Understanding Hydrogen Plants: A Comprehensive Exploration
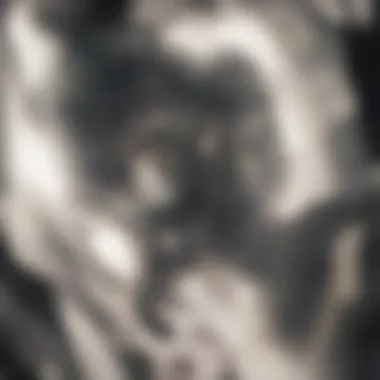
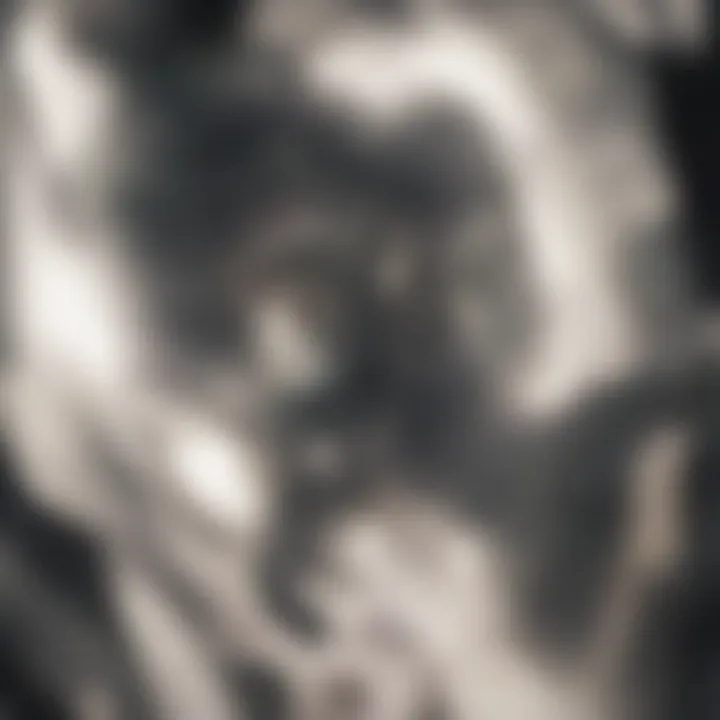
Intro
Hydrogen plants represent a critical component of the evolving energy sector. Their role extends beyond mere production of hydrogen; they are pivotal in addressing global energy challenges. Sustainable energy solutions are increasingly demanded, and hydrogen, with its unique properties, is often seen as a frontrunner in the race toward cleaner energy alternatives.
Understanding how these plants function requires an exploration of their design, operational principles, and significance in the contemporary energy landscape. This article aims to dissect these elements, providing a comprehensive foundation for students, educators, and professionals interested in hydrogen technology.
Advancements in technology have transformed hydrogen production methods, impacting economic viability and environmental sustainability. As the world shifts towards reducing carbon footprints, the relevance of hydrogen plants only intensifies. Therefore, it is imperative to grasp not just the principles behind hydrogen production, but also the various tools and techniques that facilitate efficiency in these plants.
This discussion does not avoid the challenges associated with hydrogen production, including its environmental impacts. Understanding these implications is crucial for implementing effective strategies that enhance the benefits of hydrogen energy while minimizing potential drawbacks.
In the following sections, we will delve into a structured exploration of hydrogen plants, dissect the methodologies employed, and critically analyze current findings in this dynamic field.
Foreword to Hydrogen Plants
Hydrogen plants represent a vital component in the evolving energy landscape. Understanding their role and functionality is essential for students, researchers, and professionals. As the world shifts towards sustainable energy solutions, hydrogen production technologies gain significant attention. The advantages of hydrogen as a clean fuel source align with global efforts to reduce carbon emissions and combat climate change.
What exactly are hydrogen plants? They are facilities designed to produce hydrogen, which can later be utilized in various applications including energy storage, fuel cell electric vehicles, and industrial processes. Their importance lies in addressing energy demands while reducing environmental impacts. Understanding the mechanisms of hydrogen production and the underlying technology can pave the way for future innovation and sustainability.
