Understanding Thin Film Filters: Principles and Applications
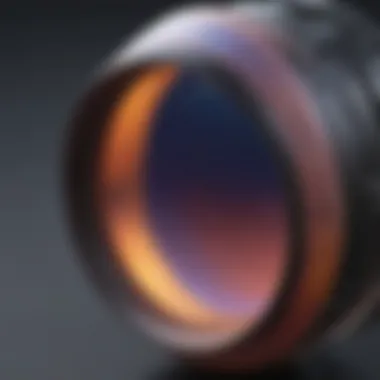
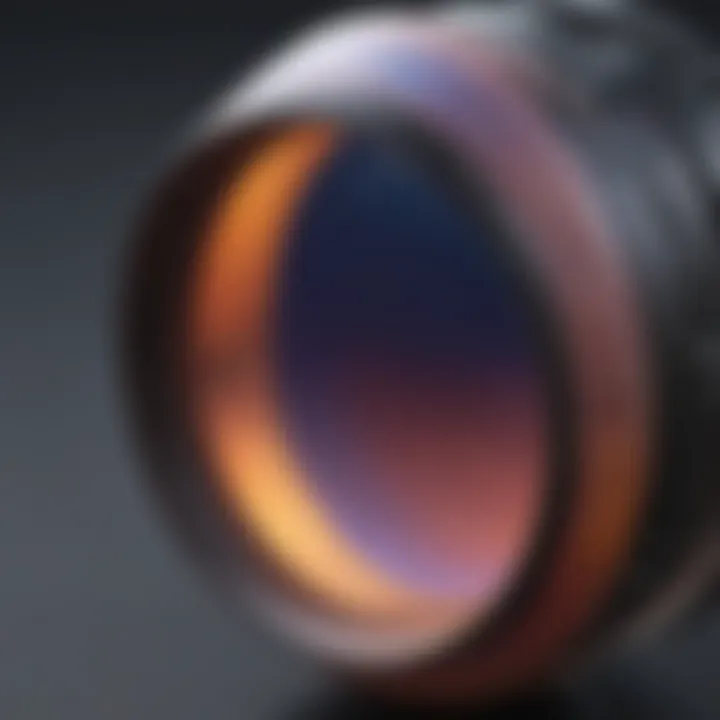
Intro
Thin film filters represent a significant advancement in optical technology, involving precise layers of materials that manipulate light according to specific needs. Their use spans various fieldsโfrom telecommunications to advanced imaging systemsโdemonstrating the filters' versatility and essential nature in modern applications.
This article aims to provide insight into the principles underlying these filters, the processes used in their manufacturing, and the impact they have across technological domains. By understanding thin film filters, one grasps not only the mechanics of light manipulation but also the broader implications for innovation in various industries.
Research Overview
Summary of Key Findings
Thin film filters are designed to selectively transmit or reflect different wavelengths of light based on their specific design and material properties. Key findings from recent studies show that the effectiveness of these filters depends heavily on their thickness, refractive index, and the number of layers involved. The advancements in material science have resulted in more efficient filters that boast improved durability and performance, suitable for high-demand applications.
Methodologies Employed
Research into thin film filters commonly utilizes a combination of theoretical modeling and empirical testing. Optical simulations play a pivotal role in predicting behavior, while experimental techniques such as spectrophotometry assess filter performance under actual working conditions. This dual approach helps verify theoretical predictions and enhances the design process.
In-Depth Analysis
Detailed Examination of Results
Recent data indicate that the design of thin film coatings can be finely tuned to optimize performance for applications like optical communication. Filters are engineered to exhibit specific characteristics, such as low loss and high passband, making them invaluable in minimizing signal degradation in fiber optic systems.
Comparison with Previous Studies
When comparing recent studies to earlier research, a distinct trend emerges. New fabrication techniques, such as atomic layer deposition, allow for greater control over layer uniformity, leading to enhanced transmission rates and reliability. As a result, contemporary filters outperform their predecessors in both efficiency and applicability across various disciplines.
