Understanding Mass Spectroscopy Data: Insights and Applications
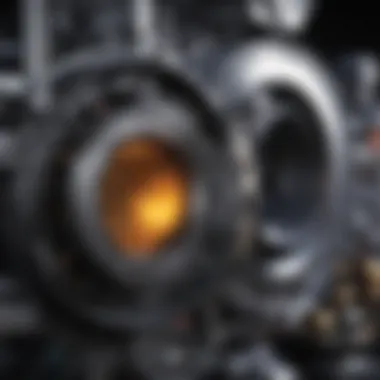
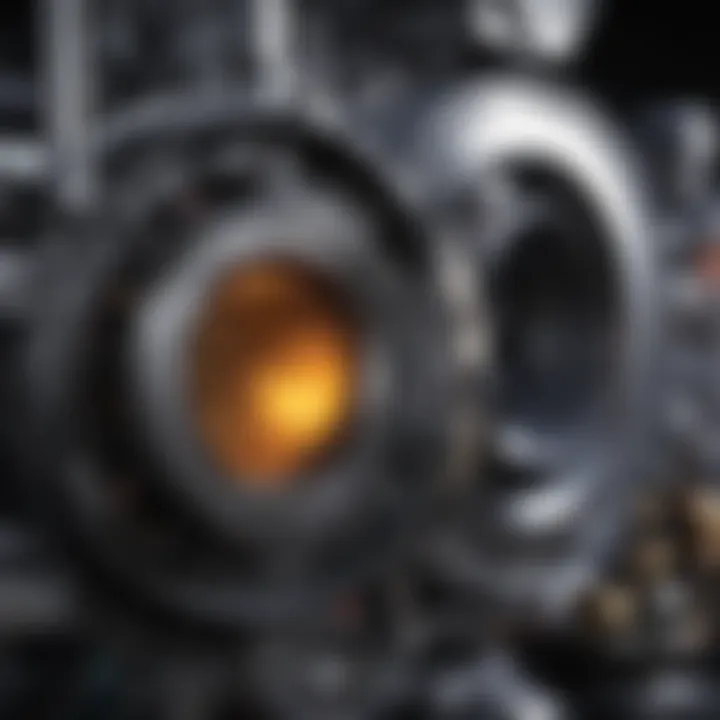
Intro
Mass spectroscopy is an analytical technique that has gained significant importance in various scientific fields. Its capability to provide precise measurements of molecular weights and understand complex mixtures makes it an invaluable tool in modern research. The essence of mass spectroscopy lies in its ability to analyze the mass-to-charge ratio of ions. Such analyses have profound implications in areas like chemistry, biology, and environmental science.
This article aims to dissect the intricacies of mass spectroscopy data, illuminating its principles, technology, and applications. We will explore how scientists generate and interpret mass spectral data, and the challenges that arise during data acquisition and analysis. By doing so, we hope to foster a deeper understanding of this essential tool, enhancing its utility among students, researchers, educators, and professionals.
Research Overview
Summary of Key Findings
In the realm of mass spectroscopy, data interpretation is paramount. The results obtained can reveal critical insights about molecular structures, allowing for elucidation of chemical reactions and biological pathways. Key findings often highlight:
- The ability to identify unknown compounds with high sensitivity.
- The role of mass spec data in proteomics and metabolomics research.
- Challenges in data calibration affecting reproducibility and accuracy.
Such findings emphasize the necessity to comprehend and refine the techniques applied in data analysis.
Methodologies Employed
Different methodologies are applied in extracting and interpreting mass spectrometry data. Among these, methodologies have advanced over time:
- Sample Preparation: The first step that determines the quality of data, involving purification and sometimes, derivatization.
- Ionization Techniques: Methods like Electrospray Ionization (ESI) and Matrix-Assisted Laser Desorption/Ionization (MALDI) are crucial for generating ions that are detected.
- Mass Analysis: Using Time-of-Flight (TOF) or Quadrupole Analyzers helps in determining m/z values accurately.
These methodologies are fundamental to ensuring that mass spectroscopy results are both reliable and replicable.
