Protein Expression Purification: Techniques & Insights
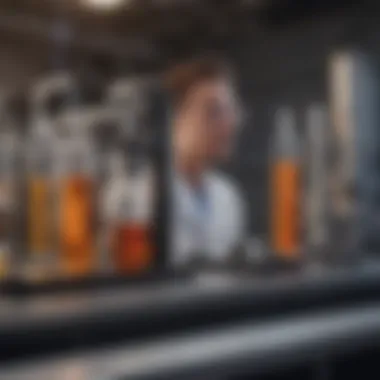
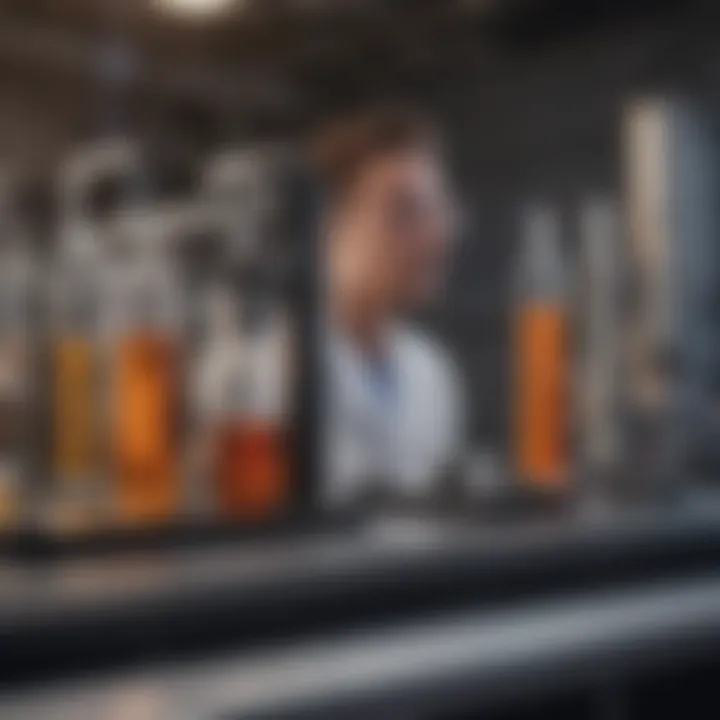
Intro
Protein purification is a crucial process in both research and industry, as it allows for the isolation and analysis of specific proteins. Given the complexity and diversity of proteins, developing effective purification techniques is essential. Understanding these methods can significantly enhance both scientific discovery and practical applications in fields like biotechnology and pharmaceuticals.
Protein expression purification involves several stages, from cell lysis to final product analysis. The methods employed can vary widely, depending on the protein of interest and the desired purity.
Despite its importance, protein purification is not without challenges. Factors such as protein stability, solubility, and post-translational modifications can impact the efficiency of purification processes. Therefore, a thorough overview of purification strategies provides valuable insights into the considerations needed for successful protein isolation.
This article aims to analyze current techniques used in protein expression purification, discussing their advantages, limitations, and best practices. By examining various methodologies, we prepare to explore the relevance of effective purification strategies in advancing science and technology.
Preface to Protein Expression Purification
Understanding protein expression purification is vital in numerous fields, such as biotechnology, pharmaceuticals, and research institutions. This article will explore the mechanisms, techniques, and considerations essential for effective protein purification. Protein purification serves as a foundation for numerous applications, helping to analyze enzymatic functions, produce therapeutic proteins, and facilitate structural biology studies.
Definition of Protein Expression
Protein expression refers to the process where a gene is transcribed into mRNA and then translated into a polypeptide chain, which folds into a functional protein. This process is fundamental in biology and biochemistry. It involves various stages that enable the synthesis of proteins in living cells.
The expression of proteins can occur in natural or modified organisms, often using recombinant DNA technology to introduce foreign genes into host cells. Common hosts include bacteria, yeast, and mammalian cells. Each system has its pros and cons, which influence the quality and quantity of the expressed proteins.
Importance of Protein Purification
Protein purification is a crucial step in the study and utilization of proteins. The significance of this process can be summarized in various aspects:
- Functional Analysis: Pure proteins allow for a more accurate assessment of their biological functions. This is essential for drug development and understanding metabolic pathways.
