Understanding Oxygen Energy: Implications and Applications
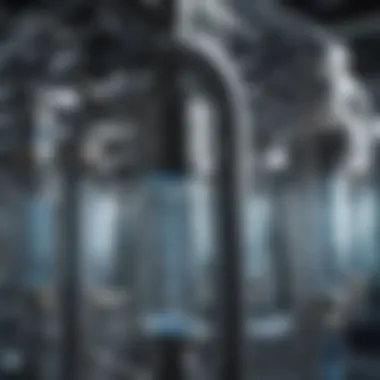
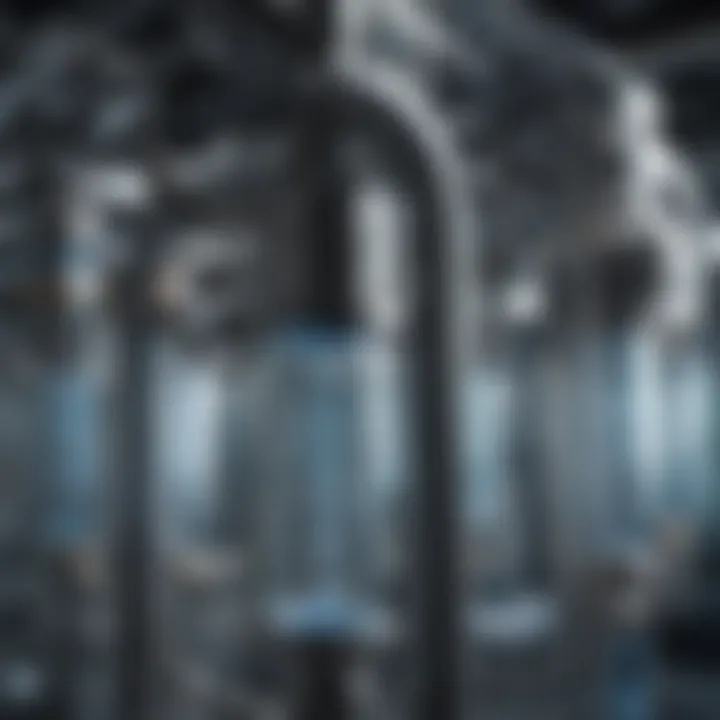
Intro
Oxygen is more than just a necessary element for human survival; it plays a crucial role in various energy systems. This article investigates how oxygen impacts energy generation, storage, and sustainability. Understanding these relationships can help researchers and professionals develop innovative solutions to modern energy problems. As the world grapples with climate change and resource depletion, oxygen energy's significance has never been more pronounced.
Research Overview
Summary of Key Findings
Research indicates that oxygen not only facilitates respiration in living organisms but also acts as a vital component in numerous energy production processes. For instance, oxygen is essential for combustion engines and fuel cells. These technologies capitalize on oxygen to release energy from fuels through oxidation reactions. Additionally, oxygen sustainable methods such as oxygen production from algae open new avenues for renewable energy sources.
Methodologies Employed
In investigating the role of oxygen in energy systems, researchers utilized various methodologies:
- Experimental Studies: Laboratory experiments that examine reactions involving oxygen to understand energy release mechanisms.
- Theoretical Models: Simulations that assess oxygenβs impact on energy efficiency.
- Field Studies: Real-world applications where oxygen is utilized in sustainable practices, like bioenergy production.
These approaches help shape a comprehensive understanding of oxygen's utility in both traditional and innovative energy systems.
In-Depth Analysis
Detailed Examination of Results
The results from these studies reveal significant insights into how oxygen enhances energy efficiency. For instance, in fuel cells, the reaction between hydrogen and oxygen produces electricity and water as a byproduct. This not only confirms oxygen's viability in clean energy applications but also offers a sustainable alternative to fossil fuel dependence.
Comparison with Previous Studies
Previous studies primarily focused on fossil fuels and alternative energy sources. However, newer research emphasizes integrating oxygen into renewable energy frameworks. Comparative analysis shows a shift in focus:
- Earlier methods relied heavily on carbon-heavy fuels, directly correlating negative environmental impacts.
- Recent works highlight cleaner alternatives utilizing oxygen, showcasing successful adoption in various sectors.
