Antisense Oligonucleotide Design: Strategies and Applications
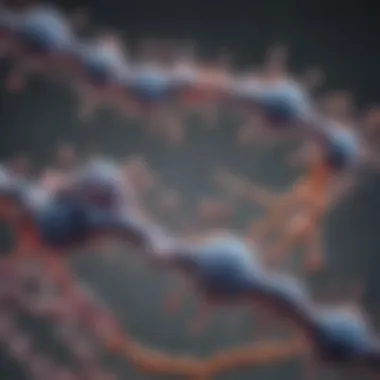
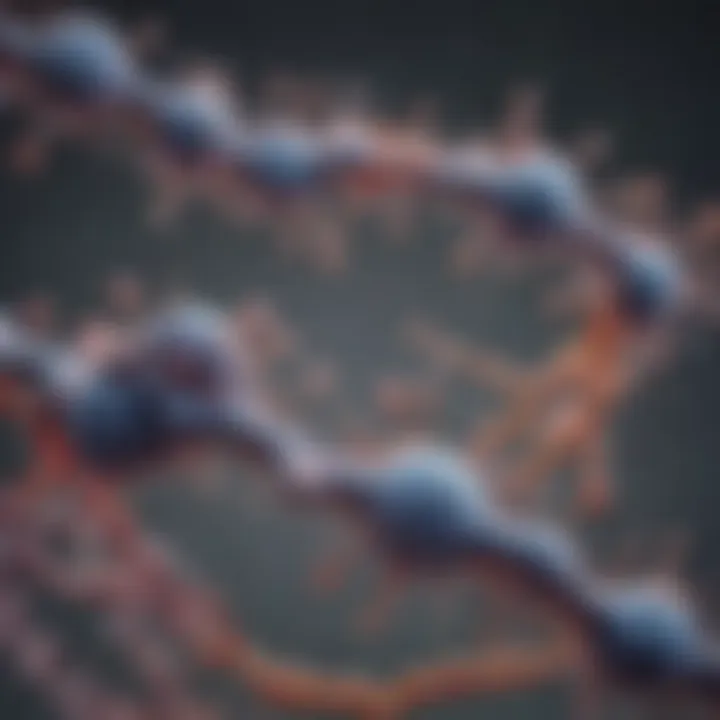
Intro
Antisense oligonucleotides (ASOs) represent a frontier in genetic research and therapeutic innovation. By strategically targeting messenger RNA (mRNA), these short, synthetic strands can effectively influence gene expression. This article provides a thorough exploration into the design, methodologies, and applications of ASOs, addressing both theoretical and practical insights.
The nuances of antisense oligonucleotide design are crucial for their functionality. Through this exploration, we aim to elucidate the various strategies that underpin successful ASO development, unpacking essential considerations such as sequence parameters and chemical modifications. Furthermore, the role of bioinformatics in enhancing ASO design will be a focal point, shedding light on how computational tools can optimize outcomes in gene modulation.
As interest in gene regulation continues to accelerate across research and therapeutic landscapes, understanding the principles behind ASO design will empower students, researchers, educators, and professionals. This article will systematically navigate through these topics, ultimately synthesizing a comprehensive view of antisense oligonucleotide usage in modern science.
Research Overview
Summary of Key Findings
Antisense oligonucleotides serve as critical tools for silencing gene expression and regulating cellular functions in various contexts. Key findings in recent studies highlight their therapeutic potential in combating genetic disorders, cancers, and infectious diseases. The efficacy of ASOs largely depends on their ability to bind specifically to the target mRNA, a process influenced by their design, chemistry, and the biological environment.
Methodologies Employed
In crafting ASOs, researchers generally utilize a variety of methodologies. Key strategies include:
- Sequence Design: It involves determining optimal target regions based on mRNA structure.
- Chemical Modification: Enhancing stability and decreasing off-target effects through nucleotide alterations.
- Bioinformatics Tools: Employing computational methods to predict binding affinity and specificity.
These methodologies are instrumental in improving the therapeutic index of ASOs, making them increasingly relevant in molecular biology.
