Poly L Lactic Acid: A Comprehensive Overview

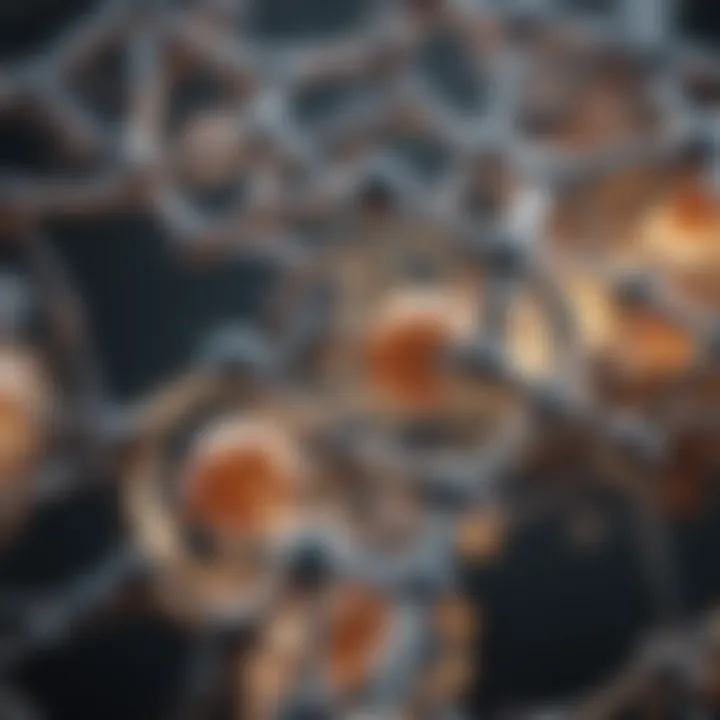
Intro
Poly L lactic acid (PLA) has emerged as a crucial material in a variety of fields. This biodegradable polymer, derived from renewable resources, plays a significant role in discussions surrounding sustainability. Its increasing adoption reflects a shift towards eco-friendly alternatives to traditional plastics, which pose serious environmental challenges. Understanding PLA involves exploring its chemical structure, unique properties, and the synthesis methods used to create it.
One of the notable aspects of PLA is its capacity to decompose naturally, minimizing environmental impact. This overview aims to dissect the implications of PLA across various industries, focusing on its practical applications and future potential. By examining PLA in detail, we gain insights into its benefits and limitations.
Research Overview
Summary of Key Findings
The study of PLA reveals multiple advantages, such as its biocompatibility and usability in diverse applications ranging from medical devices to packaging solutions. Key findings highlight that PLA performs well in applications where strength and flexibility are needed. It is also important to note that the source of lactic acid, often derived from corn starch or sugarcane, contributes to the sustainability of the production process.
Methodologies Employed
Researchers have employed various methodologies to synthesize and analyze PLA. Common methods include:
- Ring-opening polymerization: This technique is preferred for its effectiveness and ability to create high molecular weight polymers.
- Batch production: Often used in industrial settings, this method allows for the large-scale preparation of PLA.
- Solution casting: A lab technique useful for creating thin films of PLA used in packaging.
Studies frequently incorporate advanced characterization techniques such as spectroscopy and chromatography to assess the polymer's properties and performance metrics.
In-Depth Analysis
Detailed Examination of Results
The effectiveness of PLA in various applications is rooted in its thermal and mechanical properties. For instance, PLA exhibits a glass transition temperature around 60Β°C, making it suitable for many applications that require material stability at room temperature. Additionally, its tensile strength makes it comparable to conventional plastics, while its modulus of elasticity aligns well for items requiring flexibility.
Comparison with Previous Studies
Comparative studies indicate that while PLA shows promise, it also faces challenges. Previous research often points out degradation rates that can be slower than expected in certain environments. This contrasts with other biopolymers, such as polyhydroxyalkanoates (PHA), which may decompose more quickly in specific scenarios. Recent innovations are focused on enhancing PLA's degradation features and thermal stability to broaden its applicability.