This evolution in research perspectives indicates a growing recognition of oxygen's role in achieving ecological balance.
"The integration of oxygen in energy systems is not just a scientific curiosity; it's a necessary step toward sustainable energy practices."
By exploring innovative energy solutions with oxygen, we can better address the contemporary challenges in the energy landscape.
Prelude to Oxygen and Energy
The intersection between oxygen and energy is an intricate subject, woven into the fabric of biochemical processes and energy systems. Understanding this topic is critical due to the diverse applications of oxygen, ranging from biological functions to energy production. With increasing global challenges surrounding energy sources and environmental sustainability, exploring oxygen's role becomes indispensable.
Oxygen energy encompasses the utilization and transformation of oxygen within various energy systems. This includes its biological function in cellular respiration and its application in energy generation through combustion and fuel technology. By grasping these concepts, researchers and professionals can better address current energy challenges and shift towards sustainable practices.
In this segment, we delve into the definition of oxygen energy to establish a clear basis for its relevance and implications across multiple fields.
Definition of Oxygen Energy
Oxygen energy refers to the energy derived from the interactions involving oxygen in various contexts. It plays a fundamental role in cellular respiration, where oxygen acts as a crucial element for converting glucose into adenosine triphosphate (ATP), the principal energy currency of living cells. This energy is vital for numerous biological processes that sustain life.
In broader terms, oxygen energy extends to its applications in industries focused on energy production. Whether through combustion processes that allocate energy from fossil fuels or renewable sources using fuel cells, oxygen stands as a key player. Consequently, understanding the nature of oxygen energy forms a foundational pillar for examining its multiple implications in energy systems, including environmental impacts.
Historical Context of Oxygen Energy Research
The exploration of oxygen energy has deep historical roots, beginning with the discovery of oxygen in the late 18th century. Joseph Priestley and Carl Wilhelm Scheele, independently, contributed significantly to the understanding of this gas. Their findings paved the way for the realization of oxygen's critical role in combustion and respiration.
In the 19th century, scientists like Antoine Lavoisier advanced the comprehension of chemical reactions involving oxygen. Lavoisier's work in identifying the role of oxygen in oxidation processes reframed our understanding of energy release during combustion. Subsequently, the 20th century witnessed an escalating interest in oxygen's applications within power generation and biotechnology.
Today, research continues to evolve, emphasizing the integration of oxygen energy within renewable technologies. This historical context not only offers insight into the scientific progress made but also underscores the ongoing demand for efficient and sustainable energy practices in modern society.
The Biochemical Role of Oxygen
The biochemical role of oxygen is crucial for understanding energy systems. Oxygen is fundamental in various biochemical processes that occur within living organisms. It acts as a key player in the conversion of energy from food into usable forms. By examining the functions of oxygen, we can recognize its importance in cellular processes and metabolism. These insights are essential not only for biological understanding but also for energy applications in technology and sustainability.
Oxygen in Cellular Respiration
Anaerobic vs. Aerobic Processes
Cellular respiration is divided into two main types: anaerobic and aerobic processes. Anaerobic respiration occurs without oxygen, while aerobic respiration uses oxygen to maximize energy extraction from nutrients. A key characteristic of aerobic processes is their efficiency in producing adenosine triphosphate (ATP), the energy currency of cells. This makes aerobic respiration a widely favored choice in energy production discussions.