"Advancements in thin film filter technology are paving the way for innovations that can revolutionize fields like telecommunications and imaging systems."
Preamble to Thin Film Filters
Thin film filters are essential components in various optical systems, fulfilling critical roles in telecommunications, biomedical imaging, consumer electronics, and more. They selectively transmit or reflect specific wavelengths of light, enhancing the functionality and efficiency of technologies that depend on precise optical manipulation. Understanding thin film filters is vital not only for those in optical engineering but also for anyone involved in sectors relying on advanced imaging techniques.
Definition and Overview
Thin film filters are optical devices consisting of layers of thin materials, typically fewer than 500 nanometers thick. These layers are deposited on substrates, allowing for the manipulation of light by utilizing interference phenomena. The purpose of these filters is to control the light spectrum that passes through or reflects off a surface, enabling precise color filtering and enhancement in imaging and optical systems.
Thin film filters can be categorized based on their functionality, including bandpass filters that allow a specific range of wavelengths, notch filters that block certain wavelengths, and dichroic filters that reflect some wavelengths while transmitting others. Their design relies on the principles of optical interference, which empower them to perform with high efficiency and accuracy.
Historical Context
The development of thin film technology can be traced back to the early 20th century when scientists began experimenting with multiple layers of thin materials to create interference patterns. In the 1950s, advancements in material science enabled the practical application of these filters in optical devices. Pioneering work in this domain laid the groundwork for modern manufacturing techniques such as physical vapor deposition and chemical vapor deposition.
As technology progressed, the applications of thin film filters expanded dramatically. From their early uses in scientific instruments to contemporary implementations in consumer electronics, the evolution of these filters reflects the growing demand for enhanced optical performance. Today, thin film filters are not just limited to laboratories; they play a crucial role in everyday devices such as camera lenses and optical sensors.
"Thin film filters are not merely optical tools; they represent a confluence of physics, engineering, and technology that advances multiple industries."
With this understanding, we can now delve deeper into the operating principles of thin film filters and the specific technologies involved in their fabrication.
Operating Principles of Thin Film Filters
Thin film filters are crucial in various optical applications. Their operating principles are essential to understanding how these filters perform in different conditions. The significance of this topic lies not only in the mechanics of light manipulation but also in the resulting benefits observed in various technologies. By grounding oneself in the principles of thin film optics, one can appreciate the complexity and adaptability of these filters in modern applications.
Interference Phenomena
Interference phenomena are the core of the operation of thin film filters. This process involves the interaction of light waves reflected off different layers within the thin film. When light hits these layers, some light is transmitted, while some is reflected. The reflected waves can either reinforce or cancel each other out, depending on the phase relationship of the waves. This effect produces the characteristic wavelengths that the filters are designed to manipulate.
The design of a thin film filter typically involves the precise control of layer thicknesses and refractive indices to achieve a desired spectral response. For instance, constructive interference occurs when the paths of the reflected light waves align, enhancing certain wavelengths. Conversely, destructive interference diminishes specific wavelengths. This targeting of light behavior allows for a high degree of customization in filter performance, enabling their use across varied applications.
Transmission and Reflection
Transmission and reflection are two significant aspects of how thin film filters operate. Understanding these processes can lead to improvements in filter designs and applications. During transmission, a portion of the incoming light passes through the filter, while reflection involves light bouncing off the surface.
In an ideal setup, the amount of light transmitted can be maximized while minimizing reflection losses. The balance of these characteristics depends on the design parameters of the filter. These include materials used, the number of layers, and the angle of incidence of light. Each filter's specifications dictate its suitability for distinct tasks.
