RNA Stabilizers: Mechanisms and Future Applications
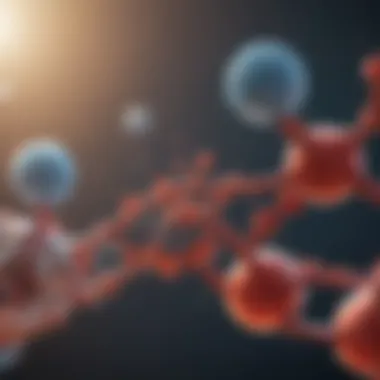
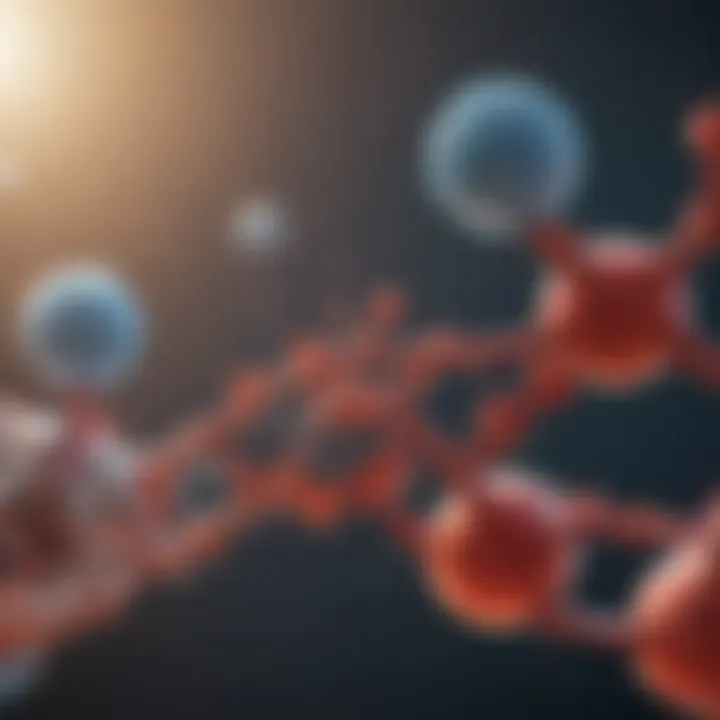
Intro
The significance of RNA stabilizers in biological and experimental fields is profound. These compounds play a crucial role in enhancing the stability and integrity of RNA molecules, which is a vital aspect for various applications in molecular biology and therapy. A detailed look into the mechanisms, applications, and future possibilities associated with RNA stabilizers reveals their potential and importance in current scientific research and medical advancements.
In this section, we will provide an overview of pertinent research relating to RNA stabilizers, examining how they function and the contexts in which they are applied. Understanding these aspects will establish a foundation for recognizing future directions and the challenges faced in RNA stabilization.
Research Overview
Summary of Key Findings
Research indicates that RNA stabilizers can effectively prolong the half-life of RNA molecules. This characteristic is especially important in therapeutic settings, where delivering RNA for gene therapy can face obstacles such as degradation before reaching the target cells.
RNA stabilizers improve the efficacy of mRNA vaccines. For instance, stabilizing modifications can enhance the translational efficiency and immune response.
Methodologies Employed
Various methodologies have been applied to study the effects of RNA stabilizers. Some notable approaches include:
- In vitro assays: Testing RNA stability and integrity using specific stabilizers in controlled laboratory settings.
- Cellular assays: Observing the effects of RNA stabilizers on RNA delivery and performance within live cells.
- Animal models: Assessing the therapeutic efficacy of RNA stabilizers through in vivo studies.
These methodologies provide insights into how RNA stabilizers enhance RNA functionality. Understanding these methods can inform further research and application in real-world contexts.
In-Depth Analysis
Detailed Examination of Results
The results indicate that RNA stabilizers vary in effectiveness based on their chemical structures and interactions with RNA molecules. Some stabilizers can protect RNA from degradation by cellular enzymes, while others may alter RNA folding and conformation to improve stability.
Comparison with Previous Studies
Previous studies have highlighted the limitations associated with traditional RNA stabilization methods, such as the use of conventional preservatives. Contrastingly, recent findings propose novel RNA stabilizers that offer better efficiency and lower toxicity levels. These improvements mark a significant step forward.