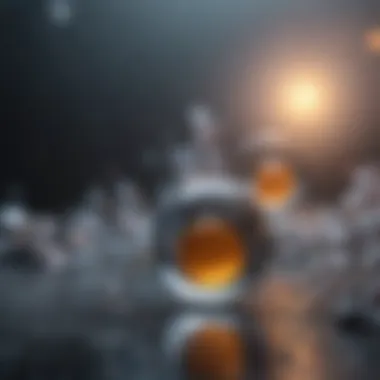
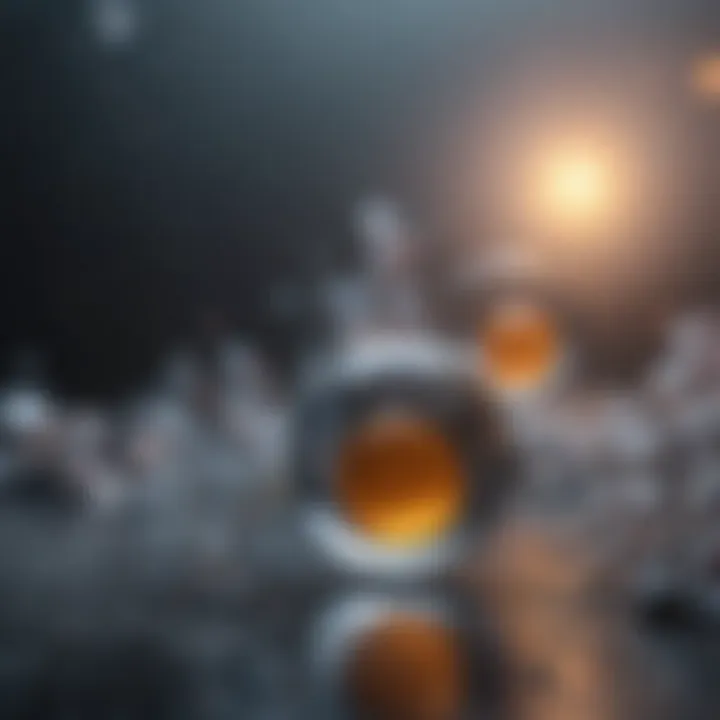
One unique feature of anaerobic respiration is that it allows organisms to thrive in oxygen-depleted environments. While useful, it produces significantly less ATP compared to aerobic respiration. Thus, while anaerobic processes can serve specific ecological niches or conditions, aerobic respiration remains the more effective route for energy efficient utilization.
ATP Production
Adenosine triphosphate production is a critical aspect of energy metabolism. ATP serves as the principal energy carrier in all living cells. The primary feature of ATP production in aerobic respiration is its high yield. This method can yield up to 36 ATP molecules from a single glucose molecule. Consequently, it is a popular topic in discussions related to energy, especially when comparing metabolic pathways.
The unique advantage of ATP production via aerobic processes is its ability to support complex life forms that require substantial energy. However, this method relies on a constant oxygen supply, which can represent a disadvantage under specific environmental conditions.
Oxygen and Metabolism
Energy Conversion
Energy conversion refers to the process of transforming energy from one form to another. In biological systems, it often involves the conversion of food energy into chemical energy stored in ATP. Oxygen plays an integral role in this process, particularly in aerobic metabolism. This metabolic pathway utilizes oxygen to extract greater energy from organic compounds.
The unique feature of energy conversion involving oxygen is its efficiency. It allows living organisms to harness maximum energy potential from their food sources. However, without oxygen, organisms must resort to less efficient methods that produce fewer energy molecules.
Role in Biochemical Pathways
Oxygen's role in biochemical pathways extends beyond merely supporting respiration. It is involved in various metabolic pathways as an essential component. The key characteristic of these pathways is their reliance on oxygen to drive chemical reactions needed for life. For example, oxygen is a crucial element in the citric acid cycle and oxidative phosphorylation.
This unique feature illustrates oxygen's importance as a reactant in numerous cellular functions. However, the dependence on oxygen can limit the ability of some organisms to survive in low-oxygen environments, indicating both advantages in energy efficiency and potential limitations in ecological adaptability.
Understanding the biochemical role of oxygen highlights its dual significance: essential for energy production but also a factor in environmental constraints.
Oxygen in Energy Production
Oxygen plays a pivotal role in various energy production methods. Understanding its function in this context is key to appreciating how energy systems operate. The presence of oxygen is essential in both combustion processes and fuel cells, which are vital for modern energy generation. This section delves into these aspects, revealing how oxygen not only influences energy efficiency but also informs environmental considerations.
Combustion Processes
In energy production, combustion processes are fundamental. They convert stored energy in fuels into usable energy, primarily heat and power. Oxygen's role in combustion is indispensable as it serves as an oxidant, a necessary component for reaction.
Fossil Fuels
Fossil fuels, such as coal, oil, and natural gas, are significant sources of energy worldwide. The key characteristic of fossil fuels is their high energy density, making them a popular choice for energy production. When burned in the presence of oxygen, they release substantial amounts of energy, primarily in the form of heat.