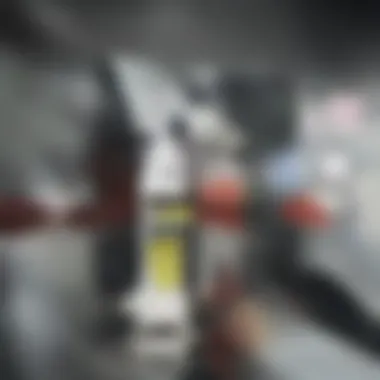
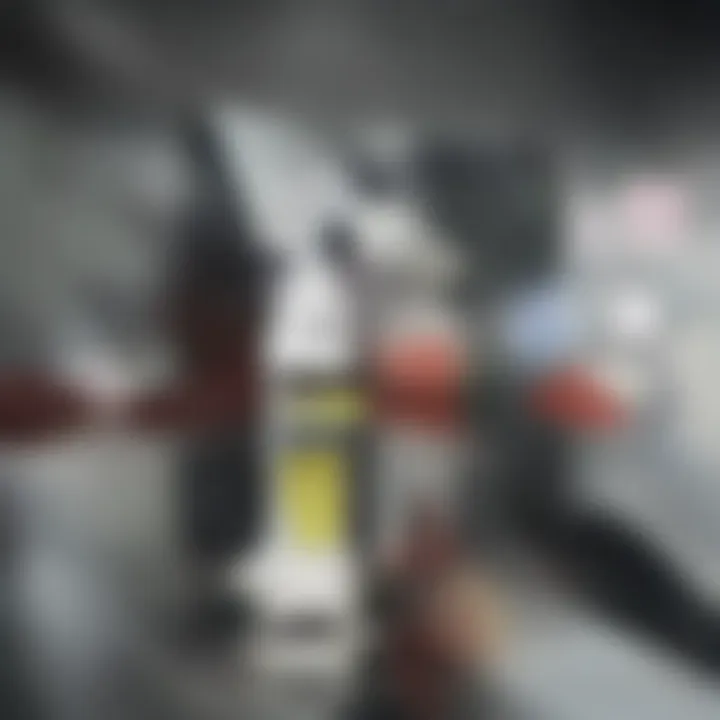
An important consideration in thin film technology is the optical quality of materials. Higher-quality materials can result in lower absorption losses and higher transmission rates, making them more effective for advanced applications.
"Thin film filters are a testament to the interplay between engineering and physics, demonstrating how intricate optical principles can yield practical technological advancements."
As thin film technology continues to evolve, understanding the principles of transmission and reflection will play a key role in advancing their application across diverse fields, from telecommunications to biomedical imaging.
Fabrication Techniques for Thin Film Filters
The fabrication techniques used in the production of thin film filters play a significant role in determining their performance and the applications they can be utilized in. Precision and control during the manufacturing process directly influence the optical characteristics, durability, and efficiency of the filters. Selecting the appropriate technique involves not only the desired optical properties but also cost, scalability, and material compatibility.
Each technique has its unique strengths and weaknesses, making it essential to consider the specific requirements of the intended application. For instance, some methods offer better uniformity, while others may excel in producing intricate multilayer designs. Understanding these various approaches enables a comprehensive grasp of how thin film filters are made and the considerations that guide these processes.
Physical Vapor Deposition
Physical Vapor Deposition (PVD) is one of the most common methods for manufacturing thin film filters. This technique involves the physical transfer of material from a solid or liquid state to the substrate, where it condenses to form a thin layer. PVD is favored for its ability to produce films with excellent uniformity and strong adhesion to the substrate.
Key advantages of PVD include:
- High Thickness Control: PVD allows for precise control over the thickness of the layers, essential for achieving the desired optical properties.
- Versatile Materials: A variety of materials, including metals, oxides, and nitrides, can be deposited using PVD techniques.
- Scalability: PVD can be scaled up for industrial applications or down for smaller-scale needs, making it versatile across different sectors.
However, some considerations include the need for a vacuum environment and potential high equipment costs.
Chemical Vapor Deposition
Chemical Vapor Deposition (CVD) is another pivotal technique in the creation of thin film filters. In this method, gaseous reactants form a solid material that deposits onto a substrate. CVD can produce high-quality films with excellent conformity, especially on complex geometries.
The benefits of CVD include:
- High Purity: The films deposited are usually of higher purity, beneficial for applications in sensitive environments.