In-Depth Analysis
Detailed Examination of Results
A critical examination of current literature reveals significant advances in ASO design techniques. For instance, recent studies demonstrate that specific chemical modifications, such as phosphorothioate linkages, can significantly improve an ASO's stability and potency. Furthermore, the incorporation of locked nucleic acids (LNAs) has been shown to enhance binding affinity, thus increasing the therapeutic potential of ASOs in various applications.
"The substantial progress in modifying oligomeric properties opens new avenues for targeted gene regulation and therapy."
Comparison with Previous Studies
When comparing findings from the current research with previous studies, it becomes evident that there is a trend towards increasing specificity in ASO design. Earlier approaches often faced challenges with off-target effects, which hindered therapeutic use. Innovations in computational modeling and experimental validation have drastically improved design accuracy, as seen in several recent case studies.
The advancements reflect a synthesis of academic inquiry and technological progress, indicating a promising future for antisense oligonucleotide therapies. As the pursuit of gene modulation continues, the understanding of design strategies around ASOs will remain critical for students, researchers, and professionals aiming to delve deeper into genetic manipulation.
Prelims to Antisense Oligonucleotides
Antisense oligonucleotides (ASOs) represent a significant advancement in molecular biology and therapeutic development. Their role in gene modulation has reshaped how researchers and clinicians approach genetic diseases. ASOs are short strands of nucleotides designed to bind to specific mRNA molecules. This binding can inhibit the translation of the target gene into protein, thereby affecting gene expression. Understanding ASOs is crucial for anyone in the field of genetics, especially those focused on developing treatment strategies for genetic disorders.
The importance of antisense oligonucleotides stems from their ability to specifically target and regulate gene expression. This specificity allows for fine-tuning therapeutic approaches while minimizing off-target effectsβa crucial element in effective treatments. ASOs can be tailored to various diseases by simply altering their sequence, which offers a versatile platform for therapeutic developments. Furthermore, their application extends beyond simply silencing genes; they can also be used to correct splicing defects or modify RNA stability.
The following subsections will provide a detailed overview of the definition and mechanism of action of antisense oligonucleotides, alongside their historical development. By grasping these foundational concepts, readers can better appreciate the complexities and potential of ASOs in contemporary biological research and therapeutic applications.
Importance of Antisense Oligonucleotide Design
The design of antisense oligonucleotides (ASOs) plays a crucial role in modern biotechnology and molecular medicine. Understanding the principles behind ASO design provides invaluable insight into how these molecules can be optimized for specific applications. The significance of this area lies in its ability to deliver targeted therapies that can modulate gene expression with remarkable precision.
Role in Modern Molecular Biology
In contemporary molecular biology, ASOs are recognized as versatile tools for gene regulation. They offer the ability to silence genes or modify splicing events at the mRNA level. This specificity leads to fewer off-target effects compared to traditional approaches. Furthermore, the ease of synthesis and modification allows for rapid adaptation to different research and therapeutic needs.
Several factors contribute to the essentiality of ASOs in research:
- Targeted gene silencing: ASOs can be designed to bind selectively to mRNA sequences, preventing translation and effectively silencing gene expression.
- Customization: Researchers can tailor the oligonucleotide sequences to address specific genetic targets, increasing the efficiency of experiments.
- Versatility: ASOs are applicable in various disciplines ranging from basic research to therapeutic interventions for diseases such as cancer, genetic disorders, and viral infections.
ASOs also provide a platform for exploring fundamental biological questions. For instance, by silencing specific genes, researchers can better understand the role of those genes in cellular processes. This capability enhances our overall comprehension of molecular mechanisms and pathways.
