Understanding Thermal Runaway: Mechanisms and Implications
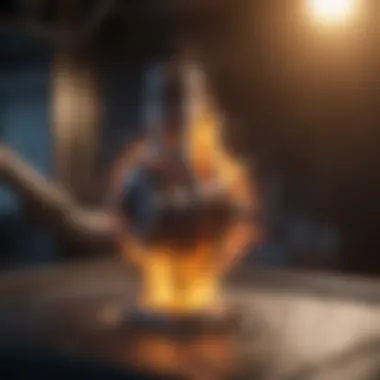
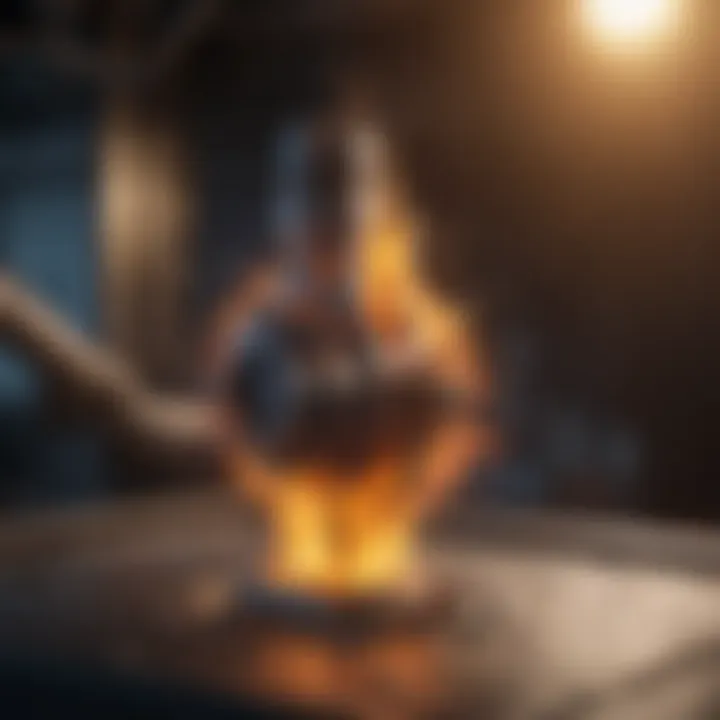
Intro
Thermal runaway is a phenomenon with significant implications in various fields, especially in chemistry and engineering. It occurs when an increase in temperature leads to further reactions that produce even more heat, creating a self-perpetuating cycle. Understanding this process is critical for ensuring safety in industrial applications, managing chemical reactions, and mitigating risks associated with energy storage systems like batteries.
In this article, we will explore the fundamental mechanisms that contribute to thermal runaway, discuss its potential hazards, and examine methods to control and prevent its occurrence. By shedding light on the underlying principles, we aim to provide a thorough understanding of thermal runaway for students, researchers, educators, and professionals alike.
Research Overview
Summary of Key Findings
Thermal runaway exhibits complex behaviors influenced by a range of factors, including reaction kinetics, material properties, and environmental conditions. Key findings indicate that specific conditions such as heat accumulation, insufficient cooling, and exothermic reactions are major contributors to the phenomenon. The article will elaborate on how these factors interplay, leading to different scenarios across various scientific and engineering contexts.
Methodologies Employed
To analyze thermal runaway, numerous methodologies can be utilized. Experimental approaches often involve calorimetry to measure heat generation rates during chemical reactions. Simulations using computational models help in predicting potential runaway conditions under varying parameters. These methods provide a robust framework for studying thermal runaway and assessing its risks effectively.
In-Depth Analysis
Detailed Examination of Results
The results of studies on thermal runaway reveal that certain variables like temperature thresholds and concentration of reactants play pivotal roles in determining the likelihood of a runaway scenario. Often, when substances are heated beyond their stable temperature ranges, they may undergo rapid decomposition or react further. This section will detail the various studies and their findings, highlighting critical data that underscores the urgency of managing thermal runaway risks in practical applications.
Comparison with Previous Studies
Previous research has laid a foundation for our understanding of thermal runaway. By examining historical cases, such as battery fires or chemical plant accidents, we can compare results of recent studies against established theories. We aim to identify trends in findings, including advances in safety measures and materials designed to mitigate risk.
"Understanding thermal runaway is not just relevant for scientific curiosity but is crucial for ensuring safety in industrial operations where heat can lead to catastrophic failures."
A comprehensive understanding equips professionals to develop effective strategies for risk management and intervention in various fields.