- Structural Studies: Purified proteins are critical for crystallization experiments, enabling scientists to study their three-dimensional structures through techniques such as X-ray crystallography or NMR spectroscopy.
- Therapeutic Proteins: The pharmaceutical industry relies on highly purified proteins, such as insulin, monoclonal antibodies, and enzymes, to ensure safety and efficacy in treatments.
- Research Advancements: High-quality purified proteins foster innovative experiments and research in various scientific domains, leading to breakthroughs in numerous fields.
Basic Concepts of Protein Expression
Understanding the basic concepts of protein expression is vital for anyone working in biotechnolgy, molecular biology or related fields. This knowledge lays the foundation for effective protein purification processes. Protein expression refers to the process by which cells generate proteins. This involves transcription of the genetic information followed by translation to assemble amino acids into functional protein chains. Mastering this topic allows researchers to manipulate and produce proteins with specific traits, crucial for diverse applications from therapeutics to industrial enzymes.
The Role of Host Cells
Host cells are pivotal in protein expression, acting as the factories where proteins are synthesized. Different types of host cells are used depending on the desired protein and its eventual application. Common choices include bacterial cells, yeast, and mammalian cells.
- Bacterial Cells:
- Yeast Cells:
- Mammalian Cells:
- Escherichia coli is frequently used due to its simplicity and fast growth.
- However, post-translational modifications may not be optimal.
- Saccharomyces cerevisiae serves as another popular option.
- These cells offer some eukaryotic post-translational modifications, making them suitable for certain proteins.
- HEK293 and CHO cells are examples that provide more accurate folding and modifications.
- They are preferred for complex proteins, particularly those intended for therapeutic uses.
Each type of host cell comes with specific advantages and disadvantages, and the choice of a host should align with the goals of the protein being produced. An optimal system can significantly enhance yield and functionality in downstream processes.
Expression Vectors and Gene Cloning
Expression vectors are essential tools in the quest for protein production. These are plasmids designed to facilitate the expression of a gene within a host cell. The selection of an appropriate vector is critical. It can greatly influence factors like protein yield, stability, and activity.
- Key Components of Expression Vectors:
- Promoter: Drives the expression of the gene.
- Selectible Marker: Allows for the identification of successfully transformed cells.
- Multiple Cloning Site: A unique region to insert the target gene.
Gene cloning is the process that inserts a fragment of DNA into the vector. This is done using restriction enzymes that cut specific DNA sequences. The cleavage creates complementary ends allowing for the insertion of target genes. Once the gene is cloned, it can be introduced into the chosen host.
In summary, comprehending the basic components of protein expression, host cell roles, and the mechanisms of gene cloning will significantly enhance any proteomics project. This knowledge not only informs the selection of suitable vectors and host cells but also aids in troubleshooting any potential issues that may arise during the protein expression process.
Purification Techniques Overview
Purification techniques are fundamental in the field of protein expression. They significantly influence the outcome of experiments and applications in both research and industry settings. Effective protein purification is critical for obtaining high-quality, functional proteins for enyzme assays, structural biology, and therapeutic applications. Selecting the appropriate purification method can enhance yield and purity while minimizing potential loss of activity.
Various methods exist, each with its strengths and weaknesses. Understanding these techniques helps researchers make informed decisions that align with their specific goals. The successful application of purification techniques often leads to streamlined workflows, reduced costs, and reproducible results in experimental methodologies.