RNA stabilizers are not just tools; they represent a leap forward in our understanding of RNA molecules and their potential to drive meaningful change in medicine and research.
Prologue to RNA Stabilizers
RNA stabilizers play a crucial role in ensuring the integrity and longevity of RNA molecules within biological systems. Understanding these stabilizers is essential due to their diverse applications ranging from fundamental research to practical therapeutic measures. Their significance is underscored by the complexity of RNA metabolism and the potential consequences of RNA degradation. By enhancing the stability of RNA, these stabilizers facilitate various important cellular functions.
Definition of RNA Stabilizers
RNA stabilizers are compounds or agents that enhance the stability of RNA molecules, preventing their degradation or loss of functionality. These can include nucleotide analogues, chemically modified oligonucleotides, and small natural compounds, among others. The primary function of RNA stabilizers is to promote the preservation of RNA structure and function, impacting how RNA fulfills its role in the cell.
Importance in Cellular Processes
The stabilization of RNA is vital for numerous cellular processes. RNA molecules are involved in protein synthesis, gene expression regulation, and more. If RNA degrades too quickly, it can lead to incomplete protein synthesis or altered cellular responses. By utilizing RNA stabilizers, researchers can ensure accurate measurement and observation of RNA activities. This is particularly important in scenarios where the stability of RNA affects experimental outcomes or therapies aimed at specific RNA targets.
Historical Context
The study of RNA stabilizers has evolved significantly over the years. Initial research focused primarily on understanding RNA structures and functions. As scientific techniques progressed, the need for RNA stability became apparent, leading to deeper investigations into various stabilizing agents.
In the late 20th century, the discovery of chemically modified oligonucleotides brought forth a new class of stabilizers, greatly enhancing researchersβ ability to work with RNA in vitro and in vivo. As technology continues to advance, the landscape of RNA stabilizers expands, leading to new applications in therapeutic areas and diagnostics. This historical perspective shapes our current understanding and guides future innovations in RNA stabilization research.
"RNA stabilizers are critical for the advancement of molecular biology and therapeutic applications."
Through the study and application of RNA stabilizers, researchers can unlock new potentials across various fields, including genetics and biotechnology, highlighting their pivotal role in modern science.
Mechanisms of Action
Understanding the mechanisms of action of RNA stabilizers is essential for grasping how they enhance the stability and functionality of RNA molecules. These mechanisms reveal how RNA stabilizers interact chemically with RNA and influence its overall structure. Moreover, they inform applications in various research and therapeutic contexts, serving as a foundation for developing more effective RNA-based strategies. By dissecting these mechanisms, researchers can identify the optimal conditions for using stabilizers, improving the outcomes of experiments and therapies.
Chemical Interactions with RNA
RNA stabilizers primarily function through specific chemical interactions with RNA molecules. These interactions may include hydrogen bonding, pi-stacking, and electrostatic interactions. Such bonds can help to protect RNA from degradation and enhance its stability.