- Excellent Conformality: CVD allows for uniform coatings on surfaces with varied topographies, crucial for intricate designs.
However, CVD can be more time-consuming and may require elevated temperatures, which can be limiting for certain substrates.
Sputtering Techniques
Sputtering techniques are widely utilized in the fabrication of thin film filters due to their versatility and ability to deposit a wide range of materials. This method involves ejecting particles from a target material that then deposit onto the substrate, forming a thin layer. Sputtering is known for producing films with excellent adhesion and uniformity.
Benefits of sputtering include:
- Material Variety: A diverse range of materials can be sputtered, allowing for tailored filter designs.
- Control Over Composition: The ability to adjust deposition parameters provides control over the film's optical properties.
Yet, there are downsides, such as lower deposition rates compared to other techniques and potential target contamination.
Spin Coating Methods
Spin coating is a technique primarily used for creating uniform thin films on flat surfaces, commonly employed in the production of optical coatings for thin film filters. In this method, a liquid material is deposited on the substrate, which is then spun rapidly to spread the liquid evenly and achieve the desired thickness.
Advantages of spin coating include:
- Ease of Use: The process is convenient and can be operated with relative simplicity.
- Cost-Effective: Compared to other techniques, spin coating can be less expensive, making it suitable for various applications.
However, it is mainly suitable for flat substrates and may not provide the same level of control for multilayer structures as other methods.
In summary, the fabrication techniques for thin film filters are critical in defining their performance and applicability. Each method has unique attributes that make it fit for specific needs in the industry.
Types of Thin Film Filters
The study of thin film filters is integral to the field of optics and photonics. Understanding the various types of these filters allows professionals and researchers to choose the right solution for specific applications. Thin film filters manipulate light in diverse ways. Their importance is highlighted in telecommunications, imaging, and various scientific instrumentation. Here, we delve into the most common types of thin film filters, focusing on their individual characteristics, benefits, and applications.
Bandpass Filters
Bandpass filters are designed to transmit light within a specific range of wavelengths while blocking others outside this range. This filtering mechanism ensures that only desired signals pass through, enhancing the clarity of transmitted data.
- Applications: Bandpass filters are widely used in telecommunications and optical instrumentation. Their role in imaging systems also cannot be understated, as they improve contrast and enhance the visibility of specific features.
- Benefits: The selective transmission of wavelengths facilitates effective signal processing and reduces noise, which is critical in many technological applications.
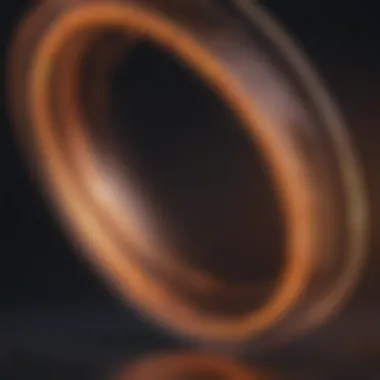
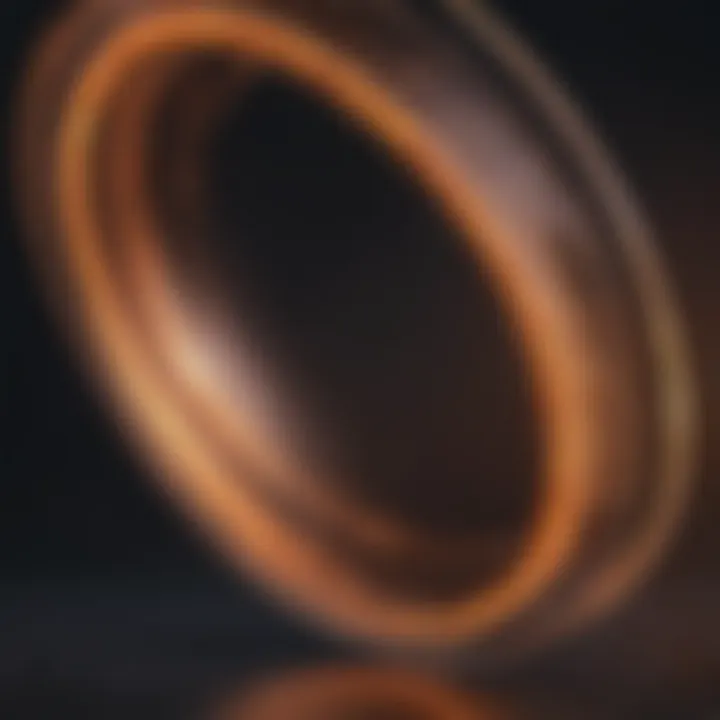
Notch Filters
Notch filters serve a precise function: they block a narrow band of wavelengths while allowing both longer and shorter wavelengths to pass with minimal loss. This distinct characteristic makes them vital in various applications where specific wavelength interference must be minimized.