Chromatography Techniques

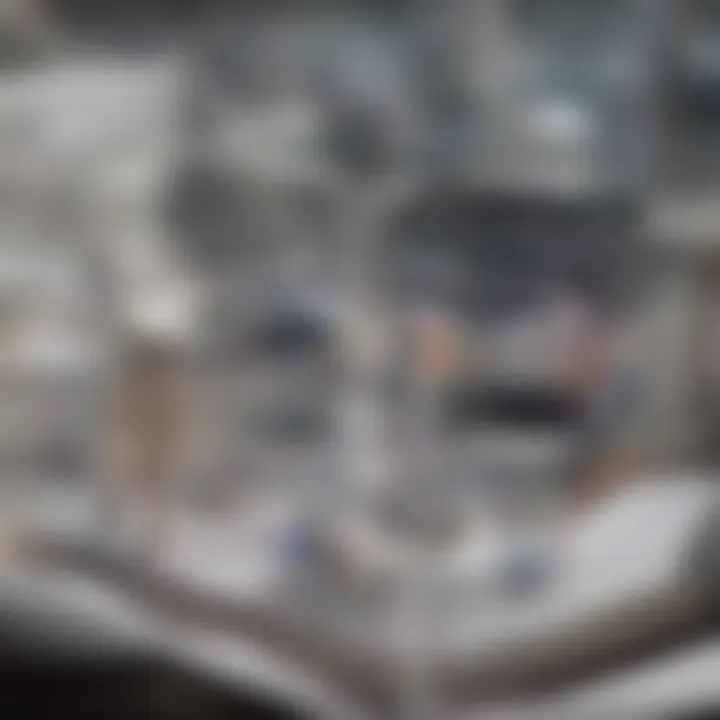
Affinity Chromatography
Affinity chromatography is a powerful technique used to isolate specific proteins based on their interactions with an immobilized ligand. This method capitalizes on the specific binding affinity of a protein to a ligand, enabling the purification of target proteins from complex mixtures. The key characteristic of affinity chromatography is its specificity, which allows for the selective capture of proteins of interest while excluding impurities.
This choice is beneficial because it typically results in higher purity levels, even with crude extracts. One unique feature of affinity chromatography is its ability to be optimized for various targets by selecting different ligands tailored to the protein being purified. However, it also has disadvantages, such as potential ligand leakage and changes in the protein's native state due to binding conditions.
Size Exclusion Chromatography
Size exclusion chromatography helps separate proteins based on their size, allowing for the effective separation of macromolecules in a solution. The principle behind this technique is straightforward: larger molecules pass through the column faster than smaller ones due to preferential flow paths. The key characteristic here is its gentle separation, which helps preserve protein functionalities by avoiding harsh conditions.
It is beneficial, especially when the goal is to remove small contaminants or buffer components from a protein solution. One unique feature of size exclusion chromatography is that it does not require specific binding interactions. However, a downside is its limited resolution for closely sized proteins, which may not be adequate for all purification tasks.
Ion Exchange Chromatography
Ion exchange chromatography relies on the interaction between charged proteins and an oppositely charged resin. This technique can effectively separate proteins based on their net charge at a given pH. A key characteristic that makes ion exchange chromatography a preferred choice is its versatility; different resins and conditions can be applied to tailor the purification process. This adaptability allows for precise control of purification outcomes.
The unique feature of this method is the ability to develop gradients that elute bound proteins selectively. Its advantages include the ability to achieve high resolution and scale-up for larger applications. A disadvantage is that success often depends on the correct choice of pH and ionic strength, which can complicate the initial setup of the purification strategy.
Precipitation Methods
Salt Precipitation
Salt precipitation is a widely used method that exploits the solubility properties of proteins. By adding saturated ammonium sulfate or another salt, proteins can precipitate out of solution based on their solubility limits. The key characteristic of salt precipitation is its simplicity and cost-effectiveness. This method is especially beneficial for concentrating proteins and can be combined with other purification techniques.
A unique feature is its ability to selectively precipitate proteins under varying conditions, allowing for the separation of proteins with similar properties. Advantages include minimal equipment needs and quick results. However, a potential downside is that salt precipitation may not yield highly purified protein, requiring subsequent purification steps.
Solvent Precipitation
Solvent precipitation involves adding organic solvents, such as ethanol or acetone, to solutions containing proteins. This technique results in protein denaturation and precipitation. A key characteristic is its effectiveness for concentrating proteins and removing lipids or other contaminants. This makes solvent precipitation a desirable choice when high purity is a secondary goal, and concentration is of primary interest.
The unique feature of solvent precipitation lies in its ability to be very efficient for certain types of proteins, particularly if they need to be isolated from non-polar impurities. While there are many advantages, such as low costs and ease of implementation, the main disadvantage includes potential loss of protein functionality due to denaturation.
Affinity Chromatography in Detail
Affinity chromatography represents a pivotal technique in the field of protein purification. It utilizes specific interactions between the protein of interest and a ligand attached to a solid support. This method stands out due to its high specificity, allowing for efficient separation of proteins based on their unique biochemical properties. Furthermore, this technique significantly enhances the yield and purity of the target protein, making it an essential approach in both research and industry settings.
