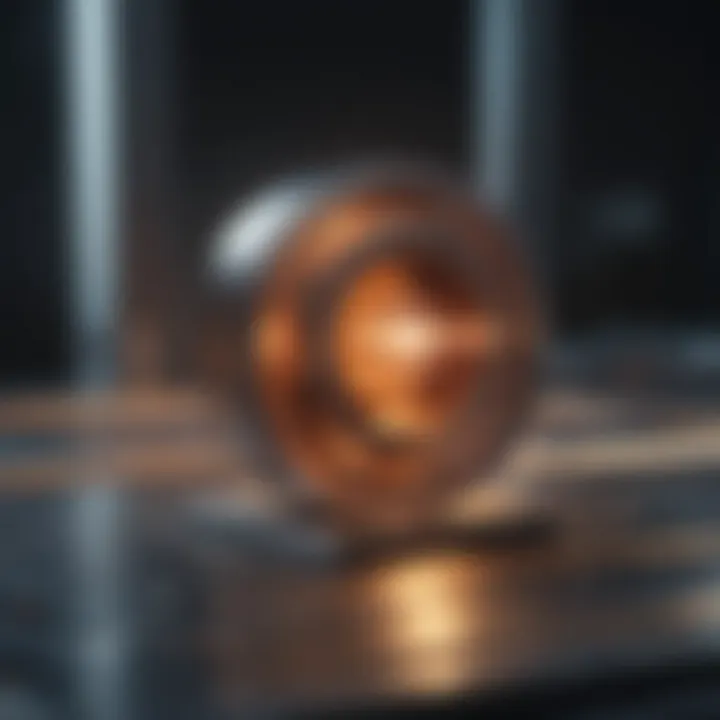
Applications of Focused Ion Beam Technology
The applications of focused ion beam (FIB) technology are integral to multiple fields of science and industry. By providing a high level of precision and customization in processing materials, FIB has become a vital tool in material analysis, fabrication, and modification. The importance of these applications lies in their impact on research capabilities, product development, and innovation in technology. The versatility of FIB systems allows researchers to conduct advanced investigations and meet specific requirements across various disciplines.
Materials Science
In materials science, focused ion beam technology is pivotal for studying and enhancing the characteristics of various substances. FIB allows for advanced imaging techniques, where high-resolution surface morphological studies can be performed. Researchers can create cross-sections of materials, facilitating the observation of internal structures and defects which are critical for understanding material properties.
The controlled removal of material with ion beams aids in the fabrication of samples for microscopy, ensuring that they meet specific requirements for detailed analysis. Moreover, the ability to do nanomachining opens up avenues for the exploration of new materials, including nanostructured materials that have unique properties that differ significantly from their bulk counterparts.
Nanotechnology
Focused ion beams play a crucial role in the field of nanotechnology. Their precision enables the fabrication of nanoscale devices and structures essential for advancements in various applications. By utilizing FIB, scientists can define features at dimensions below 100 nanometers. This capability is vital for producing components that enhance device functionality and performance.
Moreover, FIB systems can integrate with other techniques, like scanning electron microscopy (SEM), to create hybrid systems that offer more in-depth analysis and visualization of nanostructures. The capability to modify surfaces at the nanoscale allows for innovations in drug delivery systems, sensor technologies, and micro-electromechanical systems (MEMS).
Semiconductor Fabrication
In semiconductor fabrication, focused ion beam technology has revolutionized the way devices are designed and manufactured. FIB allows for precise etching and patterning of semiconductor layers, which is essential for device miniaturization. This technique leads to enhanced performance and efficiency of circuits.
Additionally, FIB can be utilized in the failure analysis of semiconductor devices. By examining the structural integrity of integrated circuits and detecting defects, engineers can improve manufacturing processes and ensure higher yield rates. The flexibility of FIB systems makes them suitable for both research and production environments, optimizing the process from design to final product.
Microelectronics
The microelectronics industry benefits significantly from focused ion beam technology, particularly in the realm of circuit design and modification. FIB can be used to diagnose issues within existing circuits. The capability to mill and modify components at the micro level allows engineers to repair or enhance routed pathways. This feature is essential for maintaining the longevity and reliability of electronic devices.
Furthermore, FIB technology facilitates the development of novel microelectronic architectures, such as 3D integrated circuits. This innovation contributes to the continuous push for more efficient and compact electronic systems, accommodating increasing demands for performance in consumer electronics.
FIB technology is no longer an auxiliary tool; it is at the forefront of technological innovation in materials science, semiconductor fabrication, and microelectronics.
In summary, focused ion beam technology harnesses the power of ions for diverse applications, promoting advancements in materials science, nanotechnology, semiconductor fabrication, and microelectronics. Each application reinforces the utility of FIB systems, contributing to significant technological progress across these fields.