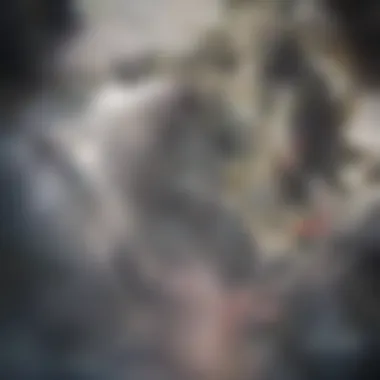
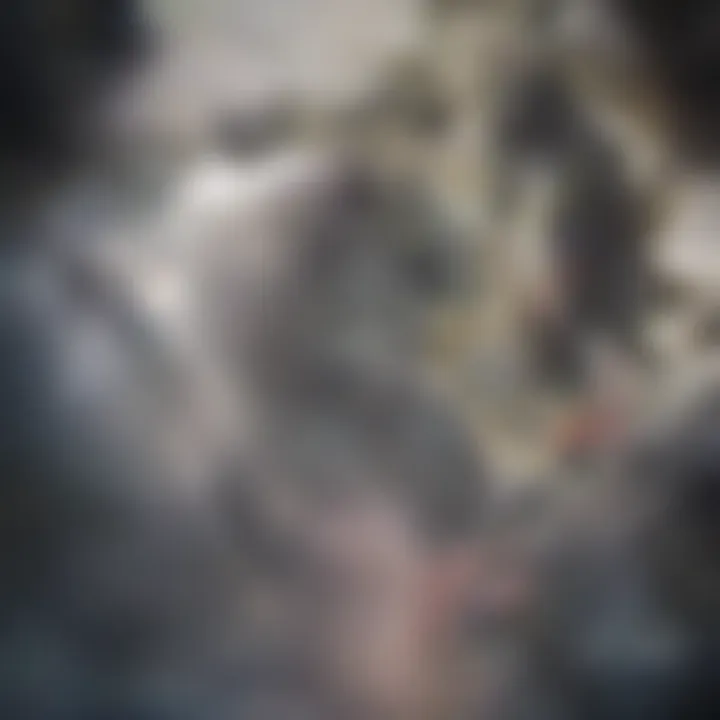
- Hydrogen bonding: This occurs when the stabilizer forms bonds with the functional groups present on the RNA, creating a protective layer.
- Pi-stacking: Aromatic stabilizers can engage in pi-stacking interactions with the RNA bases, limiting accessibility to degrading enzymes.
- Electrostatic interactions: Many stabilizers have charged groups that can interact with the negatively charged phosphate backbone of RNA, leading to increased binding affinity.
Understanding these interactions helps researchers tailor the design and application of RNA stabilizers for better performance in laboratory and therapeutic settings.
Influence on RNA Structure
The structural configuration of RNA is critical to its biological function. RNA stabilizers can significantly influence RNA folding and conformation. The presence of stabilizers may promote specific structures that are more resistant to degradation or enhance the RNA's functional capabilities.
For instance, stabilizers may induce a conformational change in an RNA molecule that retains its activity longer. The resulting specific three-dimensional shapes can dictate how RNA interacts with proteins and other nucleic acids. In some cases, this influence extends to higher-order structures, such as ribozymes or ribonucleoproteins, which are crucial for various cellular processes.
Furthermore, modifications introduced by stabilizers may lead to a better understanding of RNA behavior under physiological conditions, aiding in the design of RNA-targeted therapies and assays.
Effects on RNA Degradation Pathways
A critical aspect of RNA stabilizers is their role in modulating RNA degradation pathways. In living cells, RNA is continuously subjected to degradation by ribonucleases that are designed to regulate gene expression and RNA turnover. RNA stabilizers can interfere with these pathways, prolonging the half-life of RNA molecules.
- Blocking ribonuclease access: By binding selectively to RNA, stabilizers can physically block the ribonucleases from interacting with their targets.
- Mimicking cellular structures: Some stabilizers resemble the natural components of the cellβs RNA metabolism machinery, leading to enhanced protection against degradation.
- Altering degradation kinetics: Stabilizers can impact the rate at which RNA enters degradation pathways, delaying RNA turnover and allowing for more sustained expression or functionality.
In summary, understanding mechanisms of action contributes to the effective utilization of RNA stabilizers, providing insights that drive innovations in RNA research and application.
Types of RNA Stabilizers
Understanding the different types of RNA stabilizers is essential for researchers and practitioners alike. These compounds play a crucial role in enhancing the stability of RNA molecules, which is vital for reliable experimental outcomes and therapeutic applications. Each type presents distinct benefits and considerations, impacting their efficacy and suitability for specific uses.
Nucleotide Analogues
Nucleotide analogues are modified nucleotides designed to mimic natural RNA bases. Their importance lies in their ability to improve RNA stability and resistance to degradation. Unlike natural nucleotides, these analogues often possess chemical modifications that can enhance binding affinity to complementary sequences and inhibit enzymatic degradation. This results in prolonged activity of RNA in various applications.
Popular examples include 2'-O-methyl and 2'-O-ethyl ribonucleotides. These modifications significantly increase the resistance of RNA to nucleases, which are enzymes that typically degrade RNA molecules. The integration of nucleotide analogues has proven beneficial in fields such as antisense therapy and gene regulation.