Principles of Affinity Chromatography
The basic principle of affinity chromatography relies on the selective binding of a protein to a ligand. The ligand can be any molecule that the protein has an affinity for, such as antibodies, enzymes, or metal ions. When a mixture of proteins is passed through a column containing the immobilized ligand, only the desired protein will bind to it. Other proteins are washed away, and the target protein can be eluted using a solution that disrupts this interaction.
This technique is known for its efficiency and resolution. It can separate proteins even in complex mixtures, allowing researchers to achieve higher purity levels without extensive processing. Additionally, affinity chromatography can often be done under mild conditions, preserving the activity of sensitive proteins. However, selecting the right ligand is critical. If the ligand does not have a strong enough affinity for the target protein, the purification process will not be effective.
Types of Affinity Ligands
Several types of affinity ligands can be employed, depending on the characteristics of the target protein and the desired outcome.
- Antibodies: These are commonly used for immunoaffinity chromatography. The antibody binds specifically to the target protein, offering a high level of specificity and purification.
- His-tag: This is a histidine-based tag that can be used to bind proteins to metal ions like nickel or cobalt. It facilitates easy purification and can be removed later if needed.
- Enzyme-substrate interactions: This type uses the natural binding affinity between an enzyme and its substrate to isolate the enzyme from a complex mixture.
- Nucleic acid ligands: These can bind specifically to certain proteins, making them valuable for purifying DNA-binding proteins.
Size Exclusion Chromatography Explained
Size exclusion chromatography (SEC) is a vital technique in protein expression purification. It separates molecules based on their size. In this process, larger molecules move through the column faster than smaller ones, which can take longer due to their higher interaction with the porous matrix. This characteristic makes SEC very useful in a variety of applications where precise separation is essential.
Mechanism of Size Exclusion
The fundamental principle behind size exclusion chromatography relies on the gel filtration media, which contains pores that create a mesh-like structure. When a sample is injected into the column, the larger molecules cannot enter these pores and thus pass through more rapidly. In contrast, smaller molecules are able to enter the pores and navigate through the matrix's network, leading to a delay in their elution. Given this mechanism, SEC acts as a "molecular sieve," allowing for the separation of proteins from contaminants, aggregates, or by-products based on size alone.
Consider the following details that make SEC a preferred choice:
- Retains Activity: Since size exclusion does not involve any binding interactions, proteins retain their native conformation and biological activity.
- No Need for Specific Buffers: SEC can be performed in simple buffer systems, which simplifies the purification process.
- Scalability: The method can be easily scaled up for industrial applications, allowing for bulk purification.
Applications of Size Exclusion
Size exclusion chromatography has widespread applications across various fields, especially in biotechnology and pharmaceuticals. Some of its notable uses include:
- Purification of Proteins: SEC is commonly used to purify proteins, removing small contaminants effectively.
- Characterization of Biologics: Researchers utilize SEC to analyze the size distribution of biologics, providing essential insight into their structural properties.
- Buffer Exchange: This technique is efficient for exchanging buffer solutions, which is necessary when preparing proteins for further experiments.
- Elimination of Aggregates: SEC can successfully separate misfolded and aggregated proteins from properly folded ones, ensuring only functional proteins are used in applications.