Definition of Hydrogen Plants
Hydrogen plants are structured systems engaged primarily in the production of hydrogen gas. There are various methods through which hydrogen can be generated, each with distinct processes and technologies. The primary goal of these plants is to harness hydrogen's potential as a clean and versatile energy carrier.
According to the International Energy Agency, hydrogen can be produced from various feedstocks including natural gas, water, and biomass. The output of hydrogen is often utilized in energy applications, as well as in the chemical industry. In essence, hydrogen plants act as hubs where energy transformation occurs, specifically converting input materials into molecular hydrogen.
Historical Context and Development
The history of hydrogen plants is steeped in early scientific exploration. Hydrogen was first recognized as a distinct substance in the 18th century. Its industrial use began much later, in the early 20th century, as the importance of hydrogen as a fuel became apparent. The advent of the Haber-Bosch process for ammonia production marked a turning point. This method later paved the way for large-scale hydrogen production.
Over decades, advancements in technology and increased demand for clean energy have shaped the development of hydrogen plants. In recent years, global frameworks aimed at reducing greenhouse gases have accelerated investments in hydrogen technology. This historical context illustrates a continuous evolution toward cleaner, efficient methods of hydrogen production, such as steam methane reforming and electrolysis.
Within this landscape, hydrogen plants demonstrate both potential and challenges. The journey towards optimizing hydrogen production is significant, requiring understanding and adaptation to various economic, technological, and environmental factors.
"Hydrogen is seen as a key to the energy transition, as it can be produced from various resources and used in diverse applications."
Types of Hydrogen Production
Hydrogen production is central to the role hydrogen plays in the energy sector. Different methods yield varying qualities, efficiencies, and environmental impacts. Understanding these production types is crucial for both practical applications and future developments in hydrogen technology. The landscape of hydrogen production is constantly evolving, making it essential for professionals and researchers to keep abreast of these changes. Each method not only reflects technological advancements but also influences economic viability and sustainability.
Steam Methane Reforming
Steam methane reforming (SMR) stands as the dominant method for producing hydrogen globally, accounting for a significant portion of total hydrogen output. This process involves the reaction of natural gas with steam over a catalyst at high temperature. The primary chemical reaction can be simplified as:
[ \textCH_4 + \textH_2\textO \rightarrow \textCO + 3 \textH_2 ]\
Here, methane reacts with water to produce hydrogen and carbon monoxide. SMR is favored due to its relatively low cost and established technology. However, it has significant drawbacks, primarily concerning carbon emissions.
- Benefits:
- Considerations:
- Cost-effective and widely used
- High hydrogen yield
- Carbon dioxide emissions
- Dependence on fossil fuels
In light of the environmental impact, alternative hydrogen production methods are gaining attention.
Electrolysis of Water
Electrolysis of water represents an attractive method, especially for integrating renewable energy sources. The process involves splitting water into hydrogen and oxygen using electricity. The equation governing this reaction is:
[ \text2H_2\textO \rightarrow 2\textH_2 + \textO_2 ]\
Electricity supplied can be sourced from solar, wind, or hydroelectric energy, making this method particularly sustainable.
- Benefits:
- Considerations:
- Minimal direct emissions
- Compatible with renewable energy
- High operational costs
- Technological improvements are necessary for efficiency
Electrolysis continues to advance, drawing interest from researchers focusing on cost reduction and efficiency improvement.