However, the application of nucleotide analogues requires careful consideration. Their structural differences might influence hybridization properties and biological activity. Synthesis complexities and costs can also be a factor, which researchers must keep in mind when designing experiments or therapeutic strategies.
Chemically Modified Oligonucleotides
Chemically modified oligonucleotides consist of RNA or DNA sequences that have undergone specific modifications to enhance their stability and efficacy. These modifications can include changes in the backbone structure, sugar moieties, or base alterations.
A key benefit of chemically modified oligonucleotides is their reduced susceptibility to enzymatic degradation. For instance, phosphorothioate modifications introduce a sulfur atom into the backbone of the oligonucleotide, granting it increased resistance against nucleases. This property is crucial when oligonucleotides are used in therapeutic settings where prolonged circulation in the bloodstream is often required.
Another common type of modification is the locked nucleic acid (LNA) and the bridged nucleic acid (BNA). These modifications demonstrate high affinity for complementary sequences, leading to potent gene silencing effects. Consequently, the applications of chemically modified oligonucleotides span across diverse areas, including RNA interference and diagnostic assays.
However, the implications of various modifications must be studied extensively. Some modifications may alter the pharmacokinetics and biodistribution of the oligonucleotides, impacting their effectiveness in vivo. Thus, it becomes important for scientists to balance the advantages against possible limitations of modified sequences.
Small Molecules and Natural Compounds
Small molecules and natural compounds represent another class of RNA stabilizers. These substances can enhance RNA stability through various mechanisms, including direct binding or indirectly modulating the RNA environment within cells.
For example, certain flavonoids and polyphenolic compounds serve as natural RNA stabilizers by binding to RNA and preventing degradation. This interaction could potentially stabilize RNA secondary and tertiary structures, enhancing their functional interactions. Likewise, small molecules can be designed to disrupt RNA-degrading pathways, prolonging RNA half-life effectively.
The use of small molecules is appealing due to their potential for low toxicity and ease of administration. However, their specificity and optimization for desired outcomes remain critical hurdles. The risk of off-target effects can arise if they interact with unintended molecular partners, which can complicate the interpretation of experimental data.
Incorporating these compounds in RNA research and application requires thorough evaluation to understand their interaction dynamics better. Recognizing both their advantages and potential pitfalls is essential for maximizing the effectiveness of RNA stabilizers in diverse fields.
Applications in Research
Research into RNA stabilizers is vital for advancing the field of molecular biology and related disciplines. These stabilizers play a critical role in enhancing the integrity and longevity of RNA molecules, which are essential for numerous biological processes. Proper stabilization of RNA can significantly improve experimental outcomes and biological understanding. The varied applications of RNA stabilizers highlight their importance in both fundamental research and practical applications.
Molecular Biology Techniques
Molecular biology relies heavily on RNA manipulation and analysis. RNA stabilizers are crucial in techniques such as reverse transcription polymerase chain reaction (RT-PCR). By maintaining RNA integrity, these stabilizers ensure that the reverse transcription process is efficient. As a result, high-quality cDNA synthesis occurs, increasing the reliability of subsequent amplification steps.
Moreover, in methods like Northern blotting and RNA sequencing, RNA stabilizers help preserve the native structure of RNA samples. The preservation ensures accurate representation of transcript levels and splicing variants. This practice leads to more valid conclusions in experiments and strengthens findings across various studies.
