Exploring Polymorphism in Crystalline Structures
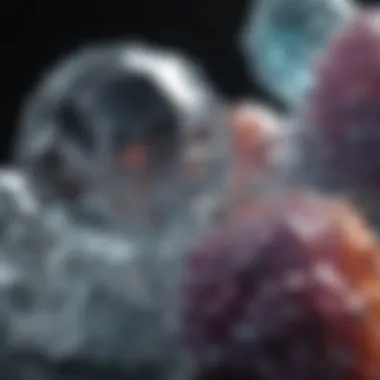
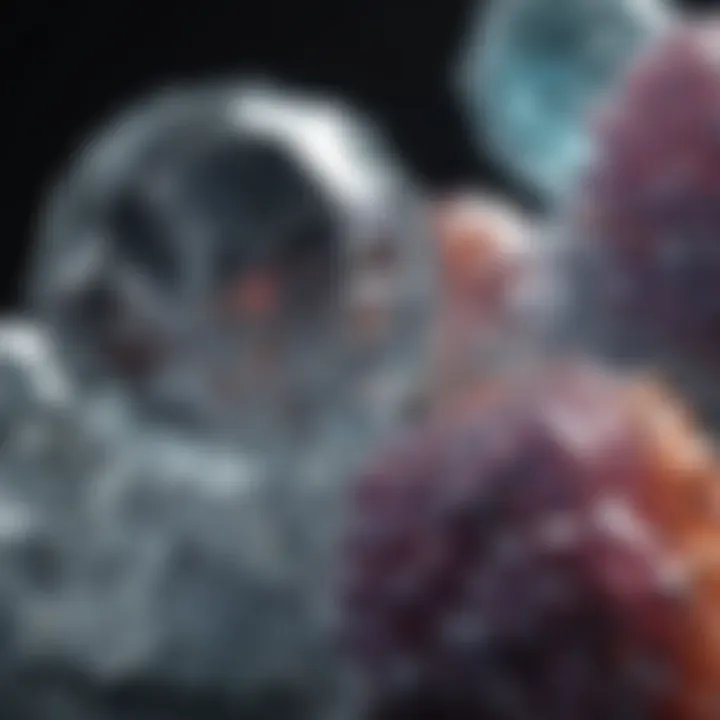
Intro
Polymorphism is the ability of a material, especially a crystalline solid, to exist in more than one form or crystal structure. This phenomenon holds substantial significance across multiple scientific disciplines and plays a crucial role in materials science. Understanding polymorphism not only informs the fundamental principles of crystallography but also helps to interpret the physical properties and applications of various materials. The exploration of polymorphism sheds light on how subtle variations in crystalline structures can lead to vast differences in functionality and performance.
This article aims to deliver a thorough investigation of polymorphism in crystals, emphasizing its types, underlying mechanisms, and the methodologies used to study it. An understanding of polymorphism is particularly relevant in fields such as pharmaceuticals where the specific crystal form can influence the solubility and bioavailability of drugs. Similarly, in materials engineering and geology, recognizing polymorphic forms aids in the development of new materials and understanding geological processes.
As research progresses, the significance of polymorphism continues to expand, revealing new insights that enhance our comprehension of structure-function relationships in crystalline materials. This exploration promises to be relevant for students, researchers, and professionals keen on uncovering the complexities of crystallization processes.
Research Overview
Summary of Key Findings
Polymorphism has shown to be a multifaceted subject with implications that are essential to various sectors. The key findings from the current research highlight that:
- Different crystalline forms of the same substance can have markedly different properties. For instance, certain polymorphs may demonstrate higher stability, solubility, or bioactivity.
- Environmental factors during crystallization influence polymorphic outcomes. Conditions such as temperature, pressure, and solvent type play critical roles in determining which crystal form predominates.
- Characterization techniques are vital for identifying polymorphs. Methods like X-ray diffraction, thermal analysis, and spectroscopy are indispensable in analyzing the structural properties of different polymorphic forms.
Methodologies Employed
The research utilizes a combination of theoretical and experimental methodologies. Crystal growth techniques and thermodynamic modeling are instrumental in predicting polymorphic transformations. Here are some specific methods used in the investigation:
- Differential Scanning Calorimetry (DSC): To assess the thermal properties of different polymorphs.
- X-ray Diffraction (XRD): Essential for determining the crystal structure and identifying the polymorphic form.