In-Depth Analysis
Detailed Examination of Results
Once mass spectra are obtained, a thorough examination is necessary. This involves analyzing peak intensities and their corresponding m/z values. High-resolution mass spectrometry can resolve differences in isotopic patterns, enabling the identification of elemental compositions. The interpretation of such data frequently requires specialized software designed to share insights from complex figures.
Comparison with Previous Studies
Historical context is important when considering mass spectroscopy data. Comparing recent findings with prior studies often uncovers trends or discrepancies that merit further investigation. A review of the literature might show:
- Advances in sensitivity that have enabled detection of trace levels of substances.
- Innovations in automation that have improved data acquisition efficiency.
Such comparisons not only validate current research but also highlight areas for future exploration.
Preface to Mass Spectroscopy
Mass spectroscopy stands as a cornerstone in modern analytical chemistry. The ability to precisely analyze the composition of substances translates to invaluable insights across various scientific avenues. Understanding this technique begins with recognizing its fundamental role in elucidating molecular structures, determining molecular weights, and identifying chemical compounds in complex mixtures. This field of study empowers researchers to investigate substances from environmental samples to biochemical substances. In addressing the intricacies of mass spectroscopy, one lays the foundation for its applications and pertinence across disciplines.
Definition and Importance
Mass spectroscopy is a technique used to measure the mass-to-charge ratio of ions. It involves the ionization of chemical compounds to generate charged molecules or molecule fragments, which are then detected and analyzed. This method offers insights into the molecular architecture of compounds, helping to identify unknown substances, quantifying known materials, and elucidating complex molecular characteristics. Mass spectroscopy is crucial for drug development, proteomics, environmental monitoring, and many other areas where understanding complex mixtures is vital. The accuracy and sensitivity of mass spectroscopy provide a beneficial edge in research and application, highlighting its eminent importance in scientific discovery.
Historical Development
The journey of mass spectroscopy has evolved significantly since its inception in the early 20th century. The first mass spectrometer was constructed by J.J. Thomson in the late 1910s. This device laid the groundwork for future innovations, activating curiosity in the analysis of atomic structures. Over the decades, advancements such as the development of the first magnetic sector mass spectrometer in the 1940s expanded the capacity to analyze more complex samples.
The 1980s ushered in a new era with the introduction of soft ionization techniques like Electrospray Ionization (ESI) and Matrix-Assisted Laser Desorption/Ionization (MALDI). These advances minimized fragmentation, allowing for more intact molecules to be analyzed. Such historical progress is not merely an account of inventions; it reflects a deeper comprehension of matter on a molecular level, impacting numerous scientific disciplines and facilitating groundbreaking research efforts.
Principles of Mass Spectrometry
Mass spectrometry is a pivotal analytical technique that offers insights into the composition and structure of chemical and biological molecules. Understanding the principles of mass spectrometry is essential for any researcher or professional working in related fields. This section emphasizes the fundamental components involved in mass spectrometry, which include ion generation, ion analysis, and detection methods. Each element plays a crucial role in determining the quality and accuracy of the spectral data obtained.
Ion Generation
Ion generation is the first step in mass spectrometry, transforming neutral molecules into ions. This process is essential because mass spectrometers analyze ions based on their mass-to-charge ratio. There are various methods for ion generation, such as Electron Ionization (EI), which uses electrons to ionize molecules, or Electrospray Ionization (ESI), which allows for the ionization of larger biomolecules.
The effectiveness of ion generation techniques can impact the results of the analysis. For instance, ESI is particularly advantageous for biological samples, as it produces ions in solution without causing extensive fragmentation. This ability to maintain the integrity of larger molecules makes it suitable for analyzing proteins, peptides, and nucleic acids.
Key points regarding ion generation include:
- The method selected influences the types of ions produced.
- Proper selection helps in obtaining high ionization efficiency.
- Different methods can lead to varied structural information about the analyte.
Ion Analysis
Once ions are generated, they are directed into the mass analyzer where they are sorted based on their mass-to-charge ratios. This part of the mass spectrometry process is vital for determining the exact composition of a sample. The predominant methods for ion analysis include Quadrupole, Time-of-Flight (ToF), and Ion Trap analyzers. Each method has distinct benefits and limitations, impacting resolution, speed, and sensitivity.