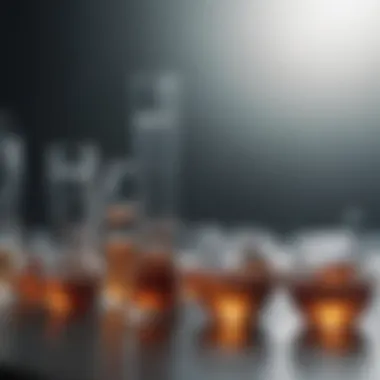
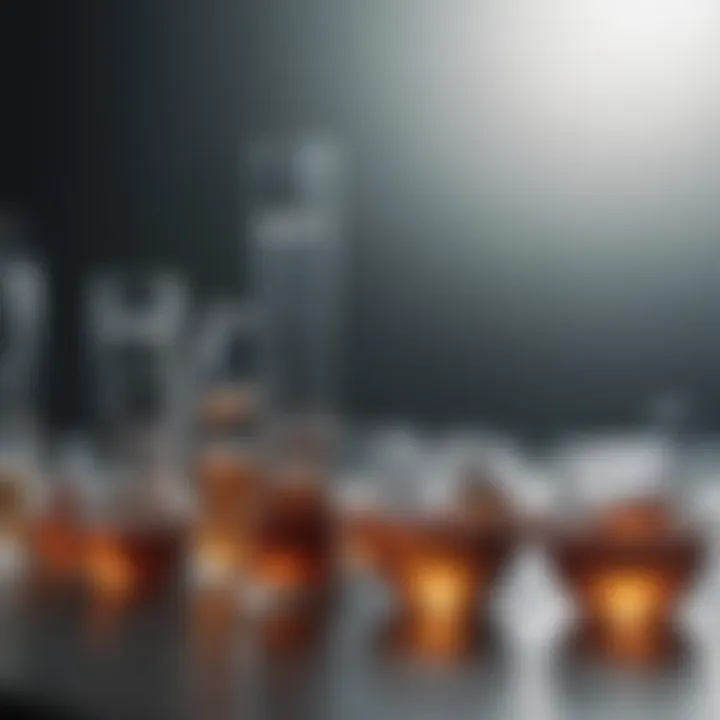
Size exclusion chromatography is critical for achieving high purity levels of proteins in research and industrial applications.
In summary, size exclusion chromatography is an essential method in the toolkit of researchers and industry professionals focusing on protein purification. Its straightforward mechanism, coupled with versatile applications, makes it invaluable for many scientific endeavors.
Ion Exchange Chromatography Fundamentals
Ion exchange chromatography is a crucial technique in the protein purification process. This method exploits the charge properties of biomolecules to separate them effectively. It is widely used because of its efficiency and ability to yield high purity protein. Understanding the principles of ion exchange chromatography is essential for optimizing purification protocols. This familiarity helps researchers mitigate potential issues that can arise during purification procedures, ensuring high-quality results.
Principles of Ion Exchange
The core principle of ion exchange chromatography revolves around the interaction between charged molecules and oppositely charged sites on a resin. The resin used can be either cationic or anionic, depending on whether the target proteins possess a positive or negative charge at a given pH. When a mixture containing various proteins is passed through the column, proteins with charges complementary to the resin will bind to it, while others will flow through.
After loading the mixture, the bound proteins can be eluted by changing the ionic strength or pH of the mobile phase. Increasing the salt concentration or adjusting the pH alters the electrostatic interactions, allowing for the selective release of specific proteins from the resin. This method permits a nuanced separation based on the net charge of the proteins present in the sample, providing significant control over purification outcomes.
Selecting the Right Ion Exchange Resin
Choosing the correct ion exchange resin is vital for achieving optimal separation efficiency. Factors to consider include:
- Type of Charged Groups: Depending on whether the target proteins are cationic or anionic, the corresponding resin type must be selected (cation resin or anion resin).
- Particle Size: Smaller particles provide a larger surface area for interactions but can lead to higher pressure in the system.
- Binding Capacity: Assessing the maximum amount of protein the resin can bind is essential for scalability and efficiency.
- pH Stability Range: The resin needs to maintain performance over the pH range relevant to binding conditions.
In the context of current proteomics applications, the right resin can greatly enhance protein recovery rates and reduce the purification time. Many researchers prefer resins with specific ligand types that match their target proteins' properties, further fine-tuning their purification process.
"Selecting an optimal ion exchange resin can drastically impact the success of the purification protocol. It is not merely a choice but a fundamental decision that guides the outcome."
In summary, grasping the fundamentals of ion exchange chromatography lays the groundwork needed to fully leverage its capabilities. By understanding the underlying principles and judiciously selecting resins, researchers can refine their purification workflows, leading to breakthroughs in various scientific domains.
Optimization Strategies in Protein Purification
Optimization strategies in protein purification are essential to achieving high yield and purity of target proteins. Ineffective purification can lead to low protein recovery and compromised functionality, ultimately impacting downstream applications. Therefore, understanding key optimization parameters ensures successful purification processes and provides both economic and scientific benefits.
Choosing Buffer Conditions
Buffer conditions play a critical role in protein stability and activity during purification. The choice of buffer must consider the pH and ionic strength, as these factors can significantly affect protein solubility and conformation. Different proteins exhibit distinctive behaviors under varying buffer conditions, leading to successful or unsuccessful purification outcomes.