- Single Crystal X-ray Analysis: Provides detailed understanding of intermolecular interactions.
- Computational methods: Such as density functional theory (DFT) assist in predicting the stability of polymorphs based on molecular arrangements.
The combination of methods ensures clarity in understanding polymorphism and its implications across various fields, leading to better research outcomes.
In-Depth Analysis
Detailed Examination of Results
The examination of various studies showcasing polymorphism reveals intricate relationships between structure and properties. For instance, the polymorphism of bicalutamide, an anti-androgen medication, is documented with different practical implications for pharmaceutical formulation. The solubility of one form may differ considerably from another, impacting drug efficacy and patient outcomes. This emphasizes the need for precise control over crystallization conditions in pharmaceutical development.
Comparison with Previous Studies
Comparative analyses with historical studies demonstrate an evolving understanding of polymorphism. Past studies primarily focused on identifying polymorphic forms based on simple crystallization techniques. Modern investigations, however, incorporate advanced techniques and comprehensive modeling to predict polymorphic transitions and their properties more accurately. Notably, research such as that by J. C. B. George in 2015 provides a deeper framework to interpret these structural variations observed in drug compounds, offering a more holistic view.
"The ability to control polymorphism is a key component in materials design, impacting not just functionality but also end-user experience."
Understanding polymorphism lays the groundwork for numerous advancements in fields like drug development, material science, and even geology, making this a crucial area for ongoing study.
Intro to Polymorphism
Polymorphism is a critical phenomenon in the realm of crystallography that dictates how substances can exist in more than one form. This section sets the stage for understanding polymorphism by elucidating its significance in scientific inquiry and practical applications. As crystals can have various structural forms, knowing how these variations influence physical properties is paramount. The benefits of comprehending polymorphism extend across multiple fields, including pharmaceuticals, material science, and geology. Each unique crystalline form can exhibit distinct behaviors in solubility, stability, and reactivity.
One must consider the implications of polymorphism in drug formulation. For instance, different polymorphic forms of the same drug can lead to significant differences in effectiveness and absorption rates in the body. Therefore, recognizing the influence of crystal structure on such properties is crucial for developing more efficient medications.
The exploration of crystalline polymorphism also opens avenues for material innovation. As research advances, the capacity to tailor properties through polymorphic manipulation can lead to the invention of advanced materials with specific attributes, enhancing their applications. Consequently, polymorphism is not merely an academic subject but a significant factor influencing outcomes across various scientific disciplines.
Defining Crystalline Polymorphism
Crystalline polymorphism refers to the ability of a solid material to exist in more than one distinct crystalline structure. These structures differ geometrically due to variations in the arrangement of atoms or molecules within the crystal lattice. This nuanced definition underscores the essential characteristics that shape the properties of materials at the atomic level. Each polymorphic form may exhibit unique physical, thermal, or chemical properties, making polymorphism a key factor in materials science.
The occurrence of polymorphism can be influenced by a range of factors, including temperature, pressure, and the presence of impurities. As conditions change, the favored crystalline structure can shift, leading to different polymorphic forms.
Historical Context of Crystalline Studies
The study of crystalline polymorphism dates back centuries, with pivotal advancements marking its evolution. One of the earliest recorded observations of polymorphism was in the mid-19th century when scientists began systematically documenting variations in crystal forms. Research has since progressed, with significant contributions from various fields such as solid-state chemistry and mineralogy.
The development of advanced characterization techniques has further propelled the study of crystalline polymorphism. Innovations such as X-ray diffraction have provided deeper insights into the structural variations of crystals, leading to an expanding understanding of how these forms influence material properties. Today, researchers continue to investigate polymorphism, revealing its complexities and enhancing our grasp of solid-state phenomena.
The historical context highlights that this topic is not static but rather an evolving frontier of scientific investigation. As new methods and tools emerge, they unveil previously hidden aspects of polymorphic behavior, facilitating deeper insights for future research.
Mechanisms of Polymorphism
Understanding the mechanisms of polymorphism is crucial for a comprehensive analysis of crystalline structures. Polymorphism refers to the ability of a substance to exist in more than one form or crystal structure. The mechanisms involved dictate how different forms of crystals arise, showcasing the dynamic nature of solid-state materials. Investigating these mechanisms reveals important insights into stability, reactivity, and properties of the materials in question.