Foreword to Thermal Runaway
Thermal runaway is a critical phenomenon that warrants significant attention in the fields of chemistry, engineering, and safety management. Understanding this concept is vital not only for researchers and professionals but also for students and educators who seek a foundational comprehension of safety-related issues in various scientific applications.
The importance of thermal runaway lies in its potential consequences. It can lead to catastrophic failures in a variety of systems, particularly those involving batteries, chemical reactions, and heat-generating processes. Awareness of thermal runaway enhances risk assessment, promotes safety protocols, and fosters the development of preventative measures.
Definition and Importance
Thermal runaway refers to a condition where an increase in temperature leads to further increases in temperature, often resulting in uncontrolled reactions. This self-perpetuating cycle can arise from exothermic reactions or inadequate cooling mechanisms. The significance of understanding thermal runaway is underscored by its implications for safety, system design, and environmental concerns. By recognizing the factors that contribute to this phenomenon, stakeholders can implement appropriate safeguards, preventing disastrous outcomes.
For example, in lithium-ion batteries, thermal runaway can result in fires or explosions, presenting risks in consumer electronics and electric vehicles. An understanding of how thermal runaway occurs enhances product safety and regulatory compliance, ensuring public trust and reliability in technologies that use these batteries.
Historical Context and Case Studies
The historical context of thermal runaway illustrates its far-reaching implications across industries. Notable incidents highlight the necessity for stringent safety standards and effective engineering solutions. One such case is the Bhopal gas disaster in 1984, where a chemical reaction led to a massive release of toxic gas, highlighting the catastrophic potential of uncontrolled exothermic reactions.
Another example is the Boeing 787 Dreamliner battery issues in 2013, where overheating batteries caused several incidents of smoke and fire. Investigations revealed that thermal runaway in lithium-ion batteries was a key factor. These case studies emphasize learning from past failures and the crucial nature of thorough understanding of thermal runaway mechanisms to prevent similar tragedies in the future.
Understanding thermal runaway is essential for the development of effective safety measures across various applications.
In summary, a detailed grasp of thermal runaway is not just an academic exercise; it is crucial in the real world. It affects how systems are engineered, how products are manufactured, and how safety protocols are established and enforced.
By integrating this knowledge into practice, individuals and organizations can work towards minimizing risks and ensuring a safer environment in laboratories, factories, and beyond.
The Mechanism of Thermal Runaway
Thermal runaway is a critical phenomenon, underpinned by complex mechanisms that require thorough exploration. Understanding these mechanisms is essential not only for managing risks but also for enhancing safety protocols across various scientific and industrial fields. For instance, analyzing how heat accumulates and leads to uncontrollable reactions helps researchers design better containment systems. It also provides clarity in evaluating existing infrastructures, which may be vulnerable to these occurrences.
Exothermic Reactions
Exothermic reactions serve as the primary trigger for thermal runaway. These types of reactions release energy in the form of heat. Understanding how these reactions function is crucial in predicting thermodynamic stability. For example, in a lithium-ion battery, the chemical processes can produce excessive heat when the cell is charged. If this heat is not effectively managed, it can initiate a self-perpetuating cycle of more heat production, culminating in a hazardous situation.
The heat released during exothermic reactions might initially be dissipated through conduction and convection processes. However, if these pathways are insufficient, the temperature can rise dramatically. Manufacturers must consider materials and designs that either minimize heat generation or improve heat dissipation to avoid the risks associated with thermal runaway.
Heat Generation and Accumulation
When discussing thermal runaway, heat generation and accumulation are pivotal concepts. In many systems, particularly those involving batteries or certain chemical processes, heat builds up over time. This buildup can be due to several factors including chemical reaction rates and low thermal conductivity of materials. Itβs important to highlight that heat accumulation can reach a point where it surpasses the systemβs ability to cool itself.