In Quadrupole mass spectrometers, ions pass through an electric field, with only specific mass-to-charge ratios reaching the detector. This results in high sensitivity and allows for quantitative analysis. Time-of-Flight analyzers, on the other hand, measure the time it takes for ions to travel a fixed distance. This method can provide high-resolution data and is suitable for a wide variety of sample types.
Considerations in ion analysis include:
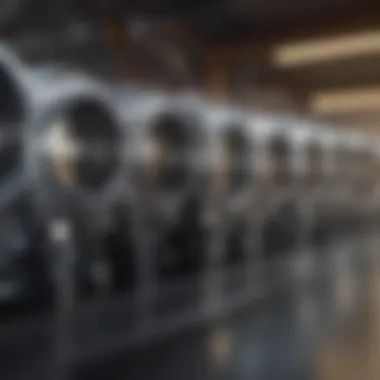
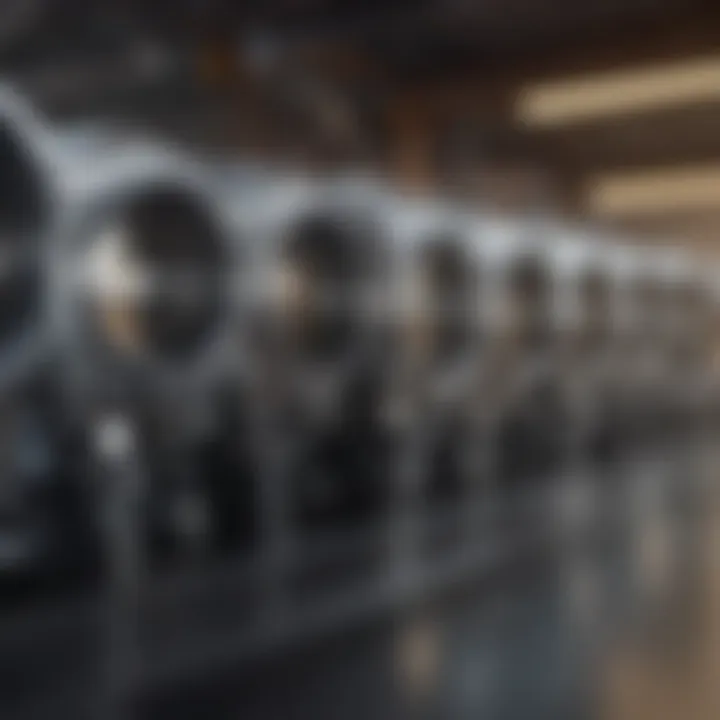
- Sensitivity is key for low-abundance samples.
- Resolution determines the ability to distinguish between closely related compounds.
- Speed can be crucial for high-throughput applications.
Detection Methods
The final phase of mass spectrometry involves detecting the ions, converting their presence into measurable signals. Various detection methods can be employed, with the most common being the Electron Multiplier and Faraday Cup detectors. The choice of detection method can greatly affect data accuracy and reliability.
Electron Multiplier detectors amplify ion signals, allowing for the detection of even minute quantities of ions. This sensitivity makes them suitable for diverse applications, including trace analysis in complex matrices. Faraday Cup detectors, meanwhile, directly measure the charge of ions, offering linear response in quantification.
Effective detection methods ensure:
- High sensitivity for analyzing trace components.
- Accuracy in quantifying ion counts.
- Reliability in the face of complex sample matrices.
In summary, the principles of mass spectrometryβion generation, ion analysis, and detection methodsβare interdependent layers that contribute to the overall efficacy of the technique. Mastery of these principles allows researchers to obtain meaningful data from their samples, paving the way for groundbreaking discoveries.
Types of Mass Spectrometers
The realm of mass spectrometry is vast and varied, with different types of mass spectrometers designed for specific analytical needs. Understanding each type provides insights into their distinct applications and functionalities. The importance of exploring the types of mass spectrometers lies in their unique mechanisms, which influence how mass spectrometry data is gathered and interpreted. If you are a student, researcher, or professional in the field, knowledge of these instruments and their strengths is crucial.