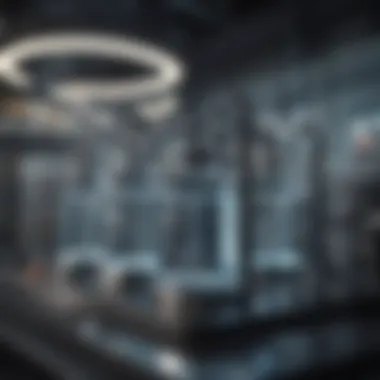
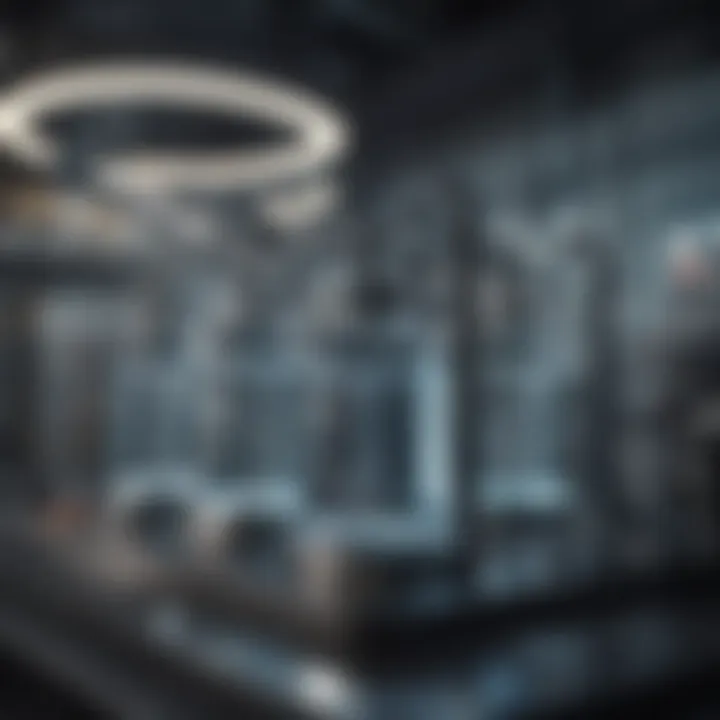
Gasification of Biomass
Gasification of biomass is another method that converts organic materials into hydrogen-rich gas. This process involves heating biomass and partially oxidizing it, which results in a synthesis gas made up of hydrogen, carbon monoxide, and carbon dioxide. One relevant reaction is:
[ \textC_6\textH_12\textO_6 \rightarrow \textH_2 + extCO + extCO_2 + \textH_2\textO ]\
This approach allows for the utilization of both agricultural residues and waste materials.
- Benefits:
- Considerations:
- Utilizes waste and reduces landfilling
- Renewable source of hydrogen
- Technology is less mature than SMR
- Variability in biomass feedstock quality
Focusing on optimizing biomass feedstocks can enhance the viability of this method.
Thermochemical Water Splitting
Thermochemical water splitting harnesses high temperatures to drive chemical reactions that produce hydrogen. This method typically requires metal oxides and heat sources such as concentrated solar power. The process can be complex, but a simplified reaction can be represented as:
It is a promising method, largely still in research phases.
- Benefits:
- Considerations:
- Potential for high efficiency
- No direct carbon emissions
- High operational temperatures necessitate advanced materials
- Commercialization is still forthcoming
Thermochemical splitting offers a unique avenue for hydrogen production but requires further research and development.
Components of Hydrogen Plants
The components of hydrogen plants serve as the backbone of their functionality and efficiency. Each element is crucial for the plant's overall operation, from production to delivery of hydrogen. Understanding these components is essential for evaluating the effectiveness and sustainability of hydrogen production.
Key components include production equipment, storage infrastructure, and transportation systems. Each of these elements not only plays a specific role but also contributes to the effectiveness of hydrogen plants in the broader context of energy production and environmental considerations.
Production Equipment
Production equipment refers to the machinery and systems that convert raw materials into hydrogen. This includes devices like electrolyzers for water splitting and reformers for extracting hydrogen from hydrocarbons. Each method demands robust and efficient equipment to maximize yield while minimizing energy consumption.
In designing hydrogen plants, selecting the right production equipment is vital. It affects the overall cost, efficiency, and environmental impact of hydrogen production. For example, modern electrolyzers are designed to operate on renewable energy sources, which enhances their sustainability profile. Equipment innovations focus on improving performance and reducing costs. Manufacturers regularly update their offerings, incorporating advances in materials, chemistry, and engineering.