Advantages and Limitations of FIB
The study of Focused Ion Beam (FIB) technology reveals several advantages and limitations that play a significant role in its utilization across various scientific and industrial applications. Understanding these factors is crucial for selecting the appropriate methods in different contexts, especially for students, researchers, and professionals.
Precision and Control
One of the primary advantages of FIB technology is its exceptional precision and control. FIB systems allow for the manipulation of materials at the nanoscale with remarkable accuracy. This capability is vital in fields such as materials science and semiconductor fabrication, where specific alterations at the atomic level can significantly affect a material's overall properties. For instance, in the manufacturing of microelectronics, the ability to selectively etch or deposit materials enables the creation of highly intricate structures required for advanced circuitry.
FIB can also perform high-resolution imaging, giving researchers insights into the nano-architectures of materials. The precise beam can selectively machine surfaces and prepare samples for further analysis, such as Transmission Electron Microscopy (TEM).
In practice, these systems can focus ion beams down to a few nanometers while maintaining a high current density. This specificity gives scientists and engineers the ability to target defects, optimize material characteristics, and innovate solutions tailored to specific tasks.
Sample Damage Concerns
Despite the advantages, the use of FIB does come with notable limitations, particularly concerning sample damage. The interaction of high-energy ions with target materials can induce significant structural changes. These changes may include displacement damage, amorphization, or even unwanted contamination. Such damage becomes a major concern, particularly in sensitive materials such as biological specimens or certain semiconductor materials.
Moreover, the high-energy state of incident ions can lead to sputtering, where atoms are ejected from the surface of a material. This can interfere with the integrity of the sample, thus complicating results in subsequent analyses. Therefore, researchers must carefully calibrate ion doses. This calibration ensures they balance the need for precise manipulation with the risk of compromising sample integrity.
"Understanding the balance between precision and damage in FIB technology is key for effective application."
Future Directions in FIB Technology
Focused Ion Beam technology continues to grow and evolve. The future directions of this technology are vital not only in advancing scientific knowledge but also in enhancing industrial applications. As research and development progresses, several specific elements emerge as critical both in terms of benefits and considerations for future applications of FIB technology.
Innovative advancements in ion source technology stand out as a primary area of focus. Enhancing the performance and versatility of ion sources allows for better resolution and improved throughput. As different materials and ions can be utilized, the adaptability of FIB systems increases significantly. This versatility can lead to more accurate characterizations and fabrications, which are crucial in fields such as nanotechnology and semiconductor manufacturing.
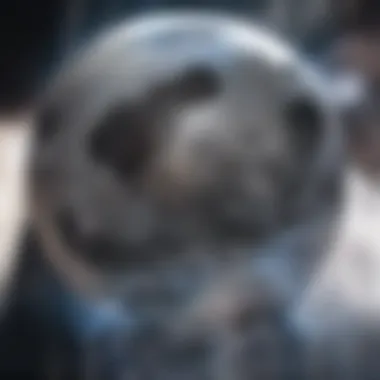
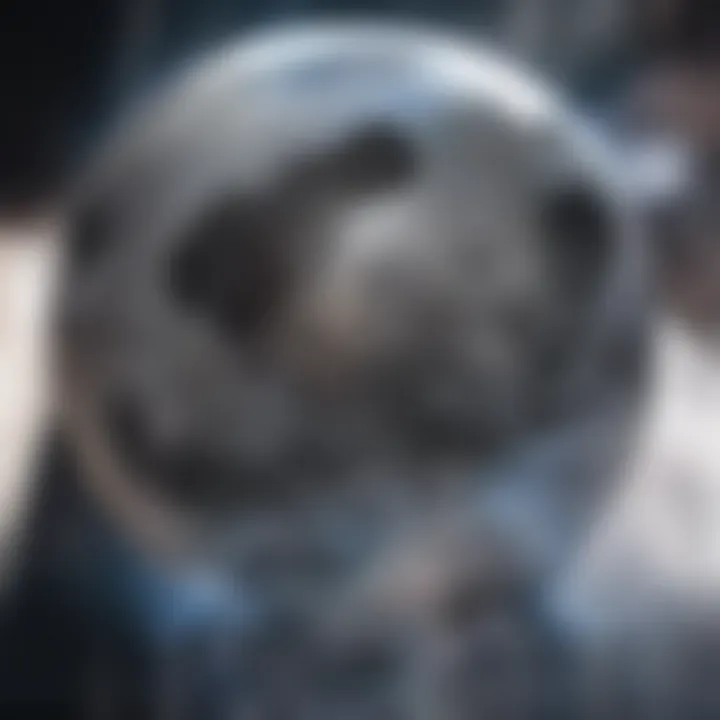
Integration with other analytical techniques represents another important avenue. By combining FIB with methods like scanning electron microscopy (SEM) and X-ray fluorescence, comprehensive data acquisition is achieved. This synergy allows researchers to gain deeper insights and higher resolution imaging. Such integrative approaches can provide a more holistic understanding of materials and their properties, making it easier to develop novel solutions to complex problems.