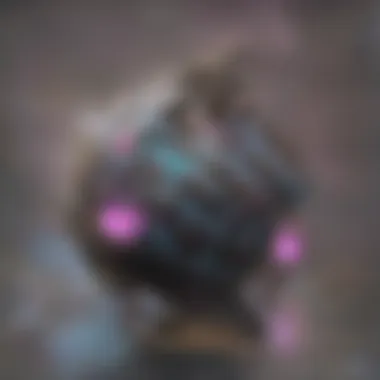
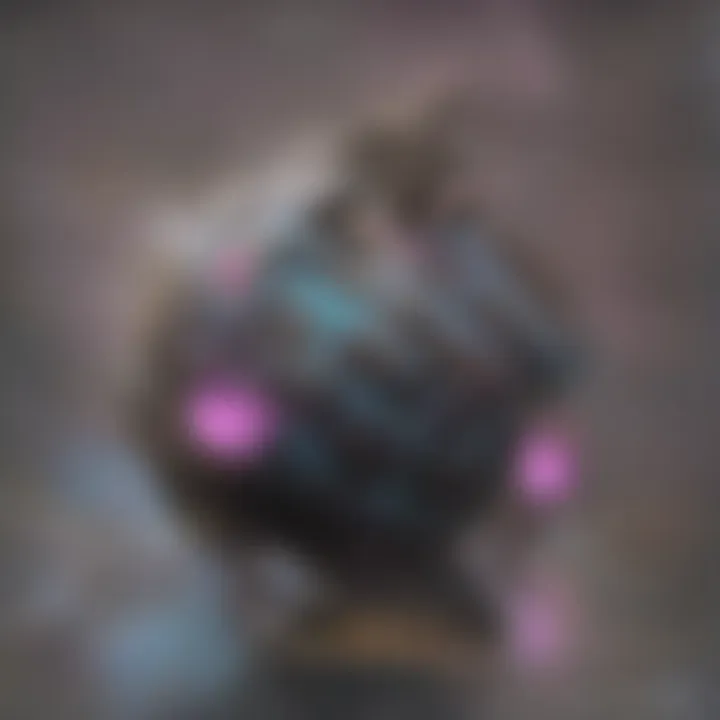
Studying polymorphism allows scientists and researchers to optimize the desired characteristics of materials, thereby enhancing their practical applications. When evaluating these mechanisms, two central aspects must be considered: thermodynamics and kinetics. Both areas significantly impact how a crystalline material develops and transforms over time, which in turn determines its use in various fields such as pharmaceuticals, materials science, and geology.
Thermodynamics of Crystallization
The thermodynamic aspects of crystallization play a vital role in understanding how different crystalline forms emerge. In essence, it addresses the energy changes that accompany phase transitions. The Gibbs free energy is a primary factor in determining the stability of a crystal form. When comparing two crystalline structures, the form with the lower Gibbs free energy is typically more stable.
Several parameters influence crystallization thermodynamics:
- Temperature: Higher temperatures typically increase the degree of disorder in a system, making it necessary to adjust temperature carefully to favor the desired polymorph.
- Pressure: Changes in pressure can stabilize certain crystalline forms over others. The stability of various allotropes can be phase-dependent and pressure-sensitive.
- Solvent interactions: In crystallization processes, the choice of solvent can greatly affect the polymorphic outcome. Certain solvents promote the formation of specific polymorphs due to their solvent-solute interactions.
One important concept in this area is the distinction between enantiotropic and monotropic systems. Enantiotropic systems can revert to another stable phase upon changing conditions, while monotropic systems can only exist in one form under all conditions. Understanding these distinctions is crucial for predicting and controlling the crystallization of materials.
Kinetic Factors Influencing Crystal Growth
Kinetics also plays an essential role in the emergence of polymorphic forms. While thermodynamics dictates what is stable at equilibrium, kinetic factors determine the pathways that lead to crystal growth. The rate of crystal formation can affect the final structure that is observed. Factors influencing the kinetics of crystal growth include:
- Nucleation: The initial formation of a crystal nucleus is a critical step in determining the eventual polymorph. A higher nucleation rate favors the formation of smaller crystals and can lead to non-equilibrium polymorphs.
- Growth rate: The speed at which particles attach to a growing crystal influences its development. Rapid attachment often produces irregular crystal shapes, while slower growth can allow for more ordered structures.
- Supersaturation: The level of supersaturation in a solution influences the likelihood of crystallization. Greater supersaturation may foster alternative polymorphs due to competing interactions in the solution.