Considerations for production equipment include:
- Integration with renewable energy sources
- Maintenance and operational efficiency
- Scalability to meet future demand
Storage Infrastructure
Proper storage infrastructure is vital for the efficacy of hydrogen plants. Given hydrogen's low density, effective storage solutions are needed. Common options include high-pressure gas cylinders and cryogenic tanks. Each method has its benefits and drawbacks.
- High-pressure tanks are often used for onboard storage in vehicles but require significant safety measures due to the pressures involved.
- Cryogenic storage entails cooling hydrogen to -253ยฐC, which allows storing larger quantities in a compact form.
Storage choices influence the economic feasibility of hydrogen projects. Effective storage solutions must also consider safety regulations and environmental impact, as hydrogen poses explosive risks. The design of storage systems should facilitate easy access and distribution while ensuring the highest safety standards.
Transportation Systems
Transportation systems are key to delivering hydrogen from production facilities to end-users. Various methods exist, including pipelines, trucks, and barges. Each mode has its unique advantages and limitations.
- Pipelines are cost-effective for large-scale distribution but require substantial investment in infrastructure. They are best suited for fixed, long-distance transport.
- Trucking provides flexibility for smaller quantities and can reach remote areas, though it may be less economical for large volumes.
Selecting transportation methods must consider:
- Distance to end-users
- Cost-effectiveness of infrastructure development
- Environmental impact and carbon footprint of transportation methods
Ultimately, optimizing these componentsโproduction equipment, storage infrastructure, and transportation systemsโguarantees that hydrogen plants run efficiently and contribute positively towards sustainable energy solutions.
"Understanding the components of hydrogen plants is the first step in harnessing their potential for a sustainable energy future."
When moving forward, it is crucial to continually assess the evolution of these components as technology advances and market demands shift.
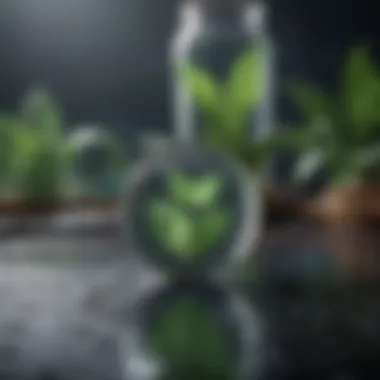
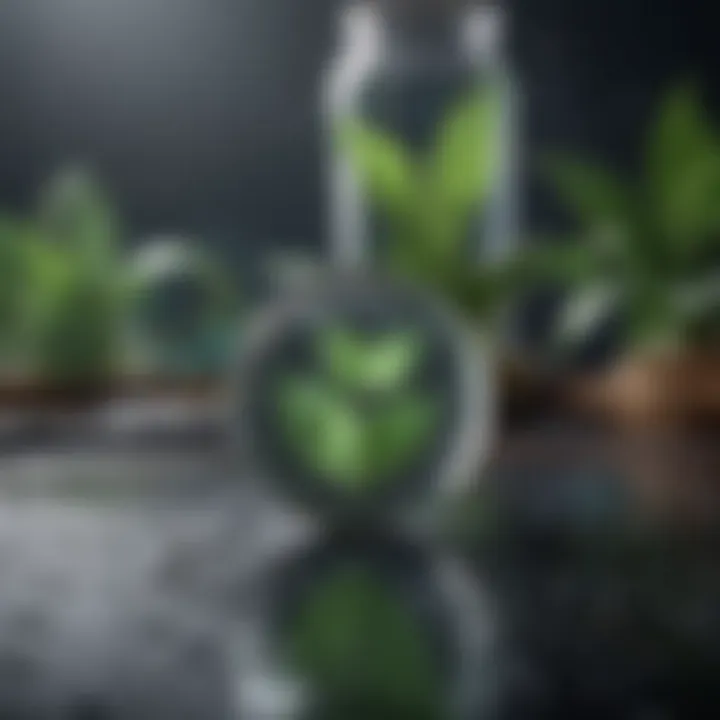
Technological Innovations in Hydrogen Production
Technological innovations are pivotal in the evolution of hydrogen production. As demands for cleaner energy alternatives grow, these advancements offer solutions that enhance efficiency, reduce costs, and minimize environmental impact. Understanding the key elements in this area is crucial for stakeholders in both academia and industry. Such innovations pave the way for a sustainable energy future, addressing urgent global challenges.