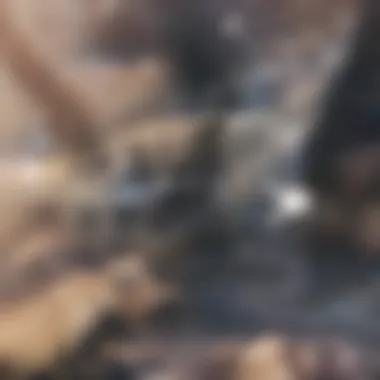
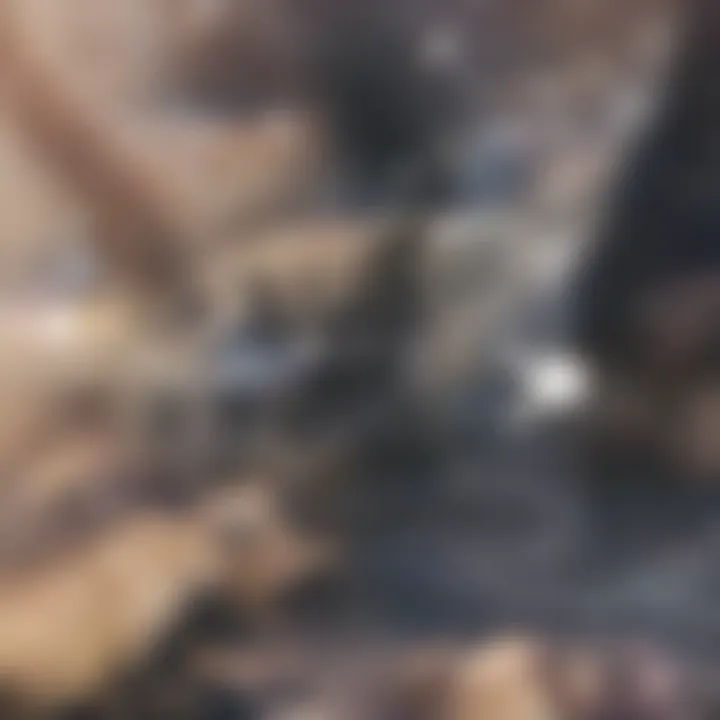
Effective heat management practices must be established to mitigate this risk. For instance, thermal insulation may be adjusted to ensure heat does not concentrate in a specific area. Likewise, incorporating cooling mechanisms into equipment can facilitate more efficient heat dissipation, thus preserving system integrity.
"Effective thermal management can significantly lower the risks of thermal runaway events, enhancing safety in various applications."
Feedback Loops
Feedback loops are another major mechanism associated with thermal runaway. Once a reaction starts generating heat, it can create a positive feedback scenario where increased temperature accelerates the reaction, leading to more heat generation. This self-reinforcing cycle can spiral out of control if left unchecked.
A classic example of feedback loops can be seen in geological processes, such as in thermal vents, where heat causes the surrounding materials to undergo changes that further enhance heat generation. In industrial applications, it becomes imperative to establish control measures that can interrupt this loop. Implementing automatic shutdown systems can provide a fail-safe to halt operations when critical temperature thresholds are reached. Moreover, operator training is vital in recognizing the onset of these loops to enable timely intervention.
In summary, understanding the mechanisms of thermal runawayβspecifically exothermic reactions, heat generation, and feedback loopsβprovides fundamental insight into preventing dangerous events. By knowing how to manage heat and recognize the symptoms of feedback loops, stakeholders can greatly reduce the associated risks.
Factors Influencing Thermal Runaway
In understanding thermal runaway, it is crucial to assess the factors that can instigate or exacerbate this phenomenon. These factors play a significant role in determining the conditions under which thermal runaway occurs. By examining these elements, individuals can gain a deeper comprehension of the risks and mitigation strategies associated with this complexity in chemical and engineering systems.
Material Properties
Material properties significantly influence the likelihood of thermal runaway. These include thermal conductivity, specific heat capacity, and chemical reactivity. For instance, materials with low thermal conductivity tend to trap heat, increasing the chances of overheating. Conversely, materials with high heat capacity can absorb significant amounts of energy, mitigating rapid temperature increases. Furthermore, the purity and composition of a material can affect its reactivity. Impurities or additives might potentially engage in exothermic reactions that can lead to thermal runaway if not managed correctly.
- Thermal Conductivity: Poor heat dissipation can lead to localized heating.
- Chemical Composition: Certain combinations can create unstable reactions that release heat quickly.
- Phase Changes: Changes in a materialβs state can also contribute to heat release.
Proper evaluation of these properties during the design phase of any system is essential to minimize risks associated with thermal runaway.
Environmental Conditions
Environmental conditions are another critical influencer of thermal runaway scenarios. Factors such as temperature, pressure, and humidity can alter material behavior significantly. For example, elevated temperatures may accelerate reaction rates, while high pressures can amplify the energy release during a reaction.
Temperature is often the most crucial factor. As ambient temperatures increase, the risk of reaching a critical threshold where thermal runaway may occur also rises. Additionally, humidity can affect the properties of materials, causing changes in both structural integrity and reactivity.