Quadrupole Mass Spectrometer
The Quadrupole Mass Spectrometer is one of the most widely used instruments in mass spectrometry. It operates on the principle of electric and magnetic fields to filter ions based on their mass-to-charge ratios. This technology allows isolating specific ions for further analysis.
Key Features:
- Simple design and operation.
- Fast data acquisition.
- Multi-stage capabilities leading to tandem mass spectrometry.
Benefits and Considerations:
The quadrupoleβs speed makes it a top choice for routine analysis. However, it has limitations regarding resolution and sensitivity when compared to other types of mass spectrometers.
Time-of-Flight Mass Spectrometer
The Time-of-Flight (TOF) Mass Spectrometer stands out due to its ability to measure the time ions take to travel a specific distance. By applying a high voltage to the ions, their time to reach the detector provides a direct indication of their mass.
Key Features:
- High mass resolution.
- The capability to analyze large molecules.
- Excellent for fast analysis of complex mixtures.
Benefits and Considerations:
The TOF mass spectrometer excels in speed and sensitivity, allowing for the assessment of low-abundance species. However, it requires precise calibration to maintain accuracy.
Ion Trap Mass Spectrometer
The Ion Trap Mass Spectrometer utilizes an electric field to collect ions in a confined space. This instrument enables multiple stages of mass analysis, which is particularly useful for tandem mass spectrometry applications.
Key Features:
- Capable of trapping ions for extended periods.
- High sensitivity and mass resolution.
Benefits and Considerations:
While the ion trap provides excellent abilities for complex mixtures, its relatively slower scan speeds can be a hindrance in certain applications.
Orbitrap Mass Spectrometer
The Orbitrap Mass Spectrometer is modern and computationally advanced. It works on principles of ion trapping within an electrostatic field, allowing for highly accurate measurements of ion masses.
Key Features:
- Ultra-high resolution and accuracy.
- Capability to perform complex analyses.
Benefits and Considerations:
Orbitrap spectrometers have become indispensable in proteomics and metabolomics due to their resolution. However, they can be costly and require specialized training to operate efficiently.
Through understanding the types of mass spectrometers, one can select the most appropriate technology for specific analytical challenges.
Mass Spectroscopy Data Acquisition
The process of acquiring data in mass spectroscopy is essential for generating accurate and reliable results. It involves several key practices that impact the quality of the data produced. Each step, from sample preparation through to calibration, plays a pivotal role in determining the validity of the analysis.
Sample Preparation
Sample preparation is the first step in ensuring successful mass spectroscopy data acquisition. This step involves processes such as dilution, extraction, and filtration. Each preparation technique must be tailored to the sample's nature and the analytical question posed. Proper sample preparation minimizes contamination and enhances the representativeness of the results. For example, liquid chromatography often precedes mass analysis to separate components of interest from potential interferences within a sample matrix.
Benefits of careful sample preparation include:
- Enhanced sensitivity and specificity.
- Improved peak resolution in mass spectra.
- Reduction of matrix effects that can skew results.