Advancements in Electrolysis
Electrolysis is an important process for hydrogen production, utilizing electricity to split water into hydrogen and oxygen. Recent advancements in electrolysis technologies have significantly increased efficiency. The introduction of proton exchange membrane (PEM) electrolysis has improved the overall energy conversion rate. This method operates at lower temperatures, allowing faster start-ups and more agile responses to fluctuating energy supplies. Additionally, integrating renewable energy sources, such as solar and wind, with electrolysis can lead to truly green hydrogen production. The result is a cleaner alternative that helps reduce carbon footprints.
Improved Catalysts for Hydrogen Production
Catalysts play a crucial role in facilitating hydrogen production reactions, making them faster and more efficient. Recent research focuses on developing cost-effective and highly active catalysts. For instance, nickel-based catalysts have gained attention due to their abundance and performance in steam methane reforming processes. Meanwhile, improvements in noble metal catalysts are also significant, enhancing their stability and effectiveness while addressing cost concerns. The ongoing exploration of non-metal catalysts presents further opportunities to optimize hydrogen generation processes.
Integration with Renewable Energy Sources
The integration of hydrogen production with renewable energy sources represents a significant breakthrough in achieving sustainable energy systems. By using excess electricity generated from renewable sources during peak production times, hydrogen can be produced without emitting greenhouse gases. This process not only balances energy supply and demand but also creates a storage medium for renewable energy. Consequently, hydrogen can serve as a vital enabler for energy transition, providing flexibility and reliability in energy systems. By making use of renewable resources more effectively, hydrogen helps further sustainable development goals.
"Innovations in hydrogen production technology will be essential to meet future energy demands and tackle climate change."
Through these innovations, the hydrogen production sector can evolve to meet the growing energy requirements, fostering a more sustainable and efficient energy landscape. As technologies advance, the path to integrating hydrogen solutions into global energy systems becomes increasingly feasible.
Environmental Considerations
The environmental implications of hydrogen plants are pivotal in shaping not only energy policies but also public perception of hydrogen as a clean fuel alternative. The urgency of addressing climate change intensifies the need for sustainable energy solutions. Understanding how hydrogen production impacts carbon emissions, water resources, and overall ecological health is essential for responsible development.
Carbon Emissions and Climate Impact
Hydrogen production methods vary significantly in their carbon footprints. Traditional methods, such as Steam Methane Reforming, release substantial carbon dioxide. However, advancements in Cleaner Hydrogen Production are underway. For instance, when hydrogen is produced from renewable sources, the embodied carbon emissions can be minimized significantly.
The role of hydrogen in reducing greenhouse gas emissions becomes critical when considering its potential uses. Hydrogen can serve as a clean fuel alternative in transportation and industrial sectors. Thus, it stands as an important contender against fossil fuels.
Evaluating the lifecycle emissions associated with hydrogenโfrom production to usageโprovides insight into its potential impacts on climate change. Striving for methods that utilize renewable energy in hydrogen production can lead to a net reduction in global emissions.
"Investing in hydrogen production from renewable resources might be one of the keys to achieving climate goals."
Water Usage and Sustainability Issues
Water is a critical input in hydrogen production, particularly in electrolysis and thermochemical processes. Understanding the relationship between water use and hydrogen production is necessary, especially in regions facing water scarcity. For example, electrolysis requires significant amounts of water, leading to sustainability concerns where water resources are limited.
Additionally, the quality of water used can affect the efficiency of hydrogen production. Utilization of advanced treatment methods to ensure water purity can mitigate some negative environmental impacts. Furthermore, exploring alternatives to water-intensive methods can lead to more sustainable practices.