Some considerations are:
- Ambient Temperature: Higher temperatures can reduce heat dissipation.
- Pressure: Increased pressure might escalate the reaction intensity.
- Humidity: Can influence the stability of certain chemical properties.
Understanding these environmental factors is essential for designing safe systems in various industries, from battery manufacturing to chemical processing.
System Design and Configuration
The design and configuration of a system directly impact the occurrence of thermal runaway. Proper engineering controls can prevent excessive heat buildup and facilitate safe operation. Key aspects include the arrangement of components, the containment of reactions, and the implementation of safety features.
For example, incorporating cooling systems can effectively manage heat removal during exothermic reactions. Also, redundant safety measures can help ensure that even if one system fails, backup systems can mitigate risks.
Key elements in system design include:
- Cooling Mechanisms: Such as heat exchangers or ventilation systems.
- Isolation Techniques: To prevent heat spread between reactive components.
- Monitoring Systems: Incorporating real-time data acquisition enables timely responses to any anomalies.
Evaluation of these design elements is paramount and should aim to minimize the risk of thermal runaway in modern technologies.
Consequences of Thermal Runaway
The implications of thermal runaway extend beyond a simple scientific curiosity. Understanding its consequences is essential for avoiding catastrophic failures in various applications. Addressing the consequences involves examining safety risks, impacts on equipment and infrastructure, and environmental concerns. Each of these elements not only affects individual systems but also has broader implications on public safety and environmental stewardship.
Safety Risks and Hazards
Thermal runaway presents significant safety risks, particularly in environments where reactive chemicals or high-energy devices operate. The elevated temperatures associated with thermal runaway can lead to uncontrolled reactions that may result in fires, explosions, or toxic gas emissions. The severity of these risks demands that serious consideration be given during the design phase of equipment.
To illustrate this, the case of lithium-ion batteries underscores the safety hazards present in modern technology. In instances of overheating, the battery can enter thermal runaway, leading to fires that are difficult to extinguish. These batteries are widely used in consumer electronics, electric vehicles, and renewable energy storage. Not only do they pose a risk to the devices they power, but they also endanger users and first responders. The potential for physical harm necessitates stringent safety protocols, along with proper training for personnel handling such materials.
Impact on Equipment and Infrastructure
The consequences of thermal runaway are not solely limited to human safety; they also extend deep into the reliability of equipment and the integrity of infrastructure. An incident of thermal runaway can result in significant damage to apparatus involving sensitive chemical processes or electronic systems. Breakdown of machinery can lead to costly repairs, prolonged downtimes, and even the complete loss of equipment.
Moreover, infrastructure can suffer from the indirect repercussions of thermal runaway incidents. For instance, in chemical processing facilities, an uncontrolled exothermic reaction can necessitate the shut down of entire sections of a plant, disrupting operations and affecting productivity. Equipment not built with the appropriate safety features may contribute to more severe repercussions, thus highlighting the importance of incorporating robust engineering controls.
Environmental Concerns
The potential environmental implications of thermal runaway incidents require careful analysis. When thermal runaway leads to chemical spills or fires, the resulting pollutants can contaminate air, soil, and water resources. Dangerous chemicals may escape into the atmosphere, contributing to air quality degradation and posing risks to human health.
Research suggests that industrial accidents linked to thermal runaway can result in long-term ecological damage. For example, hazardous substances that enter waterways through runoff can disrupt aquatic ecosystems, affecting wildlife and potentially leading to public health issues. Addressing these concerns is paramount in our increasingly industrialized world.
Awareness and prevention can significantly reduce the risks associated with thermal runaway, ensuring safer outcomes in various applications.