RNA Quantification Methods

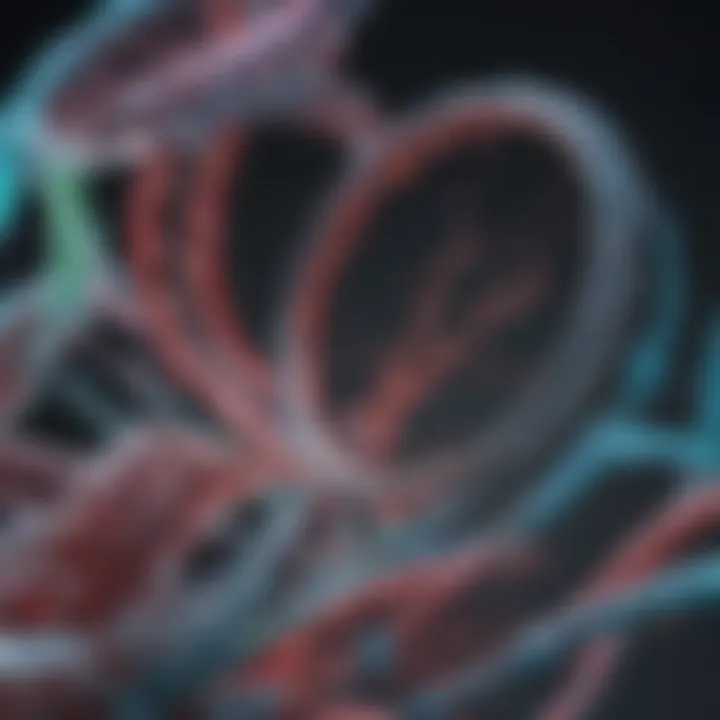
In research, quantification of RNA is necessary for understanding gene expression and biological functions. Stabilizers contribute to more accurate RNA quantification methods such as quantitative PCR and microarrays. By preventing degradation, the original concentration of RNA is maintained prior to analysis. This accuracy in quantification can determine the levels of specific RNA, which directly correlates with the expression of genes. With reliable measurements, researchers can draw more meaningful insights into cellular functions and disease mechanisms.
In addition to quantitative PCR, RNA stabilizers uplift the reliability of other techniques. For instance, in high-throughput sequencing projects, consistent RNA quality results in better data quality. This is crucial for downstream analysis, including differential expression studies and functional genomics explorations.
Gene Expression Studies
Gene expression studies explore the dynamic changes in RNA levels under various conditions. RNA stabilizers enhance this area by ensuring that samples remain intact during collection and processing. By preserving RNA integrity, these stabilizers reduce variability caused by degradation.
Critical insights gained from gene expression studies inform fields such as developmental biology and disease research. For example, in cancer research, analyzing differential RNA expression can highlight oncogenes or tumor suppressors. RNA stabilizers allow for these comparisons to be made with increased confidence, contributing to discoveries that could lead to important therapeutic advances.
"Utilizing RNA stabilizers directly affects the quality of research outcomes and is essential for reproducibility in experiments."
The incorporation of RNA stabilizers in research protocols not only optimizes techniques but also bolsters the credibility of findings. By addressing the challenges of RNA degradation, researchers can focus on obtaining valuable data that drives scientific understanding forward.
Therapeutic Uses of RNA Stabilizers
The therapeutic applications of RNA stabilizers are significant in modern medicine. With the rise of RNA-based therapies, the role of these stabilizers has become essential. They enhance the durability and functionality of RNA molecules in various treatments, leading to improved patient outcomes.
RNA Interference Applications
RNA interference (RNAi) is a crucial cellular mechanism used to silence specific genes. RNA stabilizers play a key role in optimizing RNAi by preventing degradation of small interfering RNA (siRNA) molecules. This stabilization ensures a longer half-life of siRNA in the biological environment, thus increasing its effectiveness.
The use of RNA stabilizers not only boosts the potency of siRNA therapies but also helps in designing effective delivery systems. By stabilizing the siRNA, researchers can achieve better results in gene silencing, which is critical for therapies targeting diseases like cancer and viral infections. In such cases, timing and dosage are essential, and RNA stabilizers can help control these factors more reliably.
Antisense Oligonucleotide Therapies
Antisense oligonucleotides (ASOs) are short, single-stranded DNA or RNA molecules. These are designed to bind specifically to target RNA. The role of RNA stabilizers in ASO therapies is paramount. They enhance the binding affinity and bioavailability of ASOs, ensuring they reach the intended target efficiently.
In the context of genetic disorders, ASOs have shown promise in correcting gene expression. With RNA stabilizers, the therapeutic efficacy increases, leading to improved clinical outcomes. Overall, the incorporation of stabilizers is a game-changer in developing ASO-based therapies, increasing their reliability in various treatment settings.
mRNA Vaccine Stability
The development of mRNA vaccines has revolutionized preventive medicine, particularly highlighted during the COVID-19 pandemic. RNA stabilizers ensure the stability of mRNA in vaccine formulations. This stability is crucial for maintaining the integrity of the mRNA, allowing for effective translation into proteins that trigger an immune response.