Therapeutic Potential
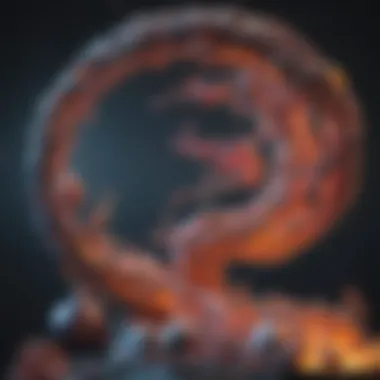
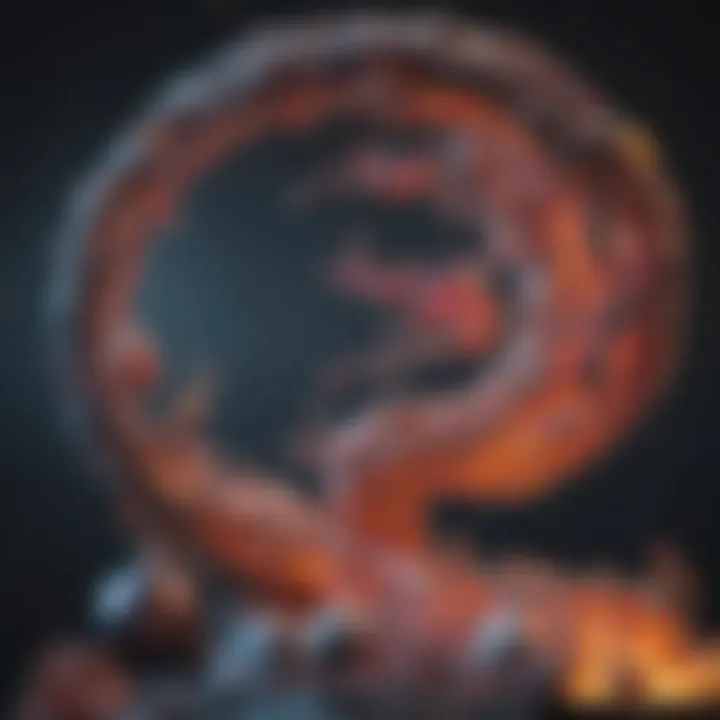
The therapeutic potential of antisense oligonucleotides is one of the defining characteristics that elevate their importance. ASOs have transitioned from experimental tools to viable treatment options for several medical conditions. The ability of ASOs to precisely modulate gene expression offers promise for treating diseases rooted in genetic abnormalities.
Key aspects of ASO therapeutic applications include:
- Diverse Disease Targets: ASOs have shown efficacy in targeting both genetic disorders and complex diseases like cancer and neurodegenerative conditions. This reveal their broad applicability in pharmacological applications.
- Reduced Side Effects: Unlike some traditional therapies, ASOs can reduce undesired interactions with unintended targets, enhancing patient safety profiles.
- Regulatory Advances: Recent approvals for ASO-based drugs underscore their viability as therapeutics. For instance, drugs like Eteplirsen and Nusinersen have made significant impacts on patient care, reshaping treatment paradigms in specific diseases.
"Antisense oligonucleotides are paving the way for innovative therapeutic solutions that embrace the future of personalized medicine."
Key Considerations in ASO Design
In the arena of antisense oligonucleotide (ASO) design, there are several pivotal factors that influence the effectiveness of these molecules. Understanding these considerations is vital for optimizing their application in gene modulation and therapeutic development. The design process demands a keen focus on scientific principles while accommodating the biological context in which these oligonucleotides function. Key considerations include target validation and selection, length and sequence characteristics, and secondary structure considerations. Each of these elements contributes significantly to the stability, specificity, and overall efficacy of ASOs.
Target Validation and Selection
The first step in the ASO design process involves a meticulous approach to target validation and selection. This entails identifying gene targets that are not only relevant to the disease in question but also amenable to modification through antisense strategies. Effective validation includes conducting thorough literature reviews and leveraging existing genomic databases to assess the gene's role in disease processes.
Choosing the right target is critical. Factors such as expression levels, functional relevance, and the presence of potential off-target effects must be considered. Ideally, the target gene should have a well-understood function and its inhibition should lead to a desirable therapeutic outcome. Successful case studies often highlight the impact of focused target selection in achieving impactful results.
Length and Sequence Characteristics
The length and sequence of an ASO are determining factors for its functional efficacy. Typically, ASOs range from 15 to 25 nucleotides in length. Longer ASOs may offer higher specificity but can also lead to increased off-target effects. Conversely, shorter sequences might lack adequate binding affinity to the target mRNA, undermining their therapeutic potential.
Additionally, the sequence composition plays a critical role in determining the binding stability of the ASO to its target RNA. Guanine-cytosine (GC) content, presence of homopolymers, and the arrangement of bases can affect melting temperatures and binding kinetics. Ensuring an optimal balance between these factors is essential for maximizing ASO performance. Most successful ASOs exhibit a sequence that is complementary to the target mRNA, thereby promoting effective hybridization.