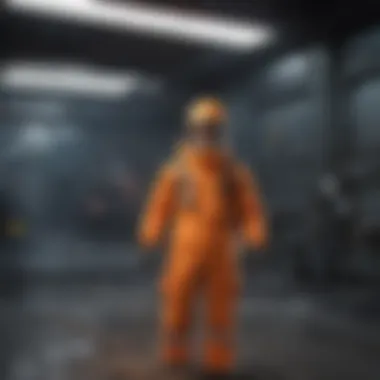
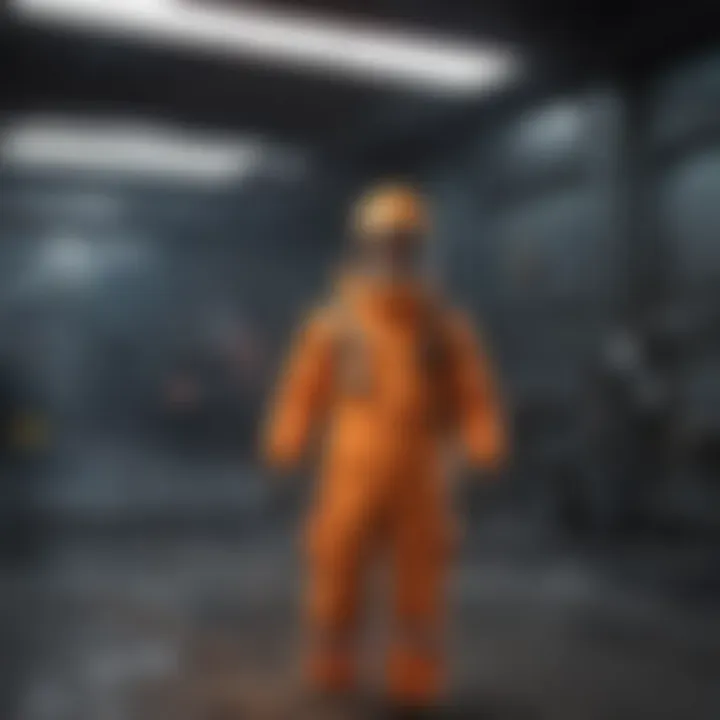
Examples of Thermal Runaway Events
Understanding the real-world implications of thermal runaway is crucial for a comprehensive analysis of this phenomenon. Examples of thermal runaway events serve to illustrate the potential hazards and consequences associated with this process across different disciplines, particularly in the realms of energy storage and chemical manufacturing. By studying specific incidents, researchers and professionals can gain valuable insights into failure mechanisms, improve safety protocols, and refine technological designs to avert future disasters. This section highlights significant case studies that underline the critical importance of managing thermal runaway effectively.
Battery Fires and Explosions
Battery fires and explosions have become more common, especially in applications involving lithium-ion batteries. These batteries are favored for their lightweight nature and high energy density, which appear ideal for consumer electronics and electric vehicles. However, they are also susceptible to thermal runaway due to several factors, such as internal short circuits, manufacturing defects, or extreme temperature conditions.
Once the thermal runaway process starts, it leads to the rapid generation of heat and gas. It can escalate quickly, leading to fires or even explosions. An infamous example of this include the incidents involving the Samsung Galaxy Note 7, which faced critical heat failures, resulting in widespread recalls. Such occurrences highlight the need for rigorous safety measures, including better battery design, improved thermal management systems, and thorough testing protocols.
"Understanding the mechanisms behind battery thermal runaway helps mitigate associated risks and enhances the overall safety of energy storage systems."
Additionally, researchers must focus on developing new materials that can withstand higher temperatures and improve the overall stability of batteries under varying environmental conditions. This proactive approach helps in reducing the likelihood of thermal runaway events in the future.
Chemical Process Incidents
Chemical process incidents represent another critical area where thermal runaway poses significant risks. In industrial settings, from petrochemical to pharmaceutical manufacturing, the reactions often involve exothermic processes. If not properly controlled, these reactions can lead to severe consequences, including explosions and fires.
A notable example is the 2004 explosion at the Bayer CropScience plant in West Virginia. This incident was attributed to a runaway reaction involving methyl isocyanate, a highly reactive and toxic chemical. A lack of adequate safety systems and monitoring led to catastrophic results, causing loss of life and extensive damage.
To prevent such incidents, it is essential to implement rigorous safety protocols and risk assessment measures. Identifying potential triggers for thermal runaway, such as equipment failure or inadequate cooling systems, can enhance safety and operational reliability. Incorporating advanced monitoring technology can also allow for timely interventions when deviations from normal operating parameters occur.
By exploring these examples, professionals can adopt lessons learned to improve safety practices and reduce risks associated with thermal runaway in both batteries and chemical processing. This knowledge not only informs policy but also paves the way for innovations that prioritize safety in technologies where thermal runaway could have dire consequences.
Preventative Strategies
Understanding the preventative strategies related to thermal runaway is vital for reducing risks and enhancing safety across various industries. Effective preventative measures can significantly mitigate the likelihood of thermal runaway events. This not only safeguards human life but also protects valuable infrastructure and the environment.
Risk Assessment Protocols
Risk assessment protocols are foundational to any strategy aimed at preventing thermal runaway. These protocols evaluate the likelihood and potential impacts of thermal runaway incidents under various operational scenarios.