However, fossil fuels come with notable disadvantages. They emit greenhouse gases, particularly carbon dioxide, contributing significantly to climate change. Additionally, they are finite resources, leading to concerns about sustainability and resource depletion.
The reliance on fossil fuels demands a careful evaluation of their environmental impact versus their energy production capabilities.
Biomass
Biomass, on the other hand, involves organic materials such as wood, agricultural crops, and waste. One of the unique features of biomass is that it is renewable; its sources can be replenished over time. Using biomass in energy production also capitalizes on the fact that it releases carbon dioxide during combustion, but this CO2 is offset by the CO2 absorbed by the plants during their growth.
Biomass offers advantages such as lower net carbon emissions and its potential for local energy production, reducing reliance on imported fossil fuels. However, there are also drawbacks. Land must be dedicated to biomass production, which may compete with food production. Additionally, inefficient conversion processes can result in lower energy yields compared to fossil fuels.
Role of Oxygen in Fuel Cells
Fuel cells are another significant avenue for energy production involving oxygen. They convert chemical energy directly into electrical energy through electrochemical reactions. The presence of oxygen is crucial in this setting, as it acts as an electron acceptor.
Types of Fuel Cells
There are several types of fuel cells, each utilizing oxygen in different ways. Protons Exchange Membrane (PEM) fuel cells are known for their efficiency and quick start-up times, making them beneficial for transportation applications. Solid Oxide Fuel Cells (SOFCs), while less efficient, provide high overall efficiency and flexibility in fuel types.
These fuel cells are popular because they produce only water and heat as by-products, presenting fewer environmental concerns. Still, the infrastructure needed for fuel distribution and the cost of materials pose challenges to broader fuel cell adoption.
Efficiency Considerations
Efficiency in fuel cells is a critical aspect that determines their potential adoption. Fuel cells usually achieve high conversion efficiencies compared to traditional combustion systems. The primary characteristics influencing their efficiency include operating temperature, types of fuel used, and design configurations.
Higher efficiency directly correlates with reduced energy waste, but it often comes at a cost. Maintaining high efficiency usually requires advanced materials and sophisticated control systems, which can be economic considerations for large-scale implementations. However, the long-term benefits in emissions reduction and sustainability can justify these costs.
Oxygen and Renewable Energy Sources
The intersection of oxygen and renewable energy sources is a significant theme in contemporary energy discussions. As the focus on sustainability becomes ever more urgent, understanding how oxygen plays a vital role in renewable energy production is crucial. This exploration highlights the mechanisms through which oxygen contributes to sustainable energy solutions, such as hydrogen production, and emphasizes the natural processes like photosynthesis that harness oxygen for energy.
Hydrogen Production via Water Electrolysis
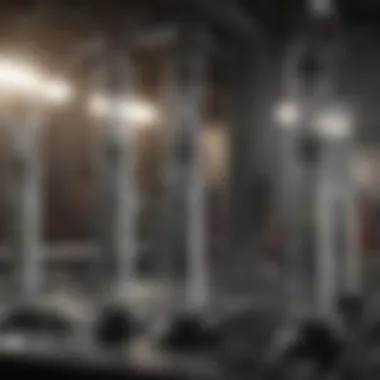
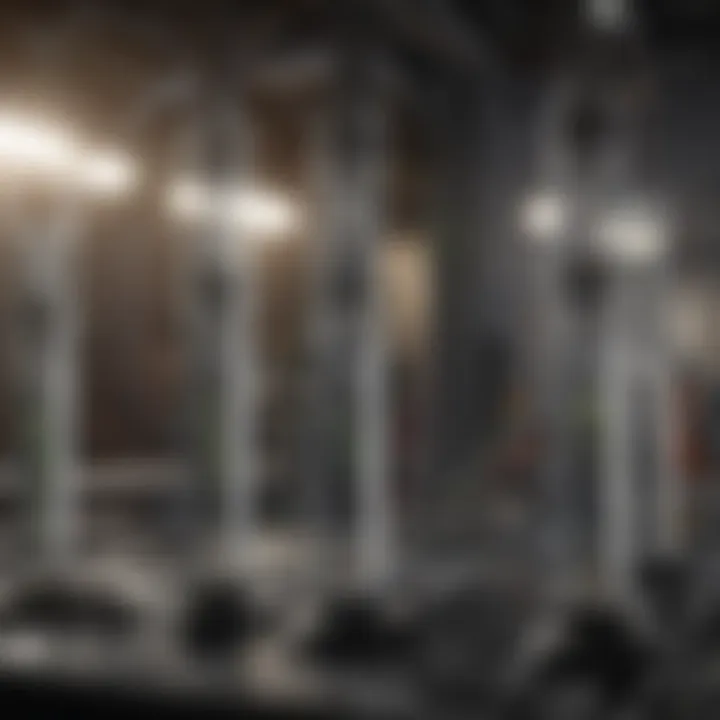
Water electrolysis stands as one of the most promising methods for producing hydrogen. In this process, water (O) is separated into hydrogen () and oxygen (O2) by applying an electric current. The generated hydrogen can be stored and used as a clean fuel source.