Factors such as temperature and time can impact mRNA stability. RNA stabilizers allow vaccines to withstand harsh conditions, ultimately leading to more effective vaccines. It is essential for enhancing the shelf-life and effectiveness of mRNA vaccines. As vaccination efforts continue globally, the role of RNA stabilizers in vaccine formulation will remain a focal point of research in biotechnology.
"RNA stabilizers are becoming an indispensable part of therapeutic strategies involving RNA therapies, including vaccines and gene therapies."
In summary, RNA stabilizers have significant therapeutic value in the applications of RNA interference, antisense oligonucleotide therapies, and mRNA vaccine stability. These stabilizers not only enhance the performance of RNA-based treatments but also open new avenues for research and improved healthcare solutions.
RNA Stabilization in Diagnostics
RNA stabilization plays a crucial role in diagnostics across various fields. The accurate detection and quantification of RNA biomarkers are essential for understanding disease mechanisms, guiding treatment decisions, and monitoring therapeutic outcomes. Without proper RNA stabilization, the integrity of biomolecular samples may suffer, leading to unreliable results. This section focuses on the importance of RNA stabilization in enhancing biomarker detection and preserving RNA integrity in samples.
Enhancing Biomarker Detection
Biomarkers are indicators of biological processes, disease states, or responses to therapeutic interventions. The detection of RNA biomarkers can inform about conditions such as cancer, infectious diseases, and genetic disorders. RNA stabilization enhances biomarker detection by minimizing degradation during sample collection, transport, and analysis.
Some key elements of enhancing biomarker detection include:
- Stabilization Solutions: Using RNA stabilization solutions helps maintain the integrity of RNA during sample collection. These solutions inhibit the enzymes that degrade RNA and prevent the introduction of contaminants.
- Optimized Protocols: Establishing clear protocols for sample handling is vital. Clear guidelines help ensure consistency and reduce variability in results, increasing the reliability of detected biomarkers.
- Sensitive Detection Methods: Advanced detection methods, including quantitative polymerase chain reaction (qPCR) and next-generation sequencing (NGS), require intact RNA. The use of RNA stabilizers ensures that high-quality RNA is available for these sensitive assays.
"The application of RNA stabilization technologies can significantly impact the reliability of biomarker detection assays."
Preservation of RNA Integrity in Samples
Preserving RNA integrity in biological samples is vital for diagnostic accuracy. Various factors, such as temperature, time of storage, and mechanical stress, can adversely affect RNA stability. Addressing these factors through stabilization strategies is key to ensuring accurate diagnostic outcomes.
Important considerations for the preservation of RNA integrity include:
- Storage Conditions: Proper storage conditions are essential. Samples should be kept at low temperatures to slow down degradation processes. In some cases, using flash freezing techniques may be beneficial.
- Sample Type Considerations: Different sample types, like blood, tissue, or saliva, present distinct stabilization challenges. Tailoring the approach to the specific sample type can lead to improved outcomes and more reliable results.
- Quality Control Measures: Implementing stringent quality control measures ensures that the RNA remains intact. Routine assessment of RNA quality using methods like agarose gel electrophoresis or spectrophotometric analysis can be useful to verify sample integrity prior to analysis.
In summary, RNA stabilization is pivotal in diagnostics, impacting both biomarker detection and the preservation of RNA integrity in samples. By utilizing effective stabilization techniques, researchers and clinicians can enhance the quality and reliability of diagnostic tests.