- pH Levels: Selecting the right pH ensures that the protein maintains its native state. For many proteins, biological activity is highest at a physiological pH. However, certain proteins may require more acidic or alkaline conditions for optimal purification.
- Ionic Strength: This can influence the interaction between proteins and purification media, affecting adsorption and elution. Adjusting ionic strength during the purification process can help to minimize non-specific interactions and enhance the resolution of separation.
Using a buffer system that stabilizes target proteins while minimizing aggregation can greatly improve results. It is wise to conduct preliminary experiments to find the optimal buffer composition.
Temperature and Time Management
Temperature and time management are also crucial in purification processes. Both factors can impact protein stability, solubility, and activity. Careful regulation aids in maintaining the integrity of the protein during purification steps.
- Temperature Regulation: Most proteins are temperature-sensitive. Elevated temperatures can denature proteins or alter their active sites, reducing yield. A controlled environment often leads to preferable outcomes.
- Time Management: Overly extended purification procedures can lead to degradation or loss of function in sensitive proteins. Efficiently managing each purification step can enhance overall yield without compromising quality.
- Refrigeration: Using cold conditions can help suppress proteolytic activities from contaminating enzymes.
- Optimal Operating Temperatures: Determine an optimal working temperature for each purification step after consideration of the target proteinβs characteristics.
- Minimize Exposure: To protect against denaturation, limit the time proteins are exposed to purification conditions.
- Real-Time Analysis: Implementing in-line monitoring of purification processes assists in evaluating the proteinβs status throughout the workflow.
In summary, careful optimization of buffer conditions, temperature, and time during protein purification can lead to significant improvements in both purity and yield of target proteins, benefiting various applications in research and industry.
Troubleshooting Common Purification Issues
Protein purification can present several challenges. Troubleshooting common purification issues is essential for obtaining high-quality proteins. Recognizing these problems and knowing how to address them can greatly improve overall efficiency and yield in protein purification processes.
Identifying Purification Failures
Purification failures can occur at various stages. The most common indicators of a failed purification process include poor yield, inadequate purity, and unexpected results in downstream applications. To systematically identify these failures, one must evaluate the entire purification protocol. Here are specific points to consider:
- Yield Reduction: If the amount of protein obtained is significantly lower than expected, analyze each step of the purification process. Consider factors such as cell lysis efficiency, protein solubility, and binding capacity during chromatography.
- Purity Issues: Check for the presence of contaminants. One can use analytical techniques like SDS-PAGE or Western blotting to assess the purity of the protein. If impurities are observed, refining purification conditions or re-evaluating the choice of resin may be necessary.
- Functional Activity: If purified proteins do not exhibit expected biological activity, it may signal that they are misfolded or degraded during purification. Evaluate temperature control and buffer composition, as these could affect protein stability.
Mitigating Contaminants
Addressing the presence of contaminants is critical in protein purification. Contaminants can originate from various sources, such as the expression system, lysis buffers, or chromatography resins. Here are some strategies to reduce or eliminate these unwanted entities:
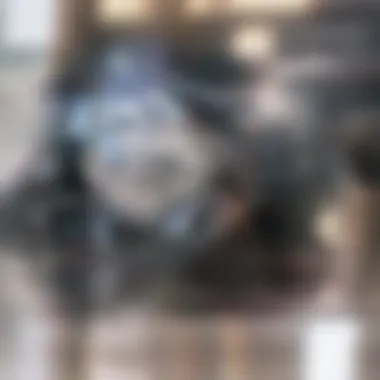
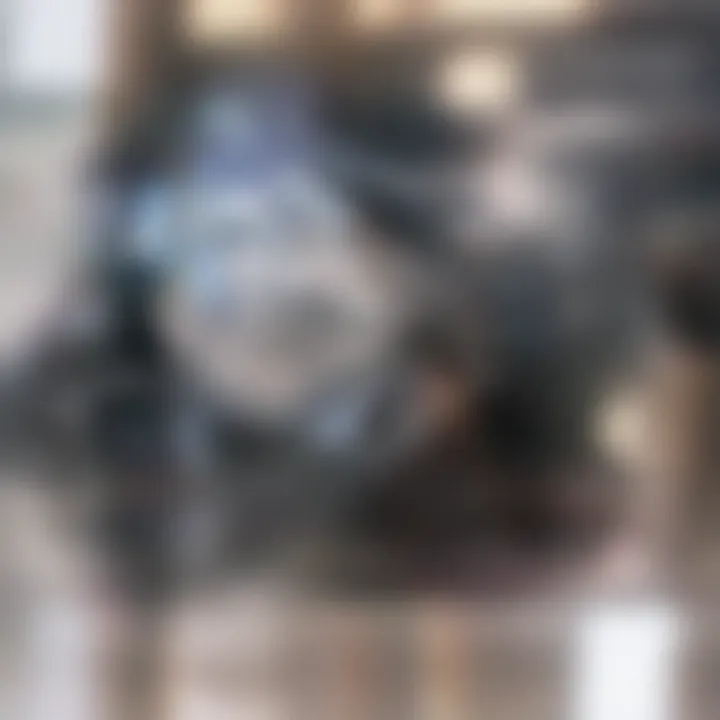
- Optimizing Lysis Conditions: Use appropriate buffers that facilitate selective lysis of cells while stabilizing the target protein. Protease inhibitors can prevent proteolytic degradation of proteins during extraction.