The critical aspect of hydrogen production via water electrolysis is that it relies heavily on the availability of a clean source of electricity, ideally derived from renewable sources such as solar or wind. This creates a sustainable cycle where renewable energy is converted into a hydrogen fuel, which itself releases only water when consumed, making it an environmentally friendly choice.
Using electrolysis, the oxygen produced can also serve other purposes, such as in industrial applications or even in improving air quality in urban areas. This dual benefit emphasizes the importance of considering oxygen not just as a supporting element but as a valuable by-product of the hydrogen production process.
Photosynthesis and Its Energy Implications
Photosynthesis is a fundamental biological process that not only sustains plant life but also impacts energy systems significantly. Plants utilize sunlight, carbon dioxide, and water to produce glucose and oxygen. The produced oxygen is then released into the atmosphere.
Mechanism of Photosynthesis
The mechanism of photosynthesis involves two main stages: light-dependent reactions and light-independent reactions (Calvin cycle). During the light-dependent stage, sunlight is harnessed to split water molecules, releasing oxygen as a by-product. This oxygen is crucial as it replenishes the atmospheric supply, underscoring its importance in both ecological and energy contexts.
The key characteristic of photosynthesis is its ability to convert solar energy into a storable chemical form, glucose, which supports the entire food chain. This process is beneficial because it directly ties the production of energy to natural cycles. Moreover, utilizing photosynthesis in bioenergy production leverages the efficiency of nature.
Bioenergy Production
Bioenergy refers to the energy derived from biological materials, primarily plants. The specific aspect of bioenergy production involves converting biomass to usable energy forms, such as ethanol or biogas. This connection to oxygen is evident, as plants capture atmospheric carbon dioxide and release oxygen during their growth.
The unique feature of bioenergy production lies in its renewable nature, contributing to a sustainable energy landscape. By using plants to produce energy, we are tapping into an earth-friendly cycle that minimizes waste and pollutants. The advantage of this approach includes reducing reliance on fossil fuels and achieving lower carbon footprints.
However, there are also challenges to consider. Land use for energy crops can conflict with food production, presenting ethical and logistical dilemmas. Thus, while bioenergy is a viable solution, it necessitates careful management to balance ecosystem health and food security.
Oxygen is not merely a by-product; it is a component of a sustainable ecosystem.
Overall, the synergy between oxygen and renewable energy sources reveals numerous opportunities for innovation in energy production. Continuing to research and harness these relationships offers a pathway to a sustainable energy future.
Implications for Environmental Sustainability
The role of oxygen in environmental sustainability cannot be overstated. In an age where climate change and pollution pose significant threats to ecosystems and public health, understanding oxygenβs potential is crucial. It is key in various processes that contribute to a healthier environment. The implications of oxygen energy span across pollution reduction and climate change mitigation, both critical elements to consider for a sustainable future.
Oxygen's Role in Pollution Reduction
Oxygen directly interacts with pollutants in the atmosphere. When discussing air quality, oxygen plays a vital role in oxidation processes that can help eliminate harmful substances. For instance, oxygen is essential in the breakdown of volatile organic compounds (VOCs). These compounds are often emitted from paints, solvents, and other industrial activities.
In addition, the presence of enough oxygen in the environment can significantly aid in bioremediation. This is a process where microorganisms, fueled by oxygen, degrade pollutants in soil and water. The effectiveness of bioremediation highly depends on an adequate supply of oxygen. More oxygen means that a larger number of microorganisms can thrive, leading to faster and more complete decomposition of harmful substances.