Secondary Structure Considerations
The secondary structure of the target mRNA is another critical consideration in ASO design. The formation of secondary structures can hinder the binding of the ASO to its intended target, thus impacting its efficacy. The presence of hairpins, loops, or other folding patterns can obstruct access to the mRNA and prevent effective modulation of gene expression.
Designers must thus examine the potential secondary structure of the target mRNA prior to ASO development. This can be accomplished using predictive algorithms that model mRNA folding. By anticipating and mitigating these structural challenges, ASOs can be designed to bypass potential obstructions, thereby ensuring better access to the target sites. Additionally, knowledge of the local RNA environment can help inform the selection of ASOs that best fit the dynamic characteristics of their targets.
In summary, the key considerations in ASO design are integral to ensuring that these oligonucleotides are effective for their intended purpose. A careful approach to target selection, attention to sequence characteristics, and an understanding of secondary structure dynamics all contribute to a more successful application of ASOs in research and therapeutic interventions.
Chemical Modifications for Enhance Efficacy
Chemical modifications serve as a crucial aspect in the design and application of antisense oligonucleotides (ASOs). These modifications are instrumental in improving the stability, binding affinity, and overall efficacy of the oligonucleotides. The natural chemical structure of nucleic acids is often susceptible to degradation in biological environments. Therefore, tweaking the nucleotide structure through various means can yield significant benefits.
Incorporating such modifications can lead to enhanced resistance to nucleases, improved hybridization to target RNA, and reduced off-target interactions. These features are particularly important for developing ASOs used in therapeutic contexts, where maintaining specificity and minimizing unintended effects is vital.
Key considerations in selecting chemical modifications include the type of target mRNA and the desired biological outcome. The balance between stability, potency, and safety must also be carefully managed. This section will delve into three significant types of modifications: Phosphorothioate modifications, Locked Nucleic Acids (LNAs), and Peptide Nucleic Acids (PNAs).
Phosphorothioate Modifications
Phosphorothioate modifications are among the most widely utilized alterations in antisense oligonucleotide design. This modification typically involves substituting one of the non-bridging oxygen atoms in the phosphate backbone with a sulfur atom. The result is increased resistance against enzymatic degradation.
The key advantages of phosphorothioate ASOs include:
- Enhanced Stability: The sulfur substitution protects the ASOs from degradation by exonucleases.
- Improved Affinity: They can exhibit higher binding affinity towards complementary RNA sequences than their unmodified counterparts.
- Biological Efficacy: The modifications can lead to greater effectiveness in gene regulation through improved target engagement.
However, the presence of phosphorothioate modifications comes with considerations for off-target effects. Researchers must balance these properties to achieve maximum therapeutic benefits.
Locked Nucleic Acids (LNAs)
Locked Nucleic Acids (LNAs) represent another promising type of modification in the landscape of ASO design. LNAs incorporate a unique sugar moiety that locks the ribose in a specific conformation, resulting in a more stable and rigid structure. This rigidity leads to improved binding properties.
The benefits of using LNAs include:
- Increased Binding Affinity: LNAs can bind to RNA targets with high specificity, often resulting in tighter hybridization.
- Resistance to Degradation: LNAs are more resistant to nucleases compared to traditional DNA and RNA sequences, which prolongs their action in biological environments.
- Therapeutic Versatility: LNAs have been utilized effectively in various therapeutic applications, showcasing their broad potential.
Despite these advantages, the higher cost of synthesizing LNA-modified ASOs can be a limiting factor in their widespread use.