- Enhanced Washing Steps: Implement additional washing steps in affinity chromatography. This can help remove unbound contaminants more effectively, resulting in higher purity levels.
- Buffer Exchange Techniques: After initial purification, applying methods like dialysis or ultrafiltration can help remove salts, small molecules, or other contaminants, ensuring a cleaner final product.
Overall, meticulous troubleshooting and attention to detail can lead to successful purification outcomes. For those involved in research or industry, understanding these common issues and implementing strategic solutions is crucial to ensuring that proteins meet the necessary standards for downstream applications.
Recent Advances in Protein Purification Technologies
Recent developments in protein purification technologies have brought significant improvements in terms of efficiency, yield, and purity. These advancements are crucial for various applications in biotechnology and pharmaceuticals. As industries demand higher quality proteins for research and therapeutic purposes, staying updated with these innovations is essential for successful outcomes.
The latest techniques focus on automation, miniaturization, and high-throughput capacities. Incorporating these elements not only streamlines the purification process but also enhances reproducibility. Additionally, advances in instrumentation and software have allowed for better scalability and process monitoring. Researchers can now expect more consistent results, which is a vital aspect of experimental reliability and efficiency.
Importantly, advancements in purification methods often come with considerations regarding costs and resource utilization. While high-throughput systems may require significant initial investments, the long-term benefits in time and resource savings often justify these expenses. Adopting modern technologies can thus lead to more economical and environmentally friendly purification processes.
"The shift towards automation and high-throughput methods is transforming how we approach protein purification."
High-Throughput Purification Methods
High-throughput purification methods represent a transforming trend in the field of protein purification. This approach involves conducting numerous purification processes simultaneously, thereby significantly reducing the time required to obtain purified proteins. By employing specialized multi-well plates and custom-designed chromatography systems, researchers can achieve rapid isolation of proteins with minimal human intervention.
High-throughput systems allow researchers to evaluate multiple conditions during purification. Screening various buffer compositions, column types, and chromatographic conditions can be executed efficiently. As a result, the optimization of purification parameters is much quicker, facilitating the discovery of ideal conditions for specific proteins.
Furthermore, these methods enable the collection of larger data sets, which can inform future experiments. By leveraging statistical analysis and machine learning techniques, researchers can refine purification strategies based on empirical data, leading to even greater efficacy.
Automation and Robotics in Purification
The integration of automation and robotics in protein purification has marked a new era in laboratory practices. Automated platforms assist in the handling of samples, execution of protocols, and analysis of results, greatly reducing human errors that can affect the outcome of experiments.
Robotic systems can perform repetitive tasks, such as liquid handling and sample transfers, with high precision. This precision is critical in maintaining consistent conditions throughout the purification process, thus enhancing both reproducibility and reliability of results.
Utilizing these technologies not only reduces the workload on scientists but enhances their ability to focus on more complex aspects of research. Consequently, the incorporation of automation can lead to innovative research breakthroughs as researchers have more time for analysis and interpretation of data.
Applications of Purified Proteins
Purified proteins play a pivotal role in various fields, including biotechnology and pharmaceuticals. The ability to isolate and purify proteins is essential for their practical applications. These applications are not only crucial for advancing scientific research, but they also have significant implications for medical treatments and industrial processes.