The balance between hydrogen production and water resource management is complex but essential. Sustainable practices in hydrogen plants can help ensure that these facilities do not exacerbate existing issues related to water scarcity or pollution. This consideration is particularly relevant in the context of future investments and policies aimed at promoting hydrogen as a cornerstone of clean energy.
In summary, addressing both carbon emissions and water usage will enhance the understanding of hydrogen's role in a sustainable future. It is crucial for professionals, researchers, and policy-makers to navigate these complex considerations when evaluating hydrogen plant development.
Economic Factors in Hydrogen Plant Development
Economic aspects are crucial in the development of hydrogen plants. They shape the feasibility, scalability, and sustainability of operations within this industry. Understanding these factors can aid stakeholders in making informed decisions on investments and policies.
Cost of Hydrogen Production
The cost of hydrogen production remains a vital topic. Production methods such as steam methane reforming and electrolysis come with different cost structures. For example, steam methane reforming has been the dominant technology. It often yields hydrogen at a lower average cost compared to electrolysis.
However, over time, the costs associated with electrolysis have decreased. This shift could influence future trends toward renewable methods.
Some specific inputs that affect these costs include:
- Raw materials: The price of feedstocks like natural gas impacts overall production costs.
- Capital investment: Significant upfront costs related to equipment and technology need consideration.
- Operational costs: Ongoing expenses for maintenance and labor play an important role.
Understanding these elements helps in gauging the economic viability of hydrogen plants.
Market Trends and Demand for Hydrogen
The market for hydrogen is evolving rapidly. As industries seek to reduce carbon emissions, the demand for hydrogen is increasing. Various sectors, such as transportation and manufacturing, are exploring hydrogen as a clean energy source.
Significant market trends include:
- Growing interest in renewable hydrogen: Companies are investing in technologies that lead to greener production methods.
- Global policies promoting hydrogen: Governments are instituting policies that support hydrogen adoption.
- Investment in infrastructure: The development of infrastructure for distribution and storage is crucial in meeting future needs.
Increased demand can drive up prices, making it more attractive for investors.
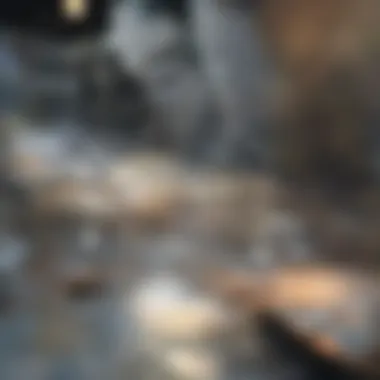
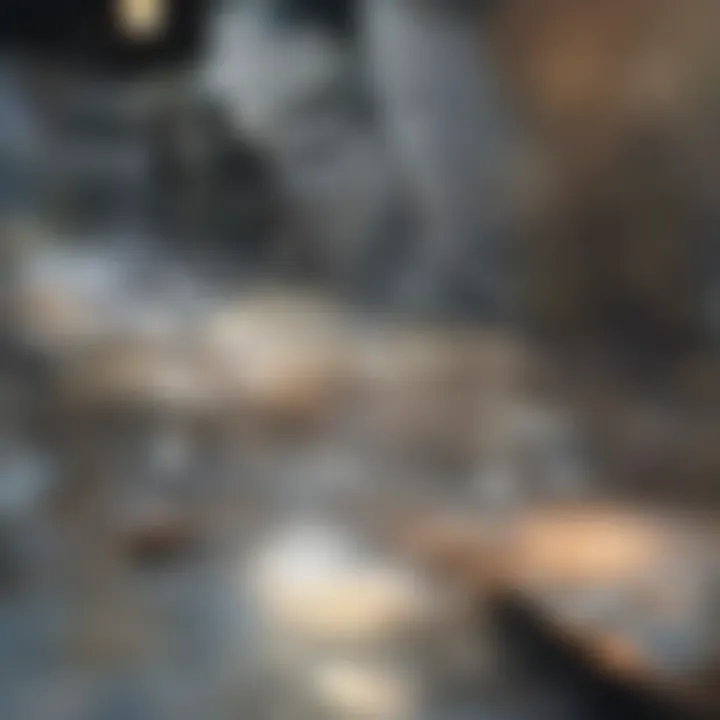
Investment Opportunities in Hydrogen Technology
Investment opportunities in hydrogen technology are expanding. Governments and private sectors are recognizing the potential of hydrogen. This growing awareness is leading to substantial funding for research and development.