Oxygen also assists in reducing nitrogen oxides and sulfur oxides emissions when used in combustion processes. Optimizing oxygen levels during combustion can decrease the formation of these gases, which are significant contributors to acid rain and respiratory problems. Thus, adopting practices that enhance oxygen availability in various sectors can result in notable pollution control benefits.
Oxygen Energy in Climate Change Mitigation
As climate change becomes an urgent global issue, oxygen energy's role in mitigation efforts is increasingly recognized. While carbon dioxide receives significant attention as a greenhouse gas, the role of oxygen in carbon cycling is often overlooked. In natural processes such as photosynthesis, oxygen is released as a byproduct. This process is essential for capturing carbon dioxide, which helps in regulating atmospheric levels and combating climate change.
Innovations in oxygen energy technologies also show promise. For instance, using oxygen-rich environments in industrial processes can reduce carbon emissions. Enhanced oxygen use in combustion and gasification can lead to cleaner energy generation, lowering greenhouse gas output. Consequently, focusing research on optimizing oxygen utilization can be a pivotal strategy in reducing our carbon footprint.
Furthermore, oxygen plays a crucial role in the development of sustainable fuels. By advancing technologies that utilize oxygen effectively, we can shift towards greener energy sources. Hydrogen production through water electrolysis, for example, also highlights the importance of oxygen. Sustainable hydrogen generation can help lower reliance on fossil fuels, contributing significantly to climate change mitigation.
"The multifaceted role of oxygen in energy systems highlights its potential for addressing pressing environmental challenges strategically."
Current Research Trends in Oxygen Energy
Current research trends in oxygen energy are critical in shaping our understanding and utilization of oxygen in various energy systems. This area is gaining traction due to the increasing need for sustainable energy solutions to combat climate change and the depletion of fossil fuels. Key elements of this research involve innovative technologies, collaboration between institutions, and the development of practices that leverage oxygen's unique properties in energy production.
Innovations in Oxygen-Based Technologies
Emerging Technologies
Emerging technologies are redefining how we harness oxygen for energy uses. One crucial aspect is the advancement of oxygen generation methods. New techniques, such as the use of artificial intelligence to optimize oxygen extraction from air, are quickly becoming popular. This is beneficial for producing clean energy efficiently and cost-effectively.
A key characteristic of these technologies is their ability to integrate with existing energy systems, enhancing their functionality. For example, systems that combine renewable energy with oxygen production can significantly lower carbon footprints. However, there are unique features, such as high initial investment costs and technical complexities, which may pose challenges. The balance between innovation and practicality is essential in evaluating these technologies.
Research Institutions
Research institutions play a fundamental role in advancing the field of oxygen energy. They are at the forefront of researching alternative methods to utilize oxygen in energy systems. Their collaborative efforts drive breakthroughs in understanding oxygenβs biochemical role and its applications in energy generation.
A notable feature of these institutions is their multidisciplinary approach, combining expertise from various fields. This is advantageous as it encourages fostering diverse ideas and strategies to tackle energy-related issues. However, research can occasionally become siloed, focusing too narrowly on specific aspects, which may limit broader applications. Connecting findings across different research areas is crucial to maximize the benefits.