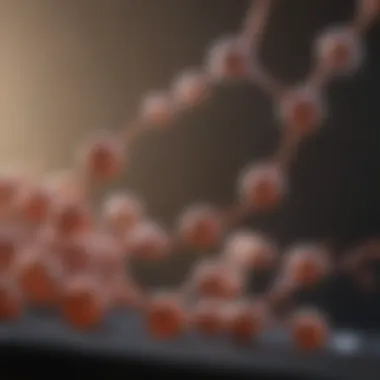
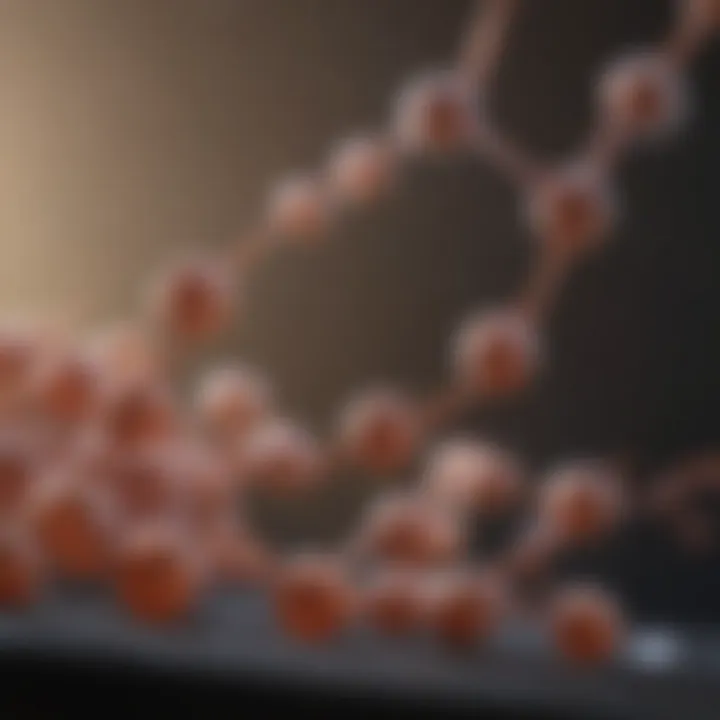
Peptide Nucleic Acids (PNAs)
Peptide Nucleic Acids (PNAs) offer a distinct approach to ASO design by replacing the sugar-phosphate backbone with a peptide-like structure. This unique configuration results in properties that can surpass traditional nucleic acids in specific applications.
Advantages of PNAs include:
- High Stability: PNAs are exceptionally stable, both in terms of thermal stability and resistance to enzymatic degradation.
- Target Specificity: PNAs exhibit strong binding to complementary RNA and DNA, which is advantageous for applications requiring high specificity.
- Reduced Off-Target Effects: Their selective binding characteristics may help minimize interactions with non-target sequences, reducing potential side effects.
Nevertheless, the challenges of cellular uptake and delivery of PNAs remain as obstacles that scientists are actively working to overcome.
These chemical modifications are not just enhancements; they are essential to the success of antisense oligonucleotide technology in therapeutic contexts.
In summary, understanding the role of chemical modifications is vital for optimizing antisense oligonucleotide design. Modifications such as phosphorothioate, Locked Nucleic Acids, and Peptide Nucleic Acids help improve efficiency and therapeutic potential, paving the way for novel treatment strategies.
Bioinformatics in ASO Design
Bioinformatics plays a crucial role in the design of antisense oligonucleotides (ASOs). It integrates computational tools and methods to analyze biological data, facilitating precision in ASO development. As antisense oligonucleotides target specific mRNA sequences to modulate gene expression, identifying optimal targets is essential for effective design. Bioinformatics allows researchers to predict target interactions, assess sequence stability, and anticipate potential off-target effects. This enhances the efficiency of the design process, ultimately leading to better therapeutic outcomes.
The importance of bioinformatics in ASO design can be summarized as follows:
- Target identification: Enables the selection of mRNA sequences relevant to the disease.
- Sequence optimization: Helps in designing the most effective ASOs with minimal off-target activity.
- Data integration: Combines various biological datasets to inform the design process.
- Predictive modeling: Facilitates the estimation of ASO efficacy and safety.
Utilizing bioinformatics in ASO design aligns with the goal of advancing molecular medicine. It implicates a shift toward more rational and data-driven approaches rather than trial-and-error methods which can be time-consuming and costly.
Computational Tools for Sequence Analysis
Computational tools are essential for sequence analysis in the design of ASOs. These tools assist in identifying potential target sites within mRNA sequences. Various software packages and online databases provide critical functionalities. Some of them include:
- BLAST (Basic Local Alignment Search Tool): Allows users to compare nucleotide sequences against a database, identifying matches that may serve as ASO targets.