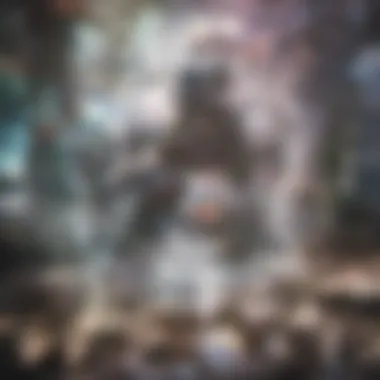
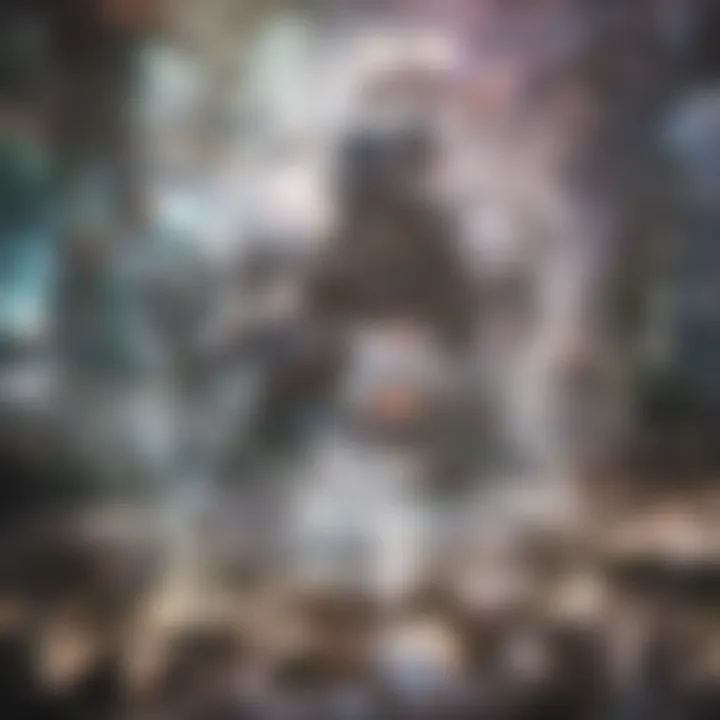
Challenges in RNA Stabilization
Stabilizing RNA molecules presents various challenges that researchers and clinicians must navigate. While RNA stabilizers play a critical role in enhancing RNA stability, there are factors and conditions that can complicate their effectiveness. Understanding these challenges is fundamental for improving research outcomes and therapeutic applications.
Stability vs. Activity Trade-offs
One major challenge in RNA stabilization is achieving a balance between stability and biological activity. While stabilizing RNA can prolong its half-life within cellular environments, this increased stability may inadvertently affect its function. For instance, certain RNA stabilizers may interfere with the RNA's ability to bind with proteins or other nucleic acids.
When designing experiments or therapies, it is crucial to consider how much stabilization is necessary. If the stabilization is excessive, it could lead to the RNA being resistant to degradation when it needs to be cleared from the system or transformed into another molecule. This gives rise to potential side effects. Researchers must therefore critically assess the required stability against the necessity for activity to ensure that RNA fulfills its intended biological roles without adverse consequences.
Component Toxicity and Biocompatibility
Another significant area of concern involves the toxicity and biocompatibility of the stabilizing agents being utilized. Some RNA stabilizers may exhibit cytotoxic effects, leading to an unintended influence on cellular health and function. For example, small molecules designed for RNA stabilization might disrupt cell membranes or interfere with other cellular components, which could ultimately compromise the validity of experimental results.
Before deploying an RNA stabilizer in experimental or therapeutic settings, it is crucial to evaluate its biocompatibility. Evaluating this aspect not only ensures that the stabilizer will not hinder cellular processes but also checks for any long-term effects that could arise from its use. Comprehensive toxicity assessments through various in vitro and in vivo studies are essential to establish safe usage protocols.
Methodological Limitations
Finally, methodological limitations pose challenges in RNA stabilization research. Many techniques for analyzing RNA stability, such as RNAseq or northern blotting, have their own set of constraints. For instance, these methods may not adequately reflect the actual stability dynamics of RNA in living cells. They can also introduce artifacts that misrepresent RNA's behavior in vivo.
Moreover, variations in cell type, experimental conditions, and the presence of interacting partners can impact results. Hence, researchers need to develop and standardize methodologies that accurately measure RNA stability in biological systems. Employing a multi-faceted approach while considering these limitations is essential for generating reliable data.
The challenges in RNA stabilization are manifold, necessitating continuous research and innovative thinking to optimize the effectiveness of RNA stabilizers across applications.
In summary, addressing these challenges β the balance of stability and activity, the issues surrounding toxicity and biocompatibility, and the limitations of current methodologies β is crucial for advancing the effective use of RNA stabilizers. Understanding and overcoming these obstacles will enhance the efficacy of RNA-based therapies and improve research outcomes.