"The interplay between thermodynamic stability and kinetic accessibility highlights the complexity of polymorphic behavior in crystals."
Types of Polymorphism
The concept of polymorphism in crystals plays a vital role in understanding material properties and their applications. The study of types of polymorphism reveals how different crystalline forms of a substance can influence physical characteristics, stability, and reactivity. Distinguishing between these forms can have significant implications, especially in fields like pharmaceuticals and materials science. Each type of polymorphism sheds light on the mechanisms that govern the solid-state behavior of compounds, guiding researchers in their quest for novel applications and improved product formulations.
Enantiotropic and Monotropic Polymorphism
Enantiotropic and monotropic polymorphism are two distinct categories, and understanding their differences is crucial. Enantiotropic polymorphism refers to systems where two polymorphic forms can interconvert reversibly with changes in temperature or pressure. The transition can involve different thermodynamic stabilities, which indicates that one form is stable at a higher temperature while another prevails at lower temperatures. Examples of enantiotropic systems can be seen in substances such as calcium carbonate, which can exist in both aragonite and calcite forms, dependent on the temperature of formation.
On the other hand, monotropic polymorphism describes systems where only one polymorphic form is stable under all conditions, while the other form is metastable. In these cases, the metastable form may persist for long periods but will ultimately convert to the stable form over time or under specific conditions. An example is the polymorphic variation observed in sucrose, where alpha- and beta-sucrose coexist at different stability levels.
The practical implications of understanding these two classes include predicting polymorphic behavior during processing and formulating products that capitalize on their unique properties. This is particularly relevant in fields like pharmaceuticals, where it can affect drug solubility and bioavailability.
Polymorphic Variation in Organic Compounds
Polymorphic variation is even more pronounced in organic compounds, which often exhibits a broader array of structures due to their complex molecular configurations. The variations can significantly affect physical properties such as melting points, solubility, and even taste or odor. This variation complicates formulation processes and requires careful management during drug development.
Several factors influence polymorphic forms in organic compounds, including:
- Temperature: Higher temperatures may destabilize certain forms.
- Pressure: Increased pressure can shift stability to less common structures.
- Solvent Effects: The presence of specific solvents during crystallization can favor the formation of one polymorph over another.
The importance of polymorphism in organic compounds can be seen through numerous case studies, such as the diverse forms of the antibiotic penicillin, which affect its stability and absorption in biological systems. Researchers must consider these variations when they design experiments or work on practical applications of these compounds.
By comprehensively studying these types of polymorphism, scientists can better optimize conditions for crystalline production, ensuring the desired form is achieved and maintained.
"Understanding polymorphism at these fundamental levels opens doors to innovation in product design and performance."
Measurement Techniques
Understanding the measurement techniques used in the study of polymorphism is crucial. These methods enable scientists to identify the structural differences in crystalline forms, which can have significant implications for various fields, including pharmaceuticals and material science. The accuracy and precision of these techniques directly affect the insights gained from research, influencing the subsequent applications of these materials.
X-ray Diffraction Analysis
X-ray diffraction analysis is among the most powerful tools for characterizing polymorphic forms. This technique relies on the interaction of X-rays with the ordered atomic arrangement in crystals. When X-rays hit a crystalline sample, they scatter in specific directions. By measuring the angles and intensities of this scattered radiation, researchers can obtain a diffraction pattern that reveals the crystal structure.
X-ray diffraction provides quantitative and qualitative data about the crystal lattice, such as unit cell dimensions, symmetry, and the presence of polymorphic forms.
Key advantages of using X-ray diffraction include:
- High resolution: Capable of detecting even minor variations in crystal structure.
- Non-destructive: Preserves the integrity of the sample for further analysis.
- Established methodology: Widely recognized and standardized in the scientific community.
But, it does come with challenges. Sample preparation must be meticulous, as impurities and other factors can distort the results. Furthermore, the interpretation of diffraction patterns requires advanced knowledge of crystallography.
Thermal Analysis Techniques
Thermal analysis techniques are invaluable when studying polymorphism. These methods assess how physical properties change with temperature, providing insights into polymorphic transitions, stability, and phase changes. Common thermal analysis methods include differential scanning calorimetry (DSC) and thermogravimetric analysis (TGA).