Data Collection Techniques
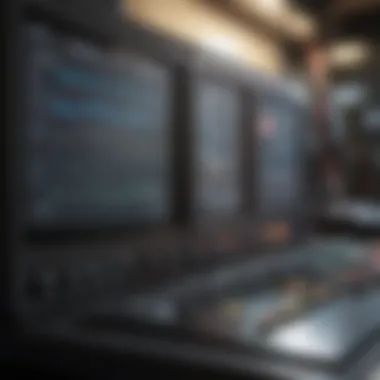
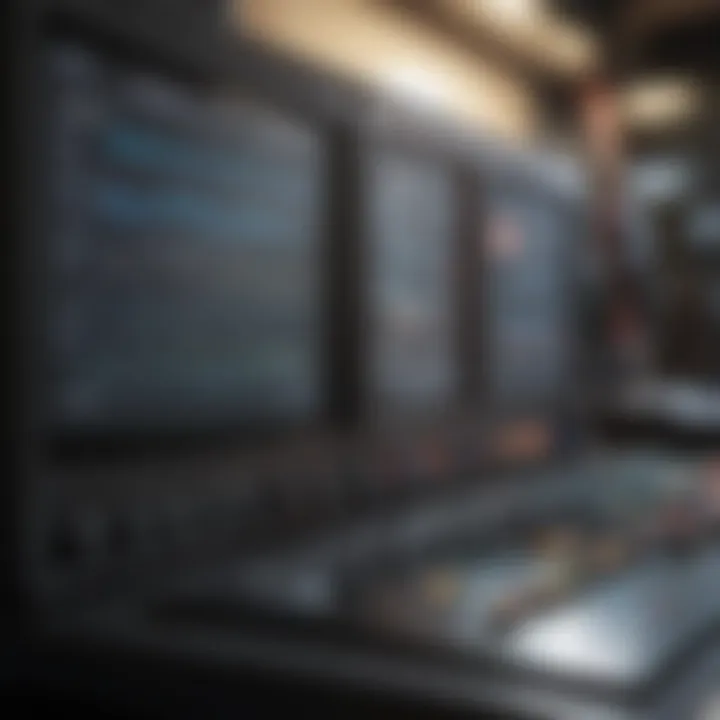
The collection of data in mass spectroscopy must be systematic and precise to ensure reproducibility. Various techniques are employed during this phase, including:
- Continuous flow analysis: Monitors changes in a sample over time.
- Batch analysis: Assesses multiple samples for comparative purposes.
- Single-shot analysis: Allows for immediate results but may sacrifice replication.
Each technique has its merits and should align with the research goals. High-resolution mass spectrometry data can inform detailed structural elucidation. It's crucial for researchers to select a method that complements the type of mass spectrometer used, balancing speed and accuracy based on the experimental demands.
Calibration of Instrumentation
Calibration of the mass spectrometer is the final, yet fundamental, element of data acquisition. It adjusts the mass scale to improve accuracy in mass measurement. Calibration should be performed regularly, using known standards such as phosphoric acid or sodium nitrate, to ensure the instrument delivers precise readings.
Calibrating the instrument involves:\n- Setting up a reference mass.
- Running standard samples at various concentrations.
- Analyzing response curves and adjusting settings for optimal performance.
Accurate calibration allows for more trustworthy analysis, enabling researchers to compare results across experiments -- crucial for drawing valid conclusions in scientific inquiry. As highlighted by experts, "A calibrated instrument transforms complex mixtures into interpretable data," illustrating its direct impact on research outcomes.
Data Interpretation and Analysis
Data interpretation and analysis are pivotal to maximizing the value derived from mass spectroscopy data. The information obtained from mass spectra can provide insights into molecular structure, composition, and dynamics. Understanding how to analyze this data is not merely an academic exercise but is crucial for practical applications in research, drug development, and environmental monitoring.
Chromatographic Separation
Chromatographic separation is often employed before mass spectrometry to purify analytes from complex samples. This process enhances sensitivity and specificity, allowing for more accurate data interpretation. The technique involves separating components in a mixture based on their interactions with stationary and mobile phases. Those separated components are then introduced into the mass spectrometer.
Key benefits of chromatographic separation include:
- Improved accuracy by reducing sample interference.
- Enhanced sensitivity, especially for trace-level analysis.
- Ability to analyze complex mixtures comprehensively.
The integration of chromatographic strategies, such as high-performance liquid chromatography (HPLC) or gas chromatography (GC), with mass spectrometry is foundational in obtaining reliable and interpretable results.
Mass Spectrum Interpretation
Interpreting mass spectra is a critical skill that demands a thorough understanding of the underlying principles of mass spectrometry. A mass spectrum displays the relative abundance of ions as a function of their mass-to-charge ratio (m/z). Each peak in the spectrum corresponds to an ion, which provides essential information about the molecule.
Important considerations during spectrum interpretation include:
- Identifying the molecular ion peak: This peak often represents the entire molecule and provides the molecular weight.
- Fragmentation patterns: Understanding how molecules break apart can help deduce structural features and potentially identify compounds.
- Isotope patterns: The presence of isotopes can provide insights into the elemental composition.
Careful analysis of these aspects can lead to robust conclusions regarding the analytes. Analysts must be cautious to avoid misinterpretation, which can arise from overlapping peaks or ion suppression effects.