- Identification of Hazards: This involves cataloging all materials and processes that could lead to thermal runaway. For instance, in battery manufacturing, identifying specific chemistries and configurations that pose a risk is crucial.
- Likelihood Evaluation: Once hazards are identified, assessing the probability of these risks occurring helps in prioritizing management strategies.
- Impact Assessment: It's important to estimate the consequences should a thermal runaway event occur. This encompasses the scale of potential damage and risks to human safety.
- Regular Reviews: Risk assessments should not be one-time events. Regular updates ensure that new materials or changes in processes are accounted for.
Implementing a comprehensive risk assessment protocol supports informed decision-making.
Engineering Controls
Engineering controls play a crucial role in preventing thermal runaway. These controls are designed to reduce or eliminate hazards from the workplace.
- Design Modifications: For example, battery systems can be engineered with thermal management features such as advanced cooling systems. This helps in dissipating heat effectively before it can escalate to a runaway condition.
- Containment Systems: Utilizing containment structures can prevent the spread of thermal runaway reactions. This is particularly important in chemical manufacturing, where reactions can quickly escalate.
- Automated Monitoring: Continuous monitoring systems can detect abnormalities such as excessive temperature or pressure. Timely alerts can facilitate rapid responses before situations escalate.
- Redundant Safety Mechanisms: Implementing multiple safety layers can provide additional assurance against failures. If one control measure fails, others can step in to mitigate risks.
These engineering solutions can significantly decrease the chances of thermal runaway incidents.
Emergency Response Measures
Preparedness for unexpected scenarios is essential in managing thermal runaway risks. Emergency response measures are designed to address incidents promptly to minimize damage and safeguard lives.
- Training and Drills: Regular training sessions for personnel on how to respond to thermal runaway incidents can enhance preparedness. Drills simulate emergency scenarios and allow teams to practice their responses.
- Clear Communication Plans: Establishing communication protocols ensures everyone knows their roles during an emergency. This reduces confusion and response times.
- Access to Emergency Resources: Ensuring availability of firefighting equipment, personal protective equipment (PPE), and first aid kits is crucial for effective responses. Teams should be well-acquainted with the location and use of these resources.
- Coordination with Local Authorities: Establishing links with local emergency services allows for better integration and support during serious incidents.
In summary, strategic emergency preparedness helps in managing the consequences of thermal runaway, thus enhancing overall safety.
Thermal Runaway in Modern Technologies
Thermal runaway represents a critical concern in modern technologies, especially as reliance on energy storage systems, electrical devices, and industrial applications increases. Technology is rapidly advancing, and with it, the potential risks associated with thermal runaway are growing. This section explores the specific elements and implications of thermal runaway across different facets of technology, highlighting both benefits and considerations.
Energy Storage Systems
Energy storage systems, particularly lithium-ion batteries, are fundamental in todayβs tech-driven world. They power everything from mobile phones to electric vehicles. However, these systems are susceptible to thermal runaway, which can lead to catastrophic failures.
The importance of understanding thermal runaway in energy storage cannot be overstated. Events like the Samsung Galaxy Note 7 recall exemplify how battery failures can lead to fires and explosions, highlighting the need for rigorous safety protocols. The exothermic reactions within these batteries, when not properly managed, generate heat beyond safe limits, triggering a feedback loop.
Key considerations for mitigating thermal runaway include:
- Battery Design: Incorporating materials that have better thermal management properties can reduce risks.
- Monitoring Systems: Implementing real-time temperature sensors can help detect early signs of thermal issues.
- Safety Protocols: Regular inspections and adherence to safety standards are crucial in averting incidents.
"Understanding how thermal runaway occurs in energy storage systems is vital for building safer technologies in the future."