- Applications: Notch filters find extensive use in scientific research, allowing instruments to avoid specific spectral lines that could interfere with measurements. They are also used in environmental monitoring and telecommunications to reject unwanted signals.
- Benefits: The precise rejection of wavelengths helps to maintain signal integrity, which is essential for accurate data collection and transmission.
Dichroic Filters
Dichroic filters are unique in that they reflect certain wavelengths while transmitting others. This capability allows for selective manipulation of light, making them invaluable in many optical applications.
- Applications: They are commonly used in projection displays, optical communications, and analytical chemistry to separate light into its constituent colors. Their usage in imaging systems is significant for enhancing color accuracy and contrast.
- Benefits: These filters offer excellent performance without significant loss of intensity, which is key in applications requiring high fidelity.
Neutral Density Filters
Neutral density filters uniformly reduce the intensity of light without affecting its color balance. By allowing specific amounts of light to pass, they help in controlling exposure in various optical systems.
- Applications: These filters are widely used in photography, cinematography, and laser applications. They help prevent overexposure and are essential for achieving optimal results under varying lighting conditions.
- Benefits: Neutral density filters provide consistency and reliability when working with different light levels, enabling photographers and technicians to manage exposure effectively.
Understanding the types of thin film filters is crucial in applying them effectively across various fields. Each filter type serves specific functions, thereby influencing the technological outcomes in telecommunications, imaging, and scientific research.
Applications of Thin Film Filters
Thin film filters exhibit a broad spectrum of applications across various domains. Their unique characteristics, such as the ability to selectively transmit or reflect specific wavelengths of light, make them valuable in both industrial and research settings. It is essential to understand these applications not only for their technical merit but also for their role in enhancing performance and efficiency in numerous systems. This section highlights how thin film filters are leveraged in telecommunication systems, biomedical imaging, consumer electronics, and astronomical observations.
Telecommunication Systems
In telecommunication, thin film filters play a critical role in signal processing. They are used to filter out unwanted wavelengths, thus enhancing the quality of the transmitted signals. The selection of specific wavelengths is particularly significant in fiber optic communications, where a precise control of light is necessary to optimize bandwidth and minimize signal loss. Thin film technology supports wavelength division multiplexing (WDM) by allowing multiple signals to be transmitted simultaneously over the same fiber without interference. This technology leads to improved data rates and more efficient use of existing infrastructure, which is crucial in an era of ever-increasing demand for bandwidth.
Biomedical Imaging Techniques
Biomedical imaging techniques benefit immensely from thin film filters. These filters are utilized in systems such as fluorescence microscopy, where they enhance contrast and improve image resolution by filtering out excess fluorescence emissions. In medical imaging, such as MRI or CT scans, thin film filters assist in isolating the relevant signals for better diagnostic capabilities. Their ability to selectively pass certain wavelengths while blocking others is vital in the analysis of biological specimens. This application underscores the importance of thin film filters not only in enhancing visualization but also in achieving precise measurements in diagnostic contexts.