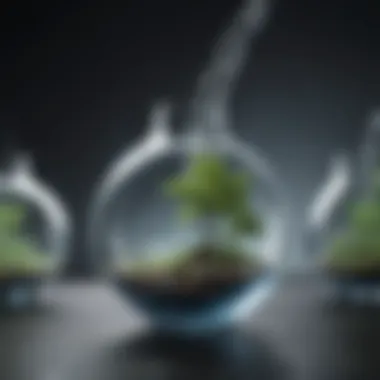
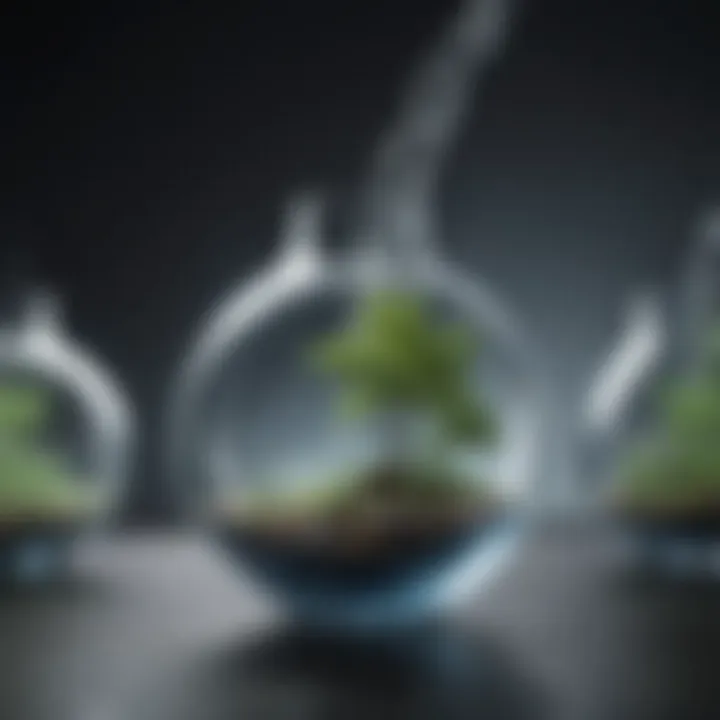
Collaborative Research and Development Efforts
Collaborative research and development efforts are essential in the context of oxygen energy. These efforts often bring together academia, industry, and government bodies. The synergy created through this collaboration enhances the pace of discovery and innovation.
Involving multiple stakeholders allows for pooling of resources and expertise, which can accelerate the development of new technologies. Moreover, partnerships can lead to shared knowledge which optimizes results, allowing researchers to tackle obstacles more effectively.
An example of a successful collaborative effort is the partnership between universities and private companies focusing on improving fuel cell technology. These collaborations help in exchanging ideas and testing new concepts in real-world scenarios.
In addition, funding opportunities associated with collaborative projects can significantly enhance research capabilities. Nevertheless, managing expectations and coordinating between different parties can be challenging, sometimes leading to conflicts or miscommunication.
In summary, understanding the trends in oxygen energy research reveals both opportunities and challenges ahead. Innovations in technologies and collaborative efforts are vital for advancing this field and addressing global energy concerns.
Challenges and Limitations
In any advancements regarding oxygen energy, understanding the challenges and limitations is crucial. This section focuses on the obstacles hindering the broader application of oxygen in energy generation and utilization. The implications of these challenges can significantly affect not only technological progress but also economic and environmental considerations.
Technical Challenges in Oxygen Energy Harnessing
The technical challenges faced in harnessing oxygen energy are multifaceted. One major issue lies in the efficiency of oxygen utilization in various energy systems. For instance, in fuel cells, achieving optimal performance often requires precise control of oxygen levels and pressure. This presents a challenge, as variations in these parameters can lead to decreased output and efficiency.
Another challenge involves the materials used in energy systems. Certain materials may react adversely with oxygen, leading to degradation over time. This necessitates ongoing research into more resilient materials that can withstand the high oxidative environments typical in energy systems. Additionally, creating systems that can effectively separate and purify oxygen from less desirable gases poses another hurdle.
"Technical innovations and robust materials are necessary to eliminate barriers in oxygen energy systems."
Furthermore, the integration of oxygen energy systems into existing infrastructures can often require significant adaptations. This can include costly modifications to current energy facilities or the development of new systems that are compatible with oxygen-based energy production.
Economic Considerations
The economic aspects of oxygen energy are significant when assessing its future viability. The initial investment for developing oxygen energy technologies can be substantial. Companies and research institutions must weigh these costs against potential returns. For large-scale implementation, the economic models must also consider long-term operational expenses.
Moreover, the market for oxygen energy solutions is still evolving. This leads to uncertainties regarding pricing and competition with established energy sources such as fossil fuels. Without regulatory frameworks or incentives favoring oxygen energy advancements, it can be difficult to justify investments.
Unexpected fluctuations in raw material costs also pose risks. If the price of necessary inputs, such as hydrogen for fuel cells, surges, this increases operational expenses, making it hard to maintain competitive pricing.