- RNAstructure: A software tool that predicts RNA secondary structures, providing insights into possible binding sites for ASOs.
- mfold: This tool predicts the structure of RNA sequences, crucial for understanding how designed ASOs may interact with their targets.
These tools streamline the design process and enhance the likelihood of selecting high-affinity targets. The results obtained from such analyses are significant in evaluating sequence characteristics that impact ASO performance.
Predictive Models for Target Affinity
Predictive models for target affinity are vital in determining the efficacy of ASOs. These models utilize algorithms to estimate the binding strength between an ASO and its target mRNA. Factors that influence binding affinity include:
- Sequence complementarity: The degree of match between the ASO and mRNA sequence affects the binding.
- Concentration: The relative concentrations of the ASO and the target can alter binding dynamics.
- Chemical modifications: Modifications influence binding stability and overall effectiveness.
By leveraging predictive modeling in the design phase, researchers can prioritize sequences that exhibit strong binding characteristics. Moreover, these models help in mitigating off-target risks to some extent.
"The integration of bioinformatics and predictive modeling represents a paradigm shift in ASO design, enabling more informed decisions and accelerating therapeutic development."
Applications of Antisense Oligonucleotides
Antisense oligonucleotides (ASOs) have significant applications in the fields of molecular biology and medicine. Their ability to specifically bind to target mRNA enables the modulation of gene expression, making them valuable tools. This section covers the diverse applications of ASOs, particularly focusing on gene knockdown strategies, therapeutic usages in diseases, and notable case studies from clinical trials.
Gene Knockdown Strategies
Gene knockdown strategies are essential for studying gene function and therapeutic interventions. ASOs provide a way to selectively inhibit the expression of specific genes. By binding to complementary mRNA sequences, ASOs can effectively degrade the target RNA through the action of RNase H or prevent translation without degradation. This feature allows researchers to:
- Investigate gene function by observing phenotypic changes following knockdown.
- Create models for diseases related to specific gene mutations.
- Determine the potential of genes as therapeutic targets.
The efficacy of gene knockdown using ASOs depends on several factors. These include the sequence design, modifications made to the oligonucleotides, and the delivery method used. Researchers often optimize these elements to achieve the desired level of gene suppression in specific cellular contexts. Careful consideration must be taken to ensure that the ASOs do not induce off-target effects, which can complicate results.
Therapeutic Applications in Diseases
The therapeutic potential of antisense oligonucleotides extends across various diseases, especially genetic disorders and cancers. By targeting the mRNA of disease-causing genes, ASOs can reduce or eliminate harmful protein products. Applications include:
- Spinal Muscular Atrophy (SMA): Nusinersen has been successfully used to treat SMA by inducing the production of a functional SMN protein.
- Duchenne Muscular Dystrophy (DMD): ASOs are in development to skip specific exons, restoring functional dystrophin protein.
- Cancer therapies: By targeting oncogenes, ASOs can inhibit tumor growth and sensitize cancer cells to chemotherapy.
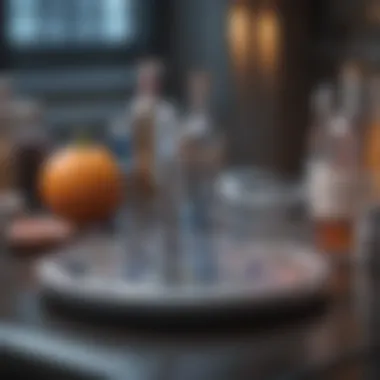
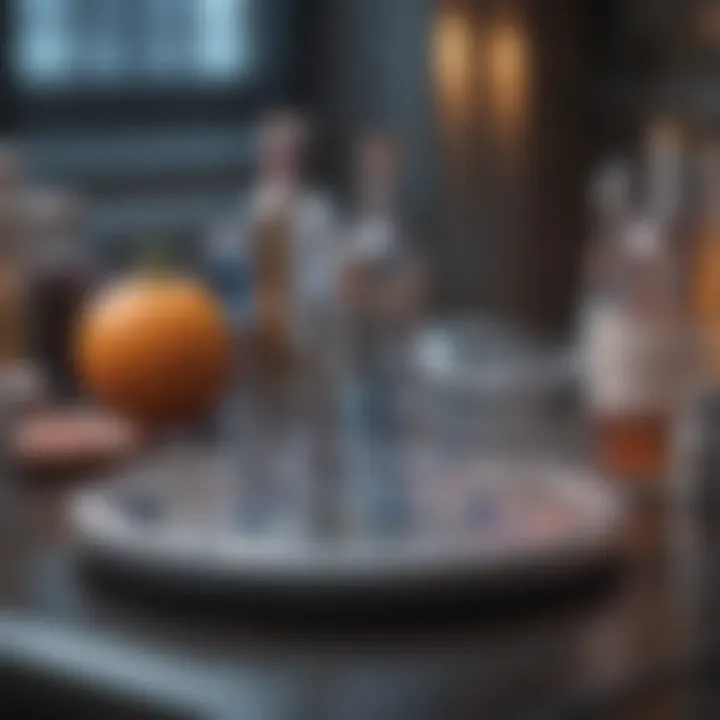
The versatility of ASOs allows for tailored approaches in treating diverse conditions. However, each application requires extensive research and trials to understand dosing, delivery, and long-term effects. This necessitates a strong foundation of preclinical models to assess safety and efficacy.