Consumer Electronics
In the realm of consumer electronics, thin film filters are prevalent in devices like cameras and display screens. For instance, in digital cameras, these filters optimize color accuracy by selectively filtering certain wavelengths. The use of polarizing filters in camera lenses is a notable example, as they reduce glare from reflective surfaces and enhance image quality. In display technology, thin film coatings are employed to improve screen visibility under various lighting conditions. They facilitate better viewing experiences in smartphones, tablets, and televisions, demonstrating their significant impact on everyday technology.
Astronomical Observations
Thin film filters are also essential in astronomical observations. They allow astronomers to isolate and detect specific wavelengths emitted by celestial objects, providing valuable data for research. For instance, Hubble Space Telescope utilizes various thin film filters to capture images in different light spectra. By filtering the light coming from stars and galaxies, astronomers can gather detailed information about their composition and behavior. This application not only advances our understanding of the universe but also highlights the instrumental role of thin film technologies in scientific exploration.
"Thin film filters have revolutionized many technological fields, enabling unprecedented advancements in data transmission, medical diagnostics, consumer products, and astronomical research."
In summary, thin film filters are indispensable in numerous applications. Their capability to finely manipulate light leads to enhanced performance and greater efficiency in diverse systems. This versatility positions them as fundamental components in technology that extends beyond mere optics, influencing various industries and scientific disciplines.
Material Selection for Thin Film Filters
Selecting the right materials for thin film filters is crucial in ensuring their effectiveness across various applications. The choice of materials affects how these filters perform in terms of transmission, reflection, and durability. Different applications may require distinct optical properties, so understanding which materials meet these needs is fundamental.
Optical Properties of Materials
The optical properties of materials refer to how they interact with light. This interaction is determined by factors such as refractive index, absorption, and transmission. For thin film filters, materials must be chosen to maximize light transmission at desired wavelengths while minimizing loss.
- Refractive Index: The refractive index affects how fast light travels through a material. Filters are often designed with layers of materials that have varying refractive indexes. This creates constructive and destructive interference, allowing specific wavelengths to pass through while blocking others.
- Absorption: Some materials absorb certain wavelengths of light. High absorption at critical wavelengths must be avoided to ensure that the intended light passes through the filter.
- Durability: Materials must also be durable enough to withstand environmental factors. For instance, coating materials must resist oxidation or damage from temperature fluctuations.
Understanding the optical properties of these materials is not only relevant during filtering but also essential for applications in telecommunications and biomedical imaging.
Material Compatibility and Stability
Material compatibility refers to how well the chosen materials will perform when combined in a multilayer filter stack. Not all materials will adhere well or maintain performance in contact with others, which can lead to issues such as delamination or poor optical quality.
- Thermal Stability: It is critical to assess how materials react to temperature changes. Some materials may expand or contract differently, creating stress that can degrade filter performance.
- Chemical Compatibility: The surroundings in which filters operate can influence their long-term stability. Choosing materials resistant to chemicals, moisture, and UV exposure is vital for longevity.
- Layer Dependence: The interaction between layers can lead to unintended optical effects if materials do not complement each other. Ensuring that materials work well together is essential for maintaining performance across diverse applications.
Compatibility and stability in the materials used for thin film filters significantly impact their operational lifespan and effectiveness. Choosing wisely can lead to better reliability and performance in practical applications.