Comparative Analysis Techniques
Comparative analysis techniques involve using mass spectroscopy data to assess differences and similarities among samples or conditions. These techniques can be especially valuable in clinical research, proteomics, and metabolomics. Methods such as differential analysis can shed light on significant changes in sample composition or concentration.
Common approaches in comparative analysis techniques include:
- Matching spectra: Comparing the resulting spectra from analyzed samples can highlight variations that indicate underlying biological changes.
- Statistical analysis: Applying statistical tools to assess data significance, which can correlate with biological or chemical implications.
- Multivariate analysis: Techniques like Principal Component Analysis (PCA) can help discover patterns and relationships in complex datasets.
Comparative analysis enriches our understanding of samples by placing the data within a broader context, essential for deriving meaningful research conclusions.
"Sampling and accurate analysis reveal the hidden aspects within complex biological systems, allowing targeted insights."
Applications of Mass Spectroscopy
Mass spectroscopy is a versatile analytical technique that finds extensive applications across various scientific disciplines. Understanding its diverse applications is essential to appreciate how mass spectroscopy catalyzes advancements in research and industrial processes. This section will detail its significance, focusing on the specific areas where mass spectroscopy is repeatedly utilized.
In Chemistry
In the field of chemistry, mass spectroscopy serves as a critical tool for identifying and characterizing chemical substances. It allows chemists to determine molecular weights and structural information about small molecules, polymers, and complex mixtures. This technique is especially valuable in organic chemistry, where accurate molecular characterization plays a key role in synthesis and analysis.
- Molecular Fragmentation - Mass spectrometry enables the study of fragmentation patterns, which help in deducing structures and functional groups of unknown compounds.
- Quantitative Analysis - It is employed for measuring concentrations of specific compounds in various matrices, which is vital for reaction monitoring.
Furthermore, the combination of mass spectroscopy with techniques such as gas chromatography and liquid chromatography enhances its capabilities, making it a crucial component of analytical laboratories.
In Biological Research
Mass spectroscopy is essential in biological research due to its ability to analyze biomolecules like proteins, nucleic acids, and lipids. Researchers utilize this technique for several purposes, including biomarker discovery, protein identification, and metabolic analysis.
- Proteomics - It allows for detailed protein profiling, revealing insights into biological pathways and functions. Tools like Matrix-Assisted Laser Desorption/Ionization (MALDI) play a significant role here.
- Metabolomics - Mass spectrometry is critical for studying metabolic profiles, helping understand disease mechanisms and biological responses.
The ability to analyze samples with minute quantities further underscores its importance in the biological sciences.
In Environmental Science

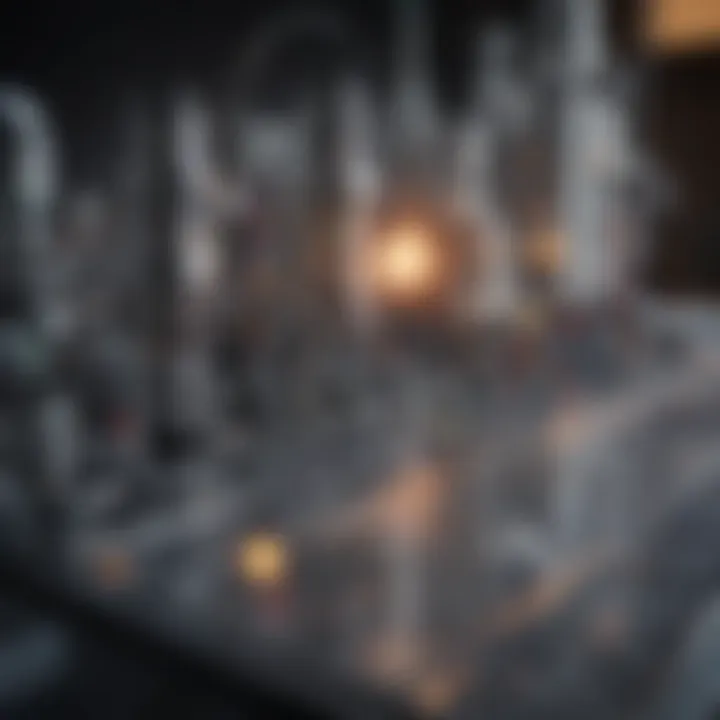
Environmental scientists apply mass spectroscopy to monitor pollutants and understand environmental processes. Its precision allows for analyzing trace levels of contaminants in various environmental matrices like soil, water, and air.