Case Studies of ASOs in Clinical Trials
Numerous clinical trials have been undertaken to assess the effectiveness of ASOs. Well-documented cases provide insights into their potential as therapeutic agents. Some notable examples include:
- Nusinersen (Spinraza): Approved in 2016 for the treatment of SMA, the drug has shown significant improvements in motor function amongst patients. Research demonstrated marked progress in infants with the most severe form of the disease, confirming the potential of ASOs in treating genetic conditions.
- Eteplirsen (Exondys 51): This ASO is designed for DMD patients with a specific mutation. Despite controversy over the approval process, studies showed that patients receiving the treatment experienced slower disease progression.
- IONIS-HTTRx: An ASO that targets the HTT gene in Huntington's disease patients. Preliminary trials have shown promise in lowering HTT levels, which may alleviate symptoms of the disease.
These case studies illustrate the varied applications of antisense oligonucleotides. They emphasize the continuous need for innovation and adaptation in ASO design to enhance their feasibility in clinical settings.
Overall, the applications of antisense oligonucleotides represent a progressive shift in modern therapeutic strategies. With advancements in technology and understanding of gene expression, ASOs are poised to play a critical role in personalized medicine and targeted therapies.
Challenges in Antisense Oligonucleotide Technology
The development of antisense oligonucleotide (ASO) technology presents numerous challenges that must be addressed for optimal efficacy and safety in therapeutic applications. These challenges will shape the direction and success of research in this field. Key considerations include delivery mechanisms and the potential for off-target effects. Both of these elements are critical in ensuring that ASOs achieve the desired therapeutic effect without introducing significant risk.
Delivery Mechanisms
Effective delivery mechanisms are essential for the successful application of antisense oligonucleotides. Given their nature as nucleic acid-based therapies, ASOs face significant hurdles when it comes to cellular uptake and distribution within the body.
- Transport Barriers: Biological membranes can be impermeable to large molecules like ASOs. This limits the ability of these oligonucleotides to reach their target cells. Enhancing delivery systems is a crucial area of ongoing research.
- Nanoparticle Carriers: One promising solution involves the use of nanoparticle carriers, which can encapsulate ASOs. These carriers help protect the oligonucleotides from degradation, allowing for more efficient delivery. Various types of nanoparticles, such as liposomes and dendrimers, are being investigated.
- Targeting Ligands: To improve specificity, researchers can attach ligands to ASOs that bind to specific receptors on target cells. This approach enhances the likelihood of ASOs being taken up by the intended cells and reduces exposure to non-target tissues.
Successful delivery of ASOs to target cells continues to be a pertinent research focus. Improved methods can greatly enhance the therapeutic utility of ASOs in clinical applications.
Off-Target Effects and Safety Concerns
While antisense oligonucleotides have great therapeutic potential, the risk of off-target effects poses significant safety concerns. Off-target effects can lead to unintended interactions that may compromise efficacy or cause adverse reactions.
- Binding Affinity: ASOs may bind to unintended mRNA targets due to sequence similarity. This unintentional binding can disrupt normal gene expression, potentially leading to harmful consequences.