Finally, public perception and acceptance play significant roles. If the benefits of oxygen energy are not effectively communicated, it may hinder potential market growth. Therefore, stakeholders must engage in clear communication about the advantages of oxygen energy to foster a supportive environment for its expansion.
Future Perspectives
The future of oxygen energy is gaining considerable attention in both scientific and policy-making circles. As the world increasingly confronts challenges such as climate change, energy depletion, and pollution, the role of oxygen in various energy systems is more pertinent than ever. This section explores potential developments in oxygen energy research and examines its implications for global energy policies.
Potential Developments in Oxygen Energy Research
Research in the field of oxygen energy is on the brink of transformative breakthroughs. Several avenues present promising opportunities:
- Advancements in Bioenergy: Research into biofuels produced from algae or agricultural waste that utilize oxygen efficiently is critical. These methods can potentially yield high energy outputs with lower greenhouse gas emissions.
- Enhanced Fuel Cell Technology: Innovations in the design and operation of oxygen-fueled cells may significantly improve efficiency and lifespan. This evolution can facilitate broader adoption in both vehicles and stationary power generation.
- Water Splitting for Hydrogen Production: Exploring more effective methods for electrolysis can lead to more sustainable hydrogen production using oxygen. This hydrogen can serve as a potent energy carrier in numerous applications.
Addressing technical challenges will be crucial. This may include material sciences dealing with electrode longevity and optimizations in catalyst performance. Direct investment in interdisciplinary research collaborations can amplify these developments, bringing together chemists, engineers, and environmental scientists.
Impact on Global Energy Policies
The implications of advances in oxygen energy will extend to global energy policies as well. As nations pivot towards sustainability, there are several considerations for policymakers:
- Renewable Energy Incentives: Countries may need to implement subsidizing programs that encourage the use of technologies utilizing oxygen in energy systems, especially within the economic framework of renewable resources.
- Legislative Frameworks: Policymakers can advocate for regulations that promote research and development in oxygen energy, ensuring that academic and industrial sectors can collaborate effectively.
- Global Cooperation: Sharing of knowledge and technologies across borders can lead to accelerated advancements in oxygen energy. Collaborative international research initiatives can enhance understanding and applications of oxygen in energy generation.
"As oxygen energy research progresses, it could redefine energy strategies worldwide, providing cleaner and more efficient methods for power generation that meet the demands of the 21st century."
Culmination
The conclusion of this article synthesizes various aspects of oxygen energy, highlighting its significance across diverse fields. In doing so, it reflects on the crucial role oxygen plays not only in our ecological systems but also in energy production and sustainability practices. As one delves into the implications and applications of oxygen energy, several key elements emerge that warrant attention.
Summarizing Key Insights
The multifaceted nature of oxygen energy encompasses various areas of study and application. Key insights include the following:
- Biochemical Significance: Oxygen is essential for cellular respiration, fundamental to life. Its role in ATP production illustrates its importance in energy metabolism.
- Energy Production Mechanisms: Oxygen fuels combustion processes, necessary for harnessing energy from fossil fuels and biomass. Moreover, its involvement in fuel cells highlights its versatility in alternative energy sources.
- Sustainability: Oxygenβs role extends to environmental health, with implications for pollution reduction and climate change mitigation. By promoting renewable resources, the pursuit of oxygen energy can align with ecological balance, ensuring a sustainable future.
- Continuous Research Trends: The ongoing advancements in oxygen-based technologies are pivotal for innovative energy solutions. Collaborative research efforts are crucial for addressing the challenges faced in this domain.
Despite these insights, ongoing challenges must also be acknowledged, particularly in harnessing oxygen energy efficiently within the economic landscape.
Call for Continued Research
Continued research in the field of oxygen energy is not merely beneficial; it is necessary. The pursuit of sustainable energy solutions requires an exploration of innovative techniques and technologies. Understanding the biochemical processes surrounding oxygen can lead to breakthroughs in energy efficiency and production.
Further, researchers and developed institutions should foster collaboration for optimal results. Addressing the technical difficulties in harnessing oxygen energy needs a cooperative approach, involving diverse expertise. Expanded funding and resource allocation are critical elements for advancing this important work.
Research in this field could uncover valuable information on integrating oxygen energy within existing energy infrastructures. The implication of this could greatly enhance energy policies worldwide, paving the way for long-term sustainability in energy production.