- Pollutants Detection - Mass spectrometry can identify organic compounds and heavy metals, providing insights into pollution sources and impacts.
- Ecotoxicology Studies - Research on the effects of pollutants on ecosystem health relies heavily on mass spectroscopy for accurate quantification of harmful substances.
The technique offers the sensitivity required to evaluate complex environmental samples effectively, aiding in policy-making and regulatory compliance.
In Pharmaceutical Development
In the pharmaceutical industry, mass spectroscopy is pivotal for drug discovery, development, and quality control. It assists in various phases, from initial compound screening to final product release.
- Drug Characterization - Mass spectrometry helps determine the pharmacokinetic properties of new drugs, guiding formulation development.
- Quality Assurance - Consistency and purity testing during manufacturing are enhanced through mass spectroscopy, ensuring products meet stringent safety standards.
Moreover, the increasing integration of mass spectroscopy with information technology facilitates data sharing and improves decision-making processes in drug development.
Mass spectroscopy is not just a technique; it is a pathway to understanding the molecular world, connecting diverse fields and fostering innovation across disciplines.
Challenges in Mass Spectroscopy Data
Mass spectroscopy serves as a critical analytical tool across various scientific fields. However, certain challenges persist that affect the effective utilization of mass spectroscopy data. Addressing these challenges is vital for researchers and practitioners aiming to fully leverage the capabilities of this sophisticated technology. Key challenges include data quality and integrity, instrument limitations, and the skill requirement for precise analysis. Each of these elements plays a significant role in the overall reliability of mass spectrometry findings.
Data Quality and Integrity
Data quality is crucial in mass spectroscopy as it directly influences the accuracy of results. Poor quality data can lead to erroneous conclusions, impacting subsequent research and applications. Factors that can degrade data quality include contamination during sample preparation, calibration errors, or inadequate storage conditions.
To ensure high-quality data, it is essential to implement rigorous protocols throughout the mass spectroscopy workflow. This includes meticulous sample handling, routine calibration of instruments, and using appropriate controls. Additionally, employing data integrity checks can help identify anomalies early on. Consistent monitoring and validation processes can significantly enhance data reliability.
Instrument Limitations
While mass spectrometers have advanced considerably, they come with inherent limitations. Each type of mass spectrometer has a unique set of capabilities and constraints. For instance, a Quadrupole Mass Spectrometer may offer fast analysis times but is limited in detecting certain low-abundance ions. Similarly, while Time-of-Flight Mass Spectrometers provide high-resolution data, they may require intensive data processing efforts.
Understanding these limitations is essential for researchers in selecting the appropriate instrument for their specific applications. Consideration of the sample type, expected ion masses, and the complexity of the matrix can guide instrument selection. Furthermore, ongoing training in instrument operation is important to maximize their potential and minimize errors during analysis.
Skill Requirements for Analysis
The complexity of mass spectroscopy data analysis necessitates a certain skill set for accurate interpretation. Analysts must possess a robust understanding of both mass spectrometry principles and the specific software used for data interpretation. Misinterpretation can lead to significant issues, including false positives or negatives in results.
Continuous education and training are critical for professionals working with mass spectroscopy. Engaging with learning resources, such as workshops or seminars, can help analysts stay updated on best practices. Additionally, collaboration with experienced colleagues can improve analysis skills. Establishing a supportive environment for skill development can enhance both individual and team outcomes in data interpretation.
By adhering to stringent standards and fostering a culture of continuous learning, researchers can overcome these hurdles, leading to more reliable and impactful scientific discoveries.