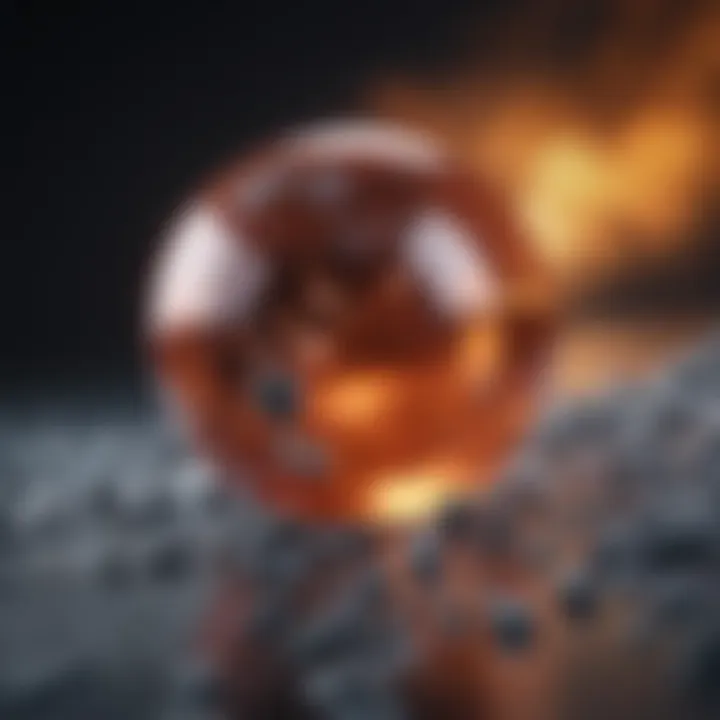
Differential scanning calorimetry enables researchers to measure the heat flow associated with transitions in materials as they are heated or cooled. This can reveal important information about melting points, solubility changes, and enthalpy variations between polymorphic forms.
Thermogravimetric analysis, on the other hand, measures weight changes in a material as a function of temperature. It can be particularly useful for understanding the thermal stability of different polymorphs.
Using thermal analysis has several benefits:
- Critical for stability studies: Helps in assessing which polymorphic form is more thermally stable under different conditions.
- Combination with other techniques: Often used in conjunction with X-ray diffraction for comprehensive analysis.
- Insightful for application: Essential for predicting how materials behave in real-world applications, especially in pharmaceuticals where stability is key.
Polymorphism in Pharmaceuticals
Polymorphism is crucial in the pharmaceutical industry as it directly influences drug formulation, efficacy, and stability. The phenomenon offers a variety of crystalline forms for the same compound, each with distinct properties. These variations can determine the solubility, stability, and bioavailability of drugs. Understanding polymorphism is essential for developing successful pharmaceutical products, as the chosen polymorph can significantly impact therapeutic outcomes.
The ability to design drugs with specific polymorphic forms adds complexity but also opportunities for innovation in drug development. Ensuring the correct polymorphic form is selected can enhance the performance of a medication while minimizing side effects and improving patient compliance. Moreover, characterization and selection of polymorphic forms can have implications in regulatory practices as well.
Impact on Drug Solubility and Bioavailability
The solubility of a drug is one of the most critical factors influencing its bioavailability. Polymorphic forms can have vastly different solubilities. For example, a more soluble form will dissolve easier in bodily fluids, leading to improved absorption in the gastrointestinal tract. Differences in solubility can arise due to crystal packing, lattice energy, and intermolecular forces present in different crystal forms.
Higher solubility enhances the therapeutic effect of a drug, ensuring that the required dose can reach systemic circulation effectively. This can be particularly significant in Class II and IV drugs, which have high permeability but low solubility.
Polymorphs can also affect the stability of drugs under various environmental conditions, potentially altering their shelf life and storage conditions. As a result, pharmaceutical companies must carefully study the polymorphic behavior of their compounds.
"Choosing the right polymorphic form can transform a poorly soluble drug into a successful treatment option."
Some examples of how polymorphism affects solubility include:
- Ibuprofen: Different polymorphs demonstrate varying solubility levels, influencing their use in formulations.
- Captopril: One polymorphic form of captopril exhibits higher solubility than another, leading to a choice in the development phase based on desired efficacy.
Case Studies of Polymorphic Drugs
Several drugs exemplify the impact of polymorphism on performance and market success. Each case highlights how different polymorphic forms can alter drug properties.