Electrical and Electronic Devices
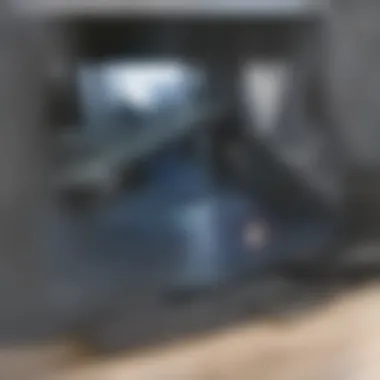
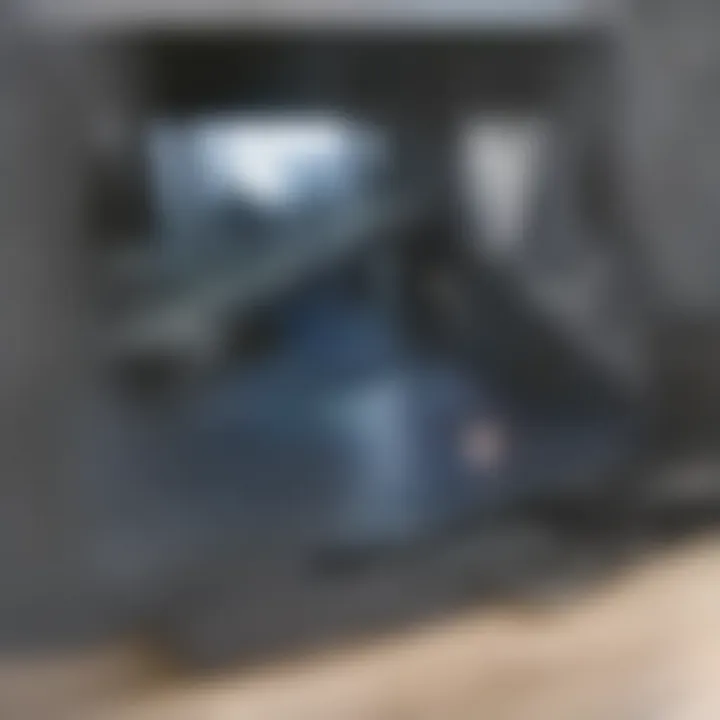
The realm of electrical and electronic devices also faces challenges related to thermal runaway. These devices use compact materials that can overheat under certain conditions, leading to circuit failures or fires. As devices become more powerful and miniaturized, the potential for thermal runaway increases.
For example, consumer electronics like laptops and tablets are designed with cooling systems to dissipate heat, yet excessive usage or faulty components can still lead to thermal runaway. The implications are serious, as failures can damage not only the devices but also pose safety risks to users.
To combat potential thermal runaway scenarios, it is necessary to:
- Enhance Cooling Systems: Use advanced materials and designs that facilitate better heat dissipation.
- Implement Fail-safes: Designs should include shutdown mechanisms to prevent overheating.
- User Education: Consumers need awareness of safe usage practices to minimize risk.
Industrial Applications
In industrial settings, thermal runaway can have dire consequences, directly impacting safety and productivity. Processes that involve chemical reactions or combine multiple materials can create conditions favorable for thermal runaway. For instance, excessive heat generation in reactors can lead to explosions and equipment failure, impacting both human safety and operational integrity.
Industries should emphasize:
- Process Control: Advanced monitoring and control methodologies are essential in identifying potential risks.
- Training and Awareness: Workers should understand thermal dynamics related to their tasks and appropriate emergency procedures.
- Regulatory Compliance: Adhering to industry standards and protocols can significantly reduce risks associated with thermal runaway.
Regulatory Framework
The regulatory framework surrounding thermal runaway plays a significant role in ensuring safety across multiple industries. These regulations help to mitigate the risks associated with thermal runaway events, particularly in environments where chemicals and energy systems are used. The development and enforcement of these regulations are essential for protecting both human health and the environment.
Standards and Guidelines
Standards and guidelines provide essential protocols that organizations must follow to manage the risks of thermal runaway. Such standards may originate from international bodies like the International Organization for Standardization (ISO) or national agencies like the Occupational Safety and Health Administration (OSHA) in the United States. These documents outline best practices in handling, storing, and processing materials that are prone to thermal runaway.
Key guidelines often cover:
- Material Safety Data Sheets (MSDS): It is crucial that these documents are readily available, offering information about materials that can lead to thermal runaway, including handling instructions and emergency measures.
- Design Standards: Engineering practices that specify safer design methodologies for equipment susceptible to overheating incidents.
- Testing Protocols: Specifications for assessing the thermal stability of materials and ensuring that systems can handle exothermic reactions.
These elements help companies minimize the likelihood of thermal runaway, fostering a safer workplace and compliant manufacturing processes.
Compliance and Enforcement
Compliance ensures that organizations adhere to established standards and guidelines, which is critical for preventing thermal runaway incidents. Regulatory agencies enforce adherence to these regulations through various measures, including inspections, audits, and penalties for non-compliance.
Responsibilities of compliance might include:
- Regular Inspections: Agencies often conduct routine checks to ensure that businesses follow safety protocols and maintain the integrity of their thermal systems.