"The future of biodegradable materials like PLA lies not only in their development but also in the understanding of their environmental implications."
Foreword to Poly Lactic Acid
The study of poly L lactic acid (PLA) has gained momentum in recent years, driven by an increased demand for sustainable materials across various sectors. PLA, a biodegradable polymer derived from renewable resources, offers numerous benefits. Recognizing its potential is vital for students, researchers, educators, and professionals alike, as it aligns with the global push towards environmentally friendly alternatives. An understanding of PLA is not just an academic exercise; it has real-world applications that could reshape how industries approach material usage.
Definition of Poly Lactic Acid
Poly L lactic acid (PLA) is a thermoplastic aliphatic polyester formed from L-lactic acid monomers. Its structure allows for a range of mechanical properties, making it suitable for diverse applications. As a biopolymer, PLA originates from renewable resources such as corn starch or sugarcane. The polymerization process of lactic acid leads to various forms of PLA, each adaptable for specific uses, such as in packaging or biomedical devices. The ability to decompose back into natural substances under appropriate conditions adds significant value to this material.
Historical Context
The origins of PLA can be traced back to the early 20th century, when chemists first synthesized lactic acid. However, PLA only began to gain traction as a commercially viable product in the late 1980s and early 1990s. The development of fermentation methods for lactic acid production from biomass marked a turning point. This innovation coincided with rising awareness of plastic pollution and a push for biodegradable materials. Today, PLA is widely used in various applications, from disposable items to medical devices, showcasing its versatility and potential influence on sustainable practices.
"Understanding the historical backdrop of PLA helps appreciate its evolution as a resource-efficient alternative in material science."
Chemical Structure of Poly Lactic Acid
Understanding the chemical structure of Poly L Lactic Acid (PLA) is fundamental to grasping its properties and functions. The structure outlines not only its composition but also the potential it harbors for various applications. PLA is primarily formed from L-lactic acid monomers, which are derived from renewable sources such as corn starch or sugarcane. This renewable origin is significant, as it emphasizes the sustainability aspect of PLA, making it an attractive alternative to petroleum-based plastics.
Monomer and Polymer Formation
Lactic acid exists in two isomeric forms: L-lactic acid and D-lactic acid. The L-isomer is the focus for PLA, as it provides the desired properties. The formation of PLA through polymerization involves linking these L-lactic acid monomers through various chemical methods. Typically, two methods are used for this purpose: ring-opening polymerization and condensation polymerization.
Ring-Opening Polymerization involves the use of cyclic lactide, which opens up and forms long chains of PLA. This method allows for a high degree of control over molecular weight and polymer architecture, which can be tailored for specific applications.
On the other hand, Condensation Polymerization generates PLA by removing a small molecule, such as water, as the L-lactic acid monomers are linked. Although it is simpler, this method might yield a product with lower molecular weight and might not provide the same consistency in properties.