Investors might consider:
- Innovative startups: Companies focused on hydrogen production technologies offer high-growth potential.
- Partnerships with established firms: Collaborations with big industries can help mitigate risks.
- Public sector projects: Participating in government-funded projects can provide steady returns.
Investing in hydrogen technologies now can yield significant benefits as the world transitions towards more sustainable energy sources.
"The future of energy is in hydrogen, and those who invest in this field early will likely reap the rewards."
Regulatory and Policy Framework
The regulatory and policy framework surrounding hydrogen plants plays a fundamental role in shaping the hydrogen economy. This framework encompasses a series of government regulations, incentives, and international agreements that can significantly influence the development and operation of hydrogen plants. It establishes guidelines for safety, environmental protection, and economic viability. Without a supportive policy environment, innovation may stagnate, investment might wane, and the full potential of hydrogen as a clean energy source could remain unrealized.
Government Policies Promoting Hydrogen
National governments are increasingly recognizing the necessity of hydrogen in their energy policies as they seek sustainable alternatives to fossil fuels. Numerous nations have implemented policies aimed at fostering hydrogen production and usage. These policies often include:
- Subsidies and Financial Incentives: Governments provide financial support to lower the cost barrier for hydrogen production technologies. This helps to facilitate research and development.
- Regulatory Frameworks: Establishing safety and operational standards that hydrogen plants must adhere to ensures compliance with environmental laws and safety regulations.
- Research Grants: Funding for research initiatives related to hydrogen technologies propels innovation in efficient hydrogen production and fuel cell applications.
Countries such as Germany, Japan, and South Korea have set ambitious hydrogen strategies, highlighting their commitment to reducing carbon emissions and promoting clean energy technologies. For example, Germany's National Hydrogen Strategy aims for significant investment in green hydrogen production facilities, which is essential for transitioning to a low-carbon economy.
International Treaties and Agreements
International collaboration is crucial in advancing the hydrogen sector. Treaties and agreements create a consistent framework that transcends national borders. These can encourage technology transfer, share best practices, and promote investment in hydrogen infrastructure. Some key aspects of these agreements include:
- Technology Sharing: Agreement on shared technologies can expedite the deployment of hydrogen systems across countries.
- Cross-Border Funding Initiatives: Often, international funds are set up to support hydrogen projects, which can boost capacity in developing countries.
- Environmental Standards: Treaties can establish comprehensive environmental standards that guide hydrogen production, guiding countries toward sustainable practices.
For instance, the Paris Agreement aims to limit global warming and has resulted in countries committing to invest in renewable technologies, including hydrogen. Through such frameworks, nations can work together toward common objectives in reducing greenhouse gas emissions.
"The policies and agreements governing hydrogen technology not only drive investment but also shape a sustainable energy future."
Future Outlook for Hydrogen Plants
The future of hydrogen plants presents a critical intersection between innovation and sustainability. As the global energy landscape shifts towards cleaner alternatives, hydrogen's potential as a versatile energy carrier becomes increasingly apparent. This section will explore the long-term viability of hydrogen systems and the emerging research directions that could either propel or hinder their development.
Long-term Viability of Hydrogen Systems
Hydrogen systems are positioned to play a significant role in the transition to a low-carbon economy. Their long-term viability hinges on several factors. Firstly, the advancement in production technologies, specifically electrolytic methods, shows promise for reducing costs. As electrolyzers become more efficient and less expensive, the overall feasibility of hydrogen production improves.