- Ritonavir: Initially marketed as a soft-gel capsule, the discovery of a more stable, less soluble polymorph led to formulation changes to ensure consistent bioavailability in clinical settings.
- Theophylline: This drug shows several polymorphs, each with distinct drug release profiles. Variants like the anhydrous and hydrated forms have been utilized in different formulations.
- Glibenclamide: This antidiabetic medication presents multiple polymorphs, including a more soluble form which has improved absorption characteristics.
Studying these cases highlights essential considerations for pharmaceutical development. Variants are not merely academic inventions; they are pivotal in ensuring that drugs remain effective, safe, and reliable across diverse patient populations.
Relevance in Material Science
Understanding polymorphism is essential for material science. The phenomena lead to tailored material properties that can improve performance in various applications. Variations in crystalline structures can drastically influence characteristics like strength, conductivity, and melting point. Therefore, a comprehensive grasp of polymorphism opens doors to innovation and refinement in material development.
Designing Advanced Materials
The design of advanced materials hinges on the ability to control polymorphism. Different polymorphic forms can offer unique properties suited for specific applications. In fields like electronics and photonics, the right crystalline structure can optimize efficiency and performance. Researchers focus on how to induce specific polymorphs during the synthesis phase. Methods such as controlled cooling, pressure application, and solvent adjustment are utilized to achieve desired structures. This precision in design can lead to the development of materials that might be lighter, stronger, or more responsive to stimuli than their counterparts.
Polymorphism in Nanomaterials
Nanomaterials present a unique arena for exploring polymorphism. The behavior of matter at the nanoscale often deviates from bulk properties. As such, polymorphic variations in nanoparticles can result in vastly different physical and chemical properties. For instance, the same compound may exhibit multiple crystalline forms, each having distinct optical or electronic behaviors when scaled to the nanosize.
Moreover, in the context of drug delivery, polymorphic nanomaterials can enhance bioavailability and targeted delivery. Understanding how polymorphism operates at the nanoscale allows for the optimization of materials used in various high-tech applications, from medicine to energy storage. This exploration not only contributes to a broader understanding of material properties but also to the invention of new technologies.
"In material science, polymorphism is not just a concept. It is the key to unlocking innovative solutions that cater to increasingly complex needs."
Geological and Environmental Implications
Understanding the geological and environmental implications of polymorphism is vital for several reasons. This area helps elucidate how variations in crystal structures impact geological processes and the stability of mineral forms found in nature. It also provides insights into how environmental conditions can alter these structures, which has far-reaching impacts on both geology and industry.
Polymorphism in Earth Minerals
Polymorphism in earth minerals demonstrates how a single compound can crystallize in different ways. For instance, carbon can be found as both diamond and graphite. Each form possesses unique physical properties, such as hardness and electrical conductivity. This polymorphism has significant implications for both the economy and scientific understanding of mineral resources.
- Economic Relevance: Different forms of minerals may have distinct applications. Diamonds, known for their hardness, are utilized in cutting tools and jewelry, while graphite is essential in batteries and lubricants.
- Indicator of Geological Processes: The study of polymorphic minerals can reveal information about the conditions under which they formed, such as temperature and pressure. For example, high-pressure conditions may facilitate the formation of diamond, while lower pressures favor graphite.
In addition to these aspects, the mnemonic value of polymorphic minerals in identifying geological phenomena cannot be understated. They assist geologists in deciphering the history of rock formation and tectonic activity in a given region, which is crucial for resource exploration and environmental assessment.