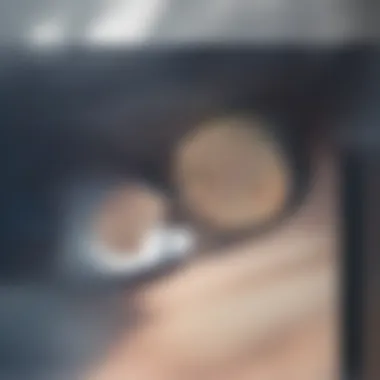
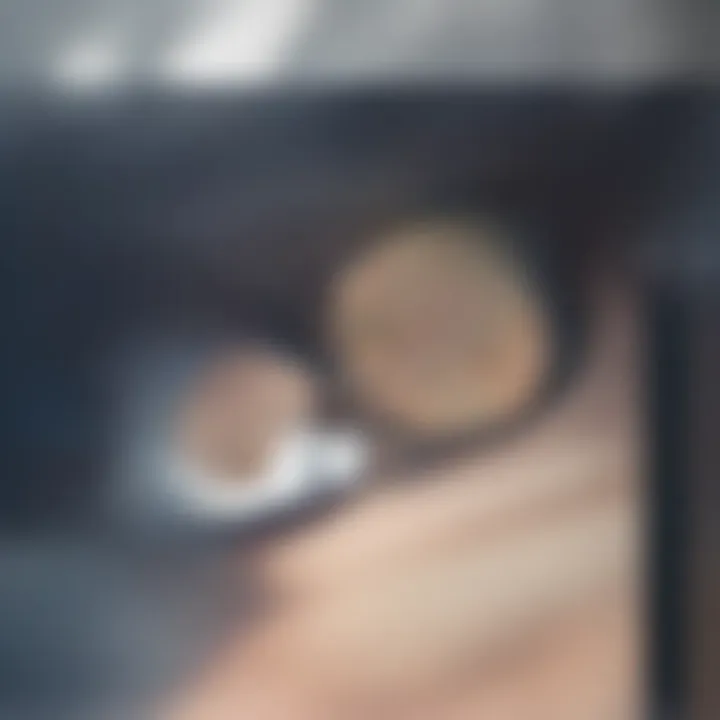
Characterization and Testing of Thin Film Filters
Characterization and testing of thin film filters are critical components in the development and application of these optical devices. They provide essential insights into the performance and effectiveness of thin film coatings across various applications. Understanding the physical and optical properties of thin film filters ensures that they meet the required specifications and can function as intended in their specific roles, from telecommunications to biomedical imaging.
The significance of precise characterization lies in its ability to help manufacturers and researchers identify the best materials and fabrication methods that align with the desired outcomes. Testing procedures not only validate the design but also uncover potential defects or weaknesses that could compromise performance. This vigilance in characterization contributes to the reliability of numerous technologies that rely on thin film filters.
Optical Transmission Measurements
Optical transmission measurements assess how much light passes through a thin film filter. These measurements are vital for determining the filter's efficiency and performance. To conduct these measurements, a light source emits light towards the filter, and detectors capture the transmitted light. This data reveals how well the filter selectively transmits certain wavelengths while blocking others.
A few notable points about optical transmission measurements include:
- The parameters of interest often include the transmission spectrum, which displays the percentage of light transmitted at different wavelengths.
- High transmission levels at desired wavelengths signify effective filter design, while low transmission levels indicate that the filter is absorbing or reflecting the light instead.
- Repeated testing may be required to confirm the filterโs consistency under varying conditions.
Accurate optical transmission measurements can inform future improvements in thin film technology, impacting areas such as sensor design and imaging systems.
Reflectance Characterization
Reflectance characterization investigates how much light is reflected off the surface of a thin film filter. This measurement is equally important as it helps to understand how well the filter operates in terms of reflection and its ability to prevent unwanted wavelengths from passing through. Much like transmission measurements, reflectance data provide a complete picture of the filter's performance.
Reflectance values are determined similarly to transmission measurements; a light beam is directed at the filter, and the reflected light is measured with a detector. Key aspects of reflectance characterization include:
- The reflectance spectrum illustrates the percentage of light reflected across different wavelengths. This data is crucial for assessing filter performance.
- High reflectance levels indicate that the filter is effectively blocking unwanted wavelengths, which is essential in applications like optical coatings.
- These measurements may be influenced by factors such as angle of incidence, which should be accounted for to obtain accurate results.
Reflectance characterization contributes to refinements in the design and application of thin film filters, influencing their effectiveness across various sectors. Understanding both transmission and reflectance is vital in optimizing the performance of thin film filters, ultimately leading to advancements in optical technology.