Future Directions in Mass Spectroscopy
The field of mass spectroscopy continues to evolve, driven by advances in technology and increasing demands from various research disciplines. Understanding future directions in mass spectroscopy is crucial for researchers and professionals. This knowledge allows them to anticipate changes, embrace new techniques, and apply cutting-edge methods to their work. Having insight into what lies ahead helps to enhance the analytical capabilities of laboratories and facilitates research breakthroughs in diverse fields.
Technological Advancements
In recent years, technological innovations have transformed mass spectroscopy. For example, developments in digital signal processing have significantly improved data analysis speed and accuracy. Software solutions that analyze mass spectra can now identify compounds in complex mixtures more efficiently. Moreover, advancements in miniaturization of instruments enable portable mass spectrometers, enhancing fieldwork and real-time analysis capabilities.
Furthermore, the integration of artificial intelligence (AI) into mass spectroscopy adds a new dimension. Machine learning algorithms can be trained to recognize patterns within large datasets. This not only increases the speed of data interpretation but also enhances the reliability of results. Such tools can assist researchers in making more informed decisions based on the data produced from mass spectrometers.
The rise of high-resolution mass spectrometry is another critical advancement. High-resolution instruments can provide detailed information about the molecular mass and structure of compounds. This level of detail is fundamental for studies in proteomics and metabolomics, where intricate biochemical pathways need exploration.
Integration with Other Analytical Techniques
The integration of mass spectroscopy with other analytical techniques is moving to the forefront of future research. By combining mass spectroscopy with techniques like Nuclear Magnetic Resonance (NMR) spectrometry or chromatography, researchers can gain comprehensive insights into complex samples. Such hybrid approaches can enhance the sensitivity and specificity of analyses.
For instance, when paired with Gas Chromatography (GC), mass spectrometry (GC-MS) allows for the separation and identification of volatile organic compounds in mixtures. This has vast applications in forensic science and environmental monitoring.
Combining mass spectroscopy with liquid chromatography (LC) produces a very powerful tool for analyzing biological samples. LC-MS is widely used in pharmacological research to evaluate drug metabolism, providing valuable data on drug efficacy and safety.
The combination of mass spectroscopy with other techniques is leading to better data resolution and more robust results across various scientific fields.
As scientific challenges evolve, so too must mass spectroscopy methodologies. The future will likely see more adaptable and multifunctional analytical systems that provide deeper insights, promoting scientific advancement through collaboration across disciplines.
Culmination
The conclusion serves as a pivotal section of this article on mass spectroscopy data. It encapsulates the main themes discussed and reinforces the overall significance of mass spectroscopy in scientific exploration. A well-structured conclusion not only summarizes the key points but also reflects on broader implications for various fields of inquiry, emphasizing the ongoing relevance of mass spectroscopy as an analytical tool.
Summary of Key Points
In synthesizing the material presented throughout the article, several essential points emerge:
- Fundamental Role: Mass spectroscopy is invaluable in both qualitative and quantitative analysis across numerous scientific disciplines.
- Technological Evolution: Advancements in mass spectrometry technology continue to enhance data acquisition and analysis capabilities.
- Interdisciplinary Applications: The versatility of mass spectroscopy allows its application in chemistry, biology, environmental science, and pharmaceutical development.
- Data Integrity Challenges: Adherence to strict data quality and integrity standards is necessary to ensure reliable results.
- Future Potential: Ongoing research and technological innovations promise exciting developments in mass spectroscopy.
Implications for Research
- Enhanced Research Accuracy: Improved techniques for data calibration and analysis bolster the reliability of research findings.
- Informed Decision-Making: Researchers can leverage mass spectroscopy to make data-driven decisions in their studies, leading to more robust conclusions.
- Cross-Disciplinary Collaboration: Knowledge transfer between disciplines can foster innovative applications of mass spectroscopy data.
- Development of Best Practices: Establishing standardized protocols for data acquisition and interpretation can elevate research quality across the board.
Overall, this article underscores mass spectroscopy's role as an essential analytical technology, influencing not only existing research paradigms but also shaping future innovations in scientific study. Its capacity to provide insightful data across various domains makes it a cornerstone of modern analytical methodology.
The future of mass spectroscopy is not just about technology; it's about redefining how we understand complex biological systems, chemical substances, and environmental interactions.