Environmental Factors Contributing to Polymorphism
Environmental factors play a critical role in influencing polymorphic behavior. Temperature, pressure, and the presence of solvents or other chemicals can lead to different crystalline forms of the same substance. Understanding these factors is important for multiple reasons:
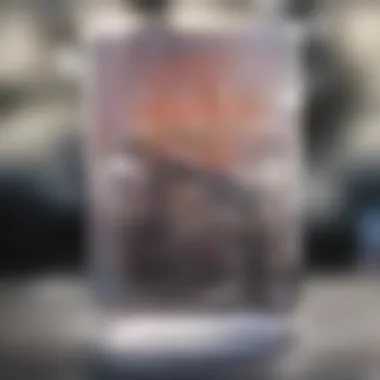
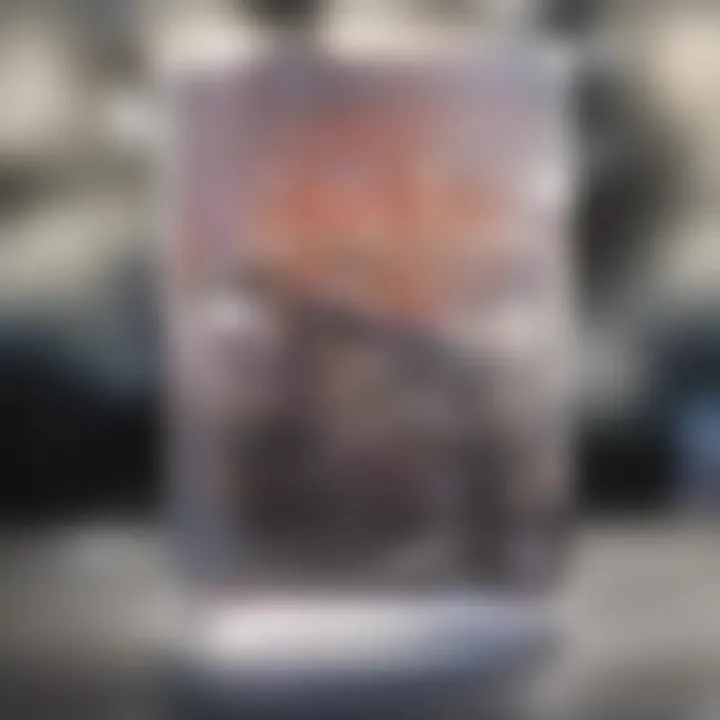
- Variability in Natural Conditions: Conditions such as temperature changes and chemical interactions can result in phase transformations. This variability can drastically affect the stability of mineral forms.
- Impact on Mineral Resources: Mining and extraction processes must account for the polymorphic nature of minerals. The presence of different variants can affect purity and yield during extraction.
- Sustainability Considerations: Environmental changes driven by human activities, such as climate change, may influence the development of new polymorphic forms, potentially leading to unforeseen ecological consequences.
"The understanding of polymorphism in minerals not only broadens our scientific knowledge but also aids in the responsible management of Earth's resources."
In summary, the geological and environmental implications of polymorphism are extensive. From analyzing mineral deposits to understanding the broader effects of environmental changes, this sector opens up valuable perspectives for research and application in numerous fields.
Computational Approaches in Polymorphism Studies
The exploration of crystalline polymorphism has greatly benefited from computational approaches. These methodologies enable researchers to simulate and predict the behavior of materials at the molecular level, thus providing insights that can be difficult to obtain from experimental methods alone. The application of computational techniques has become essential in understanding how different polymorphic forms arise and their implications on physical properties.
Molecular Dynamics Simulations
Molecular dynamics (MD) simulations are a prominent computational tool used in the study of polymorphism. In this method, atoms and molecules are treated as classical particles that are allowed to interact over time according to physical laws. By applying relevant force fields, researchers can observe how different configurations of molecules might evolve during the crystallization process.
The key advantages of MD simulations include:
- Realistic Time Frames: MD can simulate processes over time scales that are relevant to crystal growth.
- Detailed Interactions: Researchers obtain insights into the molecular interactions that govern polymorphic transitions.
- Predictive Power: Simulations can predict which polymorphic forms may occur under specific conditions, potentially guiding experimental designs.
However, there are some challenges present in the use of MD simulations. Computational resources can be a limiting factor, especially when simulating large systems over longer time spans. Additionally, force field accuracy significantly influences the reliability of the results, necessitating careful selection and validation of parameters used in the simulations.
Density Functional Theory Applications
Density functional theory (DFT) is another essential computational approach for studying polymorphism. Unlike molecular dynamics, DFT focuses on the electronic structure of materials to predict various properties. This method uses quantum mechanical principles to calculate properties such as total energy, electron density, and structural stability based on the arrangement of atoms within a crystal.
The benefits of utilizing DFT include:
- High Accuracy: DFT offers precise calculations at the electronic level, leading to an improved understanding of bonding and stability in different polymorphs.
- Energy Landscape Exploration: DFT helps in mapping the energy landscape associated with different crystalline forms.
- Predictive Analysis: Researchers can assess the stability of specific polymorphs in various environmental conditions, which is crucial for materials design.
Nevertheless, DFT also has limitations. The computational intensity of DFT calculations renders them expensive, particularly for large systems or complex materials. It also requires an understanding of different approximations which may affect the calculations' reliability.