Future Directions in RNA Stabilization Research
The landscape of RNA stabilization research holds significant promise for advancing various scientific fields. As RNA stabilizers continue to evolve, their mechanisms and applications open up new avenues. Ongoing advancements in this area are vital for both foundational research and practical applications, particularly in disease treatment and diagnostics.
Emerging Technologies and Innovations
New technologies are rapidly changing the way RNA stabilizers are developed and utilized. Innovations such as high-throughput screening techniques allow for faster identification of effective stabilizing agents. Moreover, advancements in nanotechnology present opportunities to enhance RNA delivery and stability.
Researchers are also exploring the use of artificial intelligence to predict interactions between RNA and stabilizers. This can streamline the design of custom stabilizers tailored to specific RNA structures. By employing such technologies, significant improvements can be realized in both efficiency and efficacy of RNA applications.
Integration with CRISPR and Gene Editing
The integration of RNA stabilizers with CRISPR technology represents a fascinating avenue of exploration. The CRISPR-Cas9 system has transformed gene editing, yet it often encounters challenges such as RNA instability during delivery. RNA stabilizers can play a critical role in enhancing the stability of guide RNAs used in CRISPR applications.
Furthermore, stabilizers may improve the overall efficiency of gene editing by ensuring that the RNA components remain active for a more extended period. This combination could lead to more reliable and effective gene editing strategies, particularly for therapeutic purposes.
Potential in Personalized Medicine
Personalized medicine stands to benefit immensely from advancements in RNA stabilization. By tailoring RNA stabilizers to individual patients' genetic profiles, therapies can become more effective. This precision approach requires a deep understanding of RNA interactions and the factors that govern stability and degradation.
The focus on personalized treatments can change the landscape of disease management. For instance, RNA stabilizers could optimize mRNA vaccines ensuring they remain stable within the human body, leading to better vaccination outcomes. Additionally, understanding individual variations in RNA responses can drive the development of more targeted therapies, maximizing treatment efficacy and minimizing side effects.
"Future advancements in RNA stabilization research could fundamentally alter the approach to genomics and therapeutics."
In summary, the future directions in RNA stabilization research are crucial for enhancing existing methodologies and developing new therapeutic avenues. As technologies emerge and integrate with existing methods, the potential applications expand significantly. This requires sustained research efforts to harness the full capabilities of RNA stabilizers in various scientific domains.
Closure
In summary, the exploration of RNA stabilizers reveals their crucial role in biological and experimental contexts. This section emphasizes the significance of RNA stabilizers, from their mechanisms of action to their applications in research, therapeutics, and diagnostics. These stabilizers not only enhance the stability and integrity of RNA but also facilitate a multitude of scientific advancements.
Summation of Key Insights
RNA stabilizers have shown considerable promise across various fields. Their mechanism often involves direct chemical interactions with RNA, which can affect its structure and, subsequently, its stability. Understanding this mechanism is essential. This knowledge informs the choice and application of stabilizers in experiments and therapeutic scenarios.
Several types of stabilizers exist, including nucleotide analogues and chemically modified oligonucleotides. Each type has distinct benefits, tailored for specific uses in research or clinical settings. Moreover, applications span from molecular biology techniques to enhancing the reliability of diagnostic tools.
Future research should build upon these insights. There is a potential for RNA stabilizers to be at the forefront of innovative technologies, especially in areas like CRISPR and personalized medicine. The intersection of RNA stabilization with these advancements warrants more focused investigations.
Call for Continued Research
The field of RNA stabilizers is ripe for further exploration. A better understanding of their interactions with RNA can lead to more effective applications in therapeutics and diagnostics. Future research should aim to address the challenges currently faced, such as maintaining the delicate balance between stability and bioactivity.
Furthermore, assessing the long-term impacts of RNA stabilizers on cellular function is necessary. It is essential to determine potential side effects, such as toxicity or biocompatibility issues. Addressing these concerns will not only enhance our understanding but will also improve the application of these tools in clinical settings.
In summary, continued research is imperative for realizing the full potential of RNA stabilizers. Studying their properties, refining their use, and developing new formulations could significantly enhance both the practical and theoretical understanding of RNA processes in biology.