Lastly, emerging applications of FIB are redefining the potential of this technology. Industries such as biotechnology and quantum computing are beginning to explore how focused ion beams can facilitate new discoveries. Various material processing techniques, including precise material removal and nanoscale fabrication, expand the landscape of what can be achieved with FIB systems. These applications highlight not just the adaptability of FIB technology but also its potential to influence several scientific fields.
"The advancements in focused ion beam technology open new pathways for exploration, pushing the boundaries of what is possible in scientific research and industrial applications."
In summary, the future directions in FIB technology present remarkable opportunities for innovation. Researchers and practitioners must remain attuned to these developments to leverage their benefits effectively and address emerging challenges.
Advancements in Ion Source Technology
One of the key focus areas in the evolution of those systems is the development of more sophisticated ion sources. Improvements in ion source technologies, such as gas field ion sources (GFIS) and liquid metal ion sources (LMIS), have a significant impact on the performance of FIB systems.
- Gas field ion sources can produce highly focused ion beams with superior resolution.
- Liquid metal ion sources offer excellent stability and flexibility when different ions are required for diverse applications.
It is crucial that future research also focuses on sustainability and efficiency in ion source technology. This includes efforts to minimize waste and improve the lifespan of ion sources.
Integration with Other Analytical Techniques
As FIB technology progresses, combining it with other analytical methodologies enhances its capabilities. Integration with scanning tunneling microscopy (STM) or energy-dispersive X-ray spectroscopy (EDX) allows for detailed material analysis at the nanoscale.
Benefits of such integrations include:
- Enhanced imaging at atomic resolution.
- Comprehensive elemental analysis alongside morphological examination.
- Real-time feedback during material processing.
This interplay of technologies leads to the development of multifaceted tools that can adapt to various research needs.
Emerging Applications
The versatility of focused ion beams is particularly evident in emerging fields. Applications of FIB technology have expanded from semiconductor fabrication to include realms like biotechnology and photonics.
- In biotechnology, FIB is utilized to develop more efficient drug delivery systems.
- Photonics researchers apply FIB for fabricating devices that manipulate light at the nanoscale.
These applications may inspire further research directions and stimulate innovation across multiple disciplines. The push towards nanoscale fabrication techniques is becoming increasingly relevant, driving the demand for sophisticated FIB systems.
End
In the realm of science and industry, the role of Focused Ion Beam (FIB) technology proves to be paramount. This conclusion serves to reinforce the critical insights drawn throughout the article, highlighting the significance of FIB in various practical applications and advanced research fields.
Summary of Key Points
The discussion reveals several vital elements:
- Principles of FIB Technology: Review of ion beam physics and the intricate mechanisms of acceleration and focusing, emphasizing how these underpin the operational efficacy of FIB systems.
- Instrumental Architecture: Examination of the components that make up FIB systems, including the ion sources and the necessary sample preparation techniques, which are essential for effective operation.
- Varied Applications: FIB's application spans across numerous fields such as materials science, microelectronics, and nanotechnology, showcasing its versatility.
- Advancements and Shenarios: The article highlights emerging advancements and the integration of FIB with other technologies that indicate a promising future for its applications.
These points illustrate the multifaceted impact of FIB technology, culminating in its ability to advance scientific inquiry and industrial processes.
Significance of FIB in Scientific Research
The importance of FIB cannot be overstated.\nIts precision and control allow researchers to manipulate materials at the atomic level, enabling groundbreaking discoveries and innovations. This capability enhances our understanding of material properties and behaviors, ultimately leading to the development of new techniques and products across various industries.
One significant realm influenced by FIB technology is semiconductor fabrication, where it facilitates the production of smaller and more efficient electronic components. The importance extends into nanotechnology, where FIB is pivotal in creating nanostructures that drive innovation in fields such as medicine and materials science.
Moreover, advancing FIB technology aligns with the broader goal of integrating analytical techniques, thus providing comprehensive insights into materials and structures that were previously unattainable. The continual enhancement of FIB capabilities is expected to play a crucial role in future scientific research, ultimately shaping the landscape of technology and innovation.
In essence, Focused Ion Beam technology stands as a cornerstone in modern scientific research, promising advancements that will not only expand knowledge but also revolutionize industrial applications.
Ultimately, the future of research with the use of FIB technology holds boundless potential, making it a subject worthy of in-depth examination and exploration.
Key Considerations when Reviewing References
- Ensure that references are current, especially in a rapidly evolving field like FIB technology.
- Look for comprehensive review articles that summarize recent advancements and provide extensive bibliographies for further reading.
- Understand the influence of various studies on current applications and future directions in research.
In summary, the references section encapsulates the scholarly foundation upon which the article is constructed. It not only validates the discussed content but also enriches the reader's experience by serving as a springboard for further investigation into the diverse applications and principles of focused ion beams.