"Computational approaches provide significant leverage in understanding polymorphism, guiding both theoretical and experimental investigations."
In summary, computational approaches, particularly molecular dynamics simulations and density functional theory, are vital for advancing our understanding of crystalline polymorphism. They enhance our capability to predict and manipulate the properties of materials, ultimately leading to more effective applications in various fields.
Challenges in Polymorphism Research
The study of polymorphism in crystals presents unique challenges that significantly impact research outcomes and applications. Understanding these challenges is essential for advancing knowledge in fields such as materials science, pharmacology, and geology. A deeper exploration of such issues can also guide researchers in developing improved methodologies and achieving reproducible results.
Reproducibility in Polymorphic Studies
Reproducibility is a cornerstone of scientific research. In the context of polymorphism, the ability to reproduce results adds validity to findings. However, varying crystal structures and changing environmental conditions can lead to significant discrepancies in results when experiments are repeated. Factors such as temperature, pressure, and the presence of solvents can all influence the crystallization process. This variability can create inconsistent results, impacting the reliability of studies.
Researchers face the challenge of establishing standardized protocols that account for these variables. Understanding the influence of environmental conditions on crystallization and ensuring uniformity in sample preparation are crucial. Enhanced focus on these aspects will likely improve reliability in polymorphic studies.
Technological Limitations and Advancements
The field of polymorphism research is constantly evolving. Yet, it is not without its technological limitations. Traditional techniques, while effective, may struggle to provide detailed insights into the complex behaviors of polymorphs. For instance, X-ray diffraction often requires large crystals, which can be difficult to obtain.
Advancements in technology may offer solutions to these hurdles. Techniques like high-throughput screening and advanced spectroscopy have begun to emerge, providing researchers with new methods to study polymorphs more efficiently and accurately. Utilizing tools such as cryo-electron microscopy also enhances the ability to analyze crystalline structures at a molecular level by bypassing some limitations of previous methods.
Despite these advancements, challenges remain. Investing in technology that allows for real-time monitoring of crystallization processes could further improve understanding and enable more precise control over the formation of polymorphs.
Epilogue and Future Directions
The study of polymorphism in crystals plays a crucial role in various scientific fields. It enhances our understanding of materials and their behaviors under different conditions. Recognizing how polymorphism affects properties such as stability, solubility, and mechanical strength is vital in areas like pharmaceuticals and materials science. As we conclude this comprehensive examination, it is important to reflect on the key findings and consider the future scope of research in this domain.
Summarizing Key Findings
Through detailed analysis, we have highlighted several important aspects of polymorphism in crystals:
- Diverse Manifestations: We have explored the different types of polymorphism, notably enantiotropic and monotropic forms, which demonstrate how the same chemical composition can lead to distinct structural arrangements.
- Impact on Properties: The implications of polymorphism extend to critical properties of materials. In pharmaceuticals, polymorphic forms significantly influence drug solubility and bioavailability.
- Measurement Techniques: The effectiveness of techniques like X-ray diffraction and thermal analysis in studying polymorphs ensures that researchers can accurately analyze crystalline structures.
- Computational Methods: As computational approaches such as molecular dynamics simulations and density functional theory become more advanced, they enhance our understanding of polymorphic behavior at a molecular level.
This summary encapsulates the multifaceted nature of the subject, highlighting the importance of continued exploration in this area.
Implications for Future Research
The future of research in polymorphism is promising and encourages innovative exploration. Some potential pathways include:
- Advanced Characterization Techniques: Developing new methods to characterize polymorphs can provide deeper insights into their stability and transitions. This includes more refined analytical tools that integrate both theoretical and experimental approaches.
- Cross-disciplinary Approaches: Collaboration between chemists, materials scientists, geologists, and pharmacologists can yield new discoveries. Such interdisciplinary strategies will enrich our understanding of polymorphism and its applications.
- Eco-Friendly Considerations: Research could focus on green chemistry practices when synthesizing compounds with specific polymorphic forms, helping reduce environmental impact.
- Real-World Applications: Investigating how polymorphism can be utilized in technology, such as in drug design and materials development, will bridge the gap between theory and practical use.
In summary, the study of crystalline polymorphism opens a broad array of research opportunities. It beckons a careful attention to both current findings and future advancements, paving the way for ongoing scholarly inquiry.