- Reporting Requirements: Organizations may be required to submit detailed reports on their safety measures and any incidents that occur, contributing to a larger database on thermal runaway occurrences.
- Training Programs: Providing access to training for employees on safety measures related to thermal runaway, ensuring that staff are educated on how to recognize and respond to risks.
"Monitoring and compliance mechanisms are essential to mitigate risks from thermal runaway, driving improvements in safety culture."
In summary, an effective regulatory framework is vital for managing thermal runaway risks. Standards and guidelines inform safe practices, while compliance and enforcement ensure adherence to these protocols. Together, they form a barrier against potential hazards, safeguarding lives and protecting the environment.
Future Research Directions
Future research directions in thermal runaway are crucial to understanding and preventing this phenomenon across various applications. As industries innovate, the importance of developing effective solutions and technologies becomes even more pronounced. Expanding our knowledge in this field helps mitigate risks associated with thermal runaway events and enhance overall safety. Researchers need to focus on several areas that hold promise for significant improvements and advancements.
Innovations in Safety Technology
Safety technology plays a vital role in preventing thermal runaway incidents. Innovations in this area are focused on enhancing existing control measures. For example, integrating better heat dissipation mechanisms in batteries can significantly reduce the risk of heat accumulation. Improved materials and design modifications in chemical processing equipment can also prevent runaway reactions. Emerging technologies like advanced thermal sensors and active cooling systems are being explored to provide real-time feedback.
Investing in this research not only improves safety but also boosts public confidence in technological applications. It promotes a culture of safety which is essential for wide-scale adoption of new technologies.
Improved Monitoring Systems
Monitoring systems are imperative for detecting early signs of thermal runaway. Current technologies often lack the capability to provide timely warnings about critical changes in system behavior. Future research should focus on developing improved monitoring systems that utilize better algorithms and sensors. These systems must offer a higher resolution of data and the ability to analyze trends over time.
Having a robust monitoring system can prevent minor issues from escalating into serious incidents. Researchers should also consider the integration of predictive analysis to foresee potential runaway events based on historical data patterns. This approach could potentially save lives and reduce costs associated with thermal runaway incidents.
Role of Artificial Intelligence
Artificial Intelligence (AI) is emerging as a powerful tool in various domains, including thermal runaway research. AI can analyze vast amounts of data rapidly, making it easier to identify risk factors associated with different systems. Machine learning algorithms can be trained to predict when thermal runaway might occur based on input parameters such as temperature, pressure, and reactant concentrations.
Additionally, AI can help refine the design of systems by simulating different conditions and predicting outcomes. The role of AI in improving safety protocols cannot be underestimated. As the technology advances, its implementation in monitoring and response systems will likely become more widespread, dramatically reducing risks associated with thermal runaway events.
The End
Understanding thermal runaway is essential for various scientific fields, particularly in chemistry and engineering. This article highlights the key mechanisms and factors influencing this phenomenon, emphasizing safety, prevention, and implications for real-world applications.
Summary of Key Points
- Definition and Importance: The article starts with a clear definition of thermal runaway, establishing its significance in both research and industry. It explains how thermal runaway can escalate into catastrophic incidents.
- Mechanisms: The mechanisms that contribute to thermal runaway are dissected in detail. The processes of exothermic reactions, heat generation, and feedback loops are crucial in understanding how thermal runaway can occur under specific conditions.
- Influencing Factors: Various factors, such as material properties, environmental conditions, and system design, are explored to highlight how they affect the likelihood of thermal runaway events.
- Consequences: The potential consequences of thermal runaway are serious. These include safety risks, financial impacts on equipment, and environmental concerns.
- Preventative and Regulatory Measures: The article outlines a range of preventative strategies such as risk assessment and engineering controls, discussing the importance of regulatory frameworks to ensure compliance and safety.
- Future Directions: Looking ahead, the need for innovations in safety and monitoring systems is emphasized, hinting at the role of artificial intelligence in managing and predicting thermal runaway events.
Final Thoughts on Thermal Runaway
Thermal runaway is a multifaceted issue that requires ongoing research and attention. With advancements in technology and a growing understanding of materials, strategies have improved to mitigate risks associated with this phenomenon. The implications of thermal runaway extend beyond individual incidents to influence broader industrial standards and safety protocols. It is vital for professionals in the field to stay informed about evolving practices and to contribute to enhancing safety measures. By fostering a culture of awareness and preparedness, the industry can better handle the complexities of thermal runaway, preserving both safety and efficacy in applications.