Moreover, energy storage is another area where hydrogen exhibits strength. Hydrogen can store large amounts of energy that can be utilized when demand peaks, effectively acting as a buffer for intermittent renewable sources like wind and solar. This capability to balance supply and demand enhances the reliability of energy grids.
Additionally, the decarbonization of industries, particularly in sectors such as steelmaking and chemical production, can be achieved with hydrogen. Utilizing hydrogen as a feedstock in these challenging areas could significantly lower carbon footprints and move industries towards sustainability. Thus, the integration of hydrogen throughout different sectors indicates a robust future for hydrogen systems.
Research Directions and Challenges Ahead
While the outlook appears promising, several challenges must be addressed to harness hydrogen's full potential. Research is crucial in various domains to overcome current limitations. Key areas of focus include:
- Improving Production Efficiency: Enhancing the efficiency of both steam methane reforming and electrolysis will be essential to making hydrogen production more environmentally friendly and economically viable.
- Development of Advanced Storage Solutions: Current storage methods are often costly or inefficient. Research into new materials for storage tanks or chemical hydrogen storage could improve hydrogen's practicality.
- Widespread Infrastructure Development: The infrastructure required for hydrogen production, storage, and transportation must be expanded. Policy support and research into economically viable distribution networks are paramount.
- Public Acceptance and Awareness: Engaging with communities to promote the safety and benefits of hydrogen is essential. Continued education and outreach will build public confidence in hydrogen solutions.
The future trajectory of hydrogen plants will depend not only on technological advancements but also on collaborative efforts among governments, academia, and the private sector.
In summary, the future of hydrogen plants is anchored in their potential to address significant energy challenges. To transition successfully, it is imperative that ongoing research tackles existing barriers, enabling hydrogen to contribute substantially to the worldโs energy matrix.
Ending
The conclusion serves as a crucial segment in this exploration of hydrogen plants. It encapsulates the main discussions and reveals the implications of the findings throughout the article. The careful summarization of key insights not only aids in reinforcing the material for the reader but also emphasizes the significance of hydrogen technology in contemporary energy frameworks.
A major takeaway involves recognizing hydrogen's potential as a clean energy source. Hydrogen plants are central to the production of hydrogen, made possible through various methods discussed earlier. As the world moves towards sustainable and environmentally friendly energy solutions, the role of hydrogen becomes increasingly vital.
Moreover, understanding the environmental considerations linked with hydrogen production provides valuable context. The benefits of reducing carbon emissions and promoting cleaner technologies reflect broader societal goals when addressing climate change.
The economic factors determining hydrogen plant viability also draw attention. Cost-effectiveness, market trends, and potential investments in this sector are paramount for stakeholders involved in energy production.
This comprehensive overview of hydrogen plants thus highlights not only the technologies and methods employed but also promotes an awareness of their broad implications.
"Investing in hydrogen technology may well represent a defining path towards a sustainable energy future."
Summarizing Key Insights
In summation, this article has navigated the intricate landscape of hydrogen plants. The following key insights emerge:
- Diverse Production Methods: Various hydrogen production techniques exist, each with its own advantages and challenges, including steam methane reforming and electrolysis.
- Technological Innovation: Continuous advancements in technology are crucial. For example, improved catalysts and integration of renewable energy sources can enhance production efficiency and reduce costs.
- Environmental Impact: The significance of understanding the ecological footprint of hydrogen plants is evident. Assessing water usage and reducing carbon emissions is essential for sustainable operation.
- Economic Viability: The current market dynamics indicate rising demand for hydrogen as an energy source. Cost considerations directly influence investment decisions in this field.
- Regulatory Landscape: Government policies and international agreements are shaping the hydrogen sector. These regulations either incentivize or hinder development.
The future outlook, while presenting challenges, also reveals promising directions for ongoing research and development. Hydrogen's role as a pivotal component of the global energy matrix is clear, making the insights gathered in this article not only informative but critical for stakeholders and future professionals in the energy sector.