Biotechnology and Drug Development
In biotechnology, purified proteins are used in a wide range of applications, especially in drug development. They serve as the fundamental building blocks for biopharmaceuticals, which are therapeutics produced using biological systems. These proteins can act as enzymes, antibodies, or hormones, among other functions.
- Enzymes: Many drug formulations include enzymes that catalyze specific biochemical reactions, enhancing the efficacy of the drugs. For example, recombinant DNA technology allows for the production of insulin, a hormone necessary for managing diabetes.
- Antibodies: Monoclonal antibodies are widely used in treating various diseases, including cancer and autoimmune disorders. These antibodies are engineered to target specific antigens, leading to more effective and personalized therapy.
- Therapeutic Proteins: Directly administering purified proteins to patients has become a common practice. This includes the use of growth factors and clotting factors in treating conditions like hemophilia.
Purified proteins are also crucial for diagnostics. Enzyme-linked immunosorbent assays (ELISAs) utilize purified proteins to detect specific antibodies or antigens in patient samples. This has become a mainstay in disease screening and monitoring.
Research and Academic Studies
In research and academic settings, purified proteins are vital for exploring fundamental biological processes. Proteins must be studied in purified form for detailed analysis of their structure and function. Scientists rely on these studies to understand cellular mechanisms and biochemical pathways.
- Protein Characterization: Techniques such as X-ray crystallography and nuclear magnetic resonance (NMR) spectroscopy require highly pure proteins to accurately determine the three-dimensional structure. Understanding protein structure is essential for grasping how they interact with other biomolecules.
- Functional Studies: Researchers often perform functional assays on purified proteins to investigate their activities and interactions with ligands or other proteins. Such studies can reveal insights into disease mechanisms and potential therapeutic targets.
- Biophysical Techniques: Methods such as circular dichroism or fluorescence spectroscopy assess protein folding and stability, which are critical for understanding protein behavior under various conditions such as temperature or pH variations.
Holistically, purified proteins contribute to life sciences far beyond practical applications. They are instrumental in basic research, leading to discoveries that drive innovation in health and industry.
"Purified proteins are at the heart of both therapeutic advancements and fundamental biological research, underlining their significance across various scientific fields."
Epilogue and Future Perspectives
The intricate process of protein expression purification plays a pivotal role in numerous fields. The methodologies explored throughout this article emphasize significant advances that have been made. Understanding these purification techniques is essential for both academic research and industrial applications. As we reach the conclusion of this discussion, it is important to reflect on the summary of key points and the emerging trends that shape the future of protein purification.
Summary of Key Points
The key points of protein purification techniques can be summarized as follows:
- Protein Expression is vital in biotechnology and pharmaceuticals. It is often the first step toward obtaining proteins of interest from various host systems.
- Purification Techniques such as affinity chromatography and size exclusion chromatography enable researchers to isolate proteins with high purity and yield.
- Optimization Strategies involve selecting appropriate buffers, managing temperature, and understanding the characteristics of the proteins being purified to enhance the overall efficiency of purification.
- Troubleshooting is crucial for overcoming common issues that can impede successful purification, including low yield and contamination.
- Recent Advances in technology contribute to the development of high-throughput and automated solutions that streamline protein purification processes.
These aspects underline the complexity and importance of effective protein purification in scientific endeavors.
Emerging Trends in Protein Purification
As the field of protein purification evolves, several trends are noteworthy:
- Integration of Automation: Automation reduces manual errors and increases throughput, leading to more efficient purification processes. Robotic systems are becoming commonplace in laboratories, allowing for simultaneous processing of multiple samples.
- Use of Artificial Intelligence: AI is being introduced for optimizing purification protocols. Machine learning algorithms can predict the best conditions based on previous experiments, thus improving outcomes.
- Development of New Materials: Research into novel resins and materials is ongoing. These advancements aim to enhance binding capacity and selectivity, addressing limitations of current chromatographic methods.
- Focus on Sustainability: The increasing awareness of environmental impact is driving efforts in making purification processes more sustainable. This includes reducing waste generation and utilizing greener solvents.
Keeping abreast of these trends is crucial for students, researchers, and professionals aiming to stay competitive in the field of biotechnology and pharmaceuticals.