Future Trends in Thin Film Filter Technologies
The rapid advancement of technology necessitates continual evolution in materials and methods used in thin film filters. Understanding these future trends is crucial, as they have direct implications on performance across various applications. The importance is multifaceted, involving enhancements in efficiency, adaptability, and integration into advanced systems. Future developments are likely to influence sectors from telecommunications to biomedical optics, highlighting the need for relevant research and application.
Advancements in Material Science
Material science is at the forefront of evolution in thin film technology. Researchers are focusing on novel materials that present improved optical characteristics. One area of concern is the reduction in production costs while maintaining high performance. This balance is vital for industries where margins are tight. New compositions also bring about improved durability and environmental stability, essential for outdoor applications.
Developments in nanotechnology play a key role as well. By manipulating materials at the nanoscale, scientists are improving filter efficiency. Examples include:
- Layered Coatings: Allowing for a more controlled interaction of light with the filter.
- Hybrid Materials: Combining organic and inorganic compounds to create unique properties.
Moreover, flexible and lightweight materials are becoming more common. This shift allows for easier incorporation into various devices, enhancing user experience and functionality.
Integration with Photonic Devices
Integration with photonic devices is another area garnering attention. As technologies evolve, the need for compatibility between thin film filters and photonic platforms is increasingly significant. Photonic devices, which manipulate light in various ways for applications such as signal processing, rely heavily on filters to achieve desired outcomes. The development of integrated photonic circuits is an area where thin film filters can play a critical role.
The integration process can lead to advantages like:
- Reduced Size: As devices become more compact, incorporating filters directly into circuits becomes vital.
- Enhanced Performance: Seamless interaction between components can boost overall device efficiency.
Challenges remain, such as ensuring that the filters maintain their integrity and performance when embedded within photonic systems. Ongoing research into compatible materials and methods is key to overcoming these hurdles, leading to potentially revolutionary applications in various fields.
"As technology progresses, the integration of thin film filters with photonic devices represents an essential frontier in optical engineering."
In summary, the future trends in thin film filter technologies highlight advancements in material science and integration with photonic devices. These developments promise to enhance existing applications and pave the way for new innovations in the optical domain, ultimately contributing to a more efficient technological landscape.
The End
The conclusion serves as a crucial element of this article, encapsulating the core insights gained about thin film filters. It emphasizes the principles, applications, and technological nuances of these filters, creating a comprehensive picture of their importance in various domains.
In summary, thin film filters are not just optical devices; they play a vital role in enhancing the capabilities of numerous systems, from telecommunications to biomedical imaging. Each type of filterโwhether bandpass, notch, or dichroicโoffers unique functionalities that cater to specific needs. Their fabrication methods, such as physical vapor deposition and sputtering, underline the sophistication involved in producing these essential components.
Summary of Key Points
- Definition and Purpose: Thin film filters manipulate light in precise ways, allowing selective transmission or reflection.
- Fabrication Techniques: Various methods such as chemical vapor deposition and spin coating are employed in their creation.
- Types of Filters: Each filter type addresses different optical requirements; examples include bandpass filters for specific wavelength transmission and notch filters for rejecting certain wavelengths.
- Broad Applications: Thin film filters find usage across diverse fields, such as enabling clean signals in telecommunications and enhancing image quality in biomedical imaging tools.
- Future Trends: Advances in material science and integration with photonic devices hint at ongoing innovations that will push the boundaries of what these filters can achieve.
Implications for Future Research
The study of thin film filters presents several avenues for future research. One prominent area is the advancement of materials, focusing on developing new composites that could enhance optical performance and resilience. This could lead not only to better filter efficiency but also to broader application ranges, extending into novel technologies.
Furthermore, integrating these filters with emerging photonics devices presents opportunities for groundbreaking discoveries. Techniques such as machine learning may assist in optimizing filter designs based on specific performance needs. The convergence of these fields is an exciting prospect for both academic research and applied technology.
As researchers and professionals explore these frontiers, understanding and innovating within the realm of thin film filters will remain integral to the evolution of optical technologies.