- Evaluation Tools: Several methodologies are employed to evaluate the specificity of ASOs. High-throughput sequencing technologies can identify off-target interactions, helping researchers refine designs to minimize these effects.
- Safety Profiles: Rigorous testing of safety profiles is vital before ASOs enter clinical trials. Preclinical studies must assess both intended and unintended effects to ensure the safety of ASO therapies.
Future Directions in ASO Research
The area of antisense oligonucleotide (ASO) research is evolving rapidly. This evolution shapes not just scientific inquiry but also therapeutic modalities. Future directions in ASO research highlight vital advancements and innovations that can yield significant benefits. Notably, exploring these directions can lead to enhanced efficacy, safety, and delivery mechanisms, thereby addressing some current challenges.
Novel ASO Platforms
Emerging ASO platforms provide various new strategies that can revolutionize this field. Innovations like RNA-targeting CRISPR technologies present opportunities for precise gene editing, opening new pathways to treat genetic conditions more effectively. Other platforms include next-generation RNA interference (RNAi) technologies, which capitalize on the natural cellular machinery to regulate gene expression.
For example, ASOs paired with nanoparticle delivery systems can improve cellular uptake and stability in biological systems. These developments can mitigate the limitations of traditional ASO approaches, amplifying therapeutic outcomes. Effective platforms not only target the desired mRNA but also enable potential multi-targeting strategies, creating a more holistic approach towards complex diseases.
Regulatory Perspectives
Regulation plays a crucial role in the advancement of ASO technologies. As ASOs move from laboratory research to therapeutic agents, understanding the regulatory landscape becomes key. Regulatory agencies like the FDA and EMA have set guidelines for evaluating the safety and efficacy of ASOs in clinical settings.
Future ASO research must take into account these regulatory frameworks. Researchers need to ensure compliance with guidelines for preclinical studies and clinical trial design. This may involve developing standardized assays to assess off-target effects and optimizing dosing regimens. Collaboration between scientists and regulatory bodies can facilitate a more seamless transition from preclinical development to therapeutic applications.
"Regulatory clarity can drive innovation, making a significant difference in the integration of ASOs into mainstream medicine."
Continued exploration in these areas promises to enhance treatment options and underscores the importance of ASO design in modern biomedical research.
End and Implications for the Field
The design of antisense oligonucleotides (ASOs) stands as a transformative approach in molecular biology and targeted therapies. This technology has potential that extends beyond traditional genetic interventions. Understanding the implications of ASO design is paramount for both research and clinical applications. The concluding section discusses major takeaways that can shape future research directions and therapeutic strategies, as well as the evolving landscape of genetic technology.
Summarizing Key Learnings
Throughout this article, various pivotal themes surrounding ASO design were emphasized. First, it is critical to recognize the complexity of target validation. Engaging in thorough validation processes ensures that the chosen target mRNA truly contributes to disease mechanisms. Moreover, the importance of sequence characteristics is highlighted. The precise design of the oligonucleotide sequence significantly affects its efficacy and specificity in gene silencing.
Modified nucleic acids also play a critical role. Techniques such as phosphorothioate modifications and the use of locked nucleic acids enhance ASO stability and function. As the tools in bioinformatics have advanced, researchers are now equipped to analyze vast genetic datasets, facilitating improved designs. These insights confirm that ASO technology is not merely a passing trend; it is an evolving discipline that demands ongoing attention and innovation.
Impact on Future Therapeutic Strategies
The potential applications of ASOs in therapeutic contexts are vast. As novel diseases are understood at a molecular level, ASOs can be tailored to target specific mutations and dysregulated genes. This adaptability signifies a shift in how diseases like cancer and genetic disorders are approached. The evolution of ASO design methodologies has opened avenues for personalized medicine, where treatments can be customized based on individual genetic profiles.
"Antisense oligonucleotides will play a crucial role in future therapeutic strategies, addressing unmet medical needs directly at the genetic level."
Future research should focus on improving delivery mechanisms to enhance tissue uptake and reduce off-target effects. Understanding these aspects will be paramount for the clinical success of ASOs. Additionally, regulatory frameworks need to evolve in tandem with advancements in ASO technology to ensure patient safety and ethical research practices.