Crystallinity and Molecular Weight
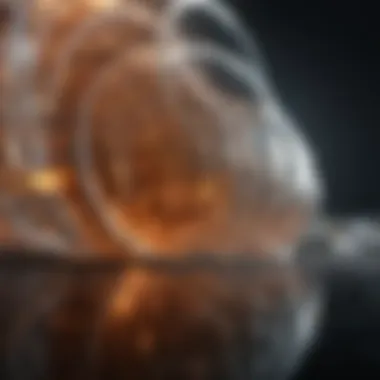
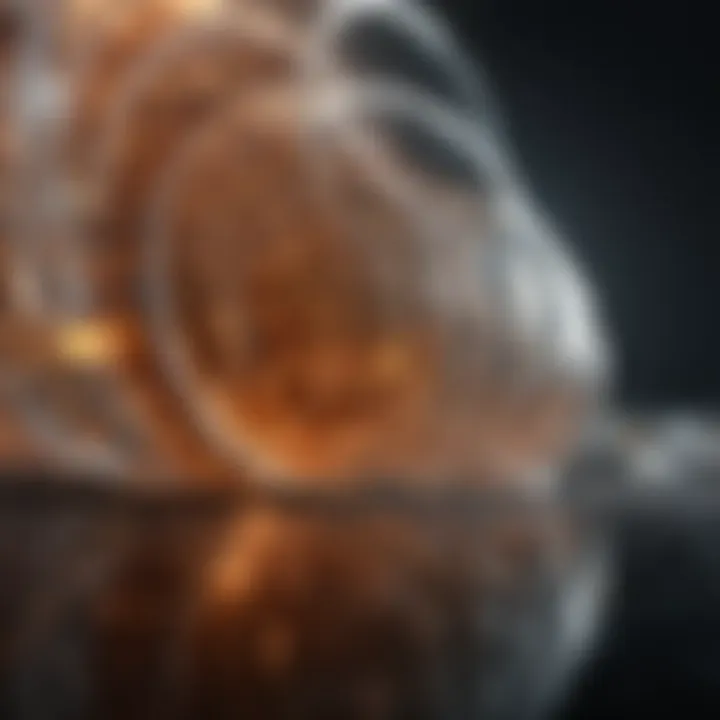
Crystallinity in PLA refers to how ordered the molecular structure is. PLA can exist in both amorphous and crystalline forms, and the balance between these two states is crucial. Higher crystallinity generally leads to improved mechanical properties and thermal stability, which are desirable for many applications. In contrast, the amorphous form tends to have better transparency and flexibility, making it suitable for packaging.
Molecular weight is another critical factor that influences the performance of PLA. Higher molecular weight correlates with better mechanical strength and thermal resistance. This means that as PLA is synthesized with care to maintain a high molecular weight, its application can expand into more demanding fields, such as biomedical devices.
In summary, the chemical structure of PLA dictates its functionalities and is essential in determining its suitability for various uses in several industries, from medical applications to sustainable packaging solutions. Understanding these structural characteristics is paramount for both researchers and manufacturers aiming to innovate using PLA.
Properties of Poly Lactic Acid
Understanding the properties of poly L lactic acid (PLA) is crucial for comprehending its performance in various applications. This section outlines the key thermal, mechanical, and biodegradability attributes, which collectively illustrate its benefits and considerations in todayβs material science landscape. These properties significantly dictate how PLA interacts with different environments and how it can be applied efficiently and sustainably.
Thermal Properties
The thermal properties of PLA are essential for its processing and application. PLA has a glass transition temperature ranging from 50 to 65Β°C, making it suitable for applications involving moderate heat. Its melting temperature can reach up to 180Β°C, which influences how the polymer can be molded and shaped.
Heat resistance is a vital performance metric, especially in packaging and medical applications. For example, products must withstand various temperature conditions without compromising structural integrity. PLAβs thermal stability allows for effective use in applications requiring autoclaving, such as surgical sutures.
Additionally, the thermal degradation of PLA begins around 250Β°C. Beyond this, the polymer starts to lose its mechanical strength and can decompose. Notably, researchers focus on how blending PLA with other polymers can enhance its thermal resistance, expanding its usability in more demanding scenarios.
Mechanical Properties
Mechanical properties determine how materials perform under stress and strain. PLA exhibits impressive tensile strength, which can be comparable to that of polystyrene and polyethylene. This characteristic makes it ideal for applications where strength is required, such as in 3D printing and automotive parts.
The tensile modulus of PLA is generally rigid, promoting durability in structural applications but potentially compromising flexibility. The balance of strength and flexibility is an important consideration for designers. Incorporating plasticizers or copolymerizing with other materials can adjust properties to match specific needs.
Moreover, the impact resistance of PLA is lower than that of some other plastics. This is a limitation in certain industries, necessitating modifications or hybrid composites for increased resilience.
Biodegradability and Environmental Impact
One of the most significant advantages of PLA is its biodegradability, a quality that aligns with current ecological objectives. PLA can decompose under industrial composting conditions, breaking down into carbon dioxide and water in as little as 60 days. This biodegradation process presents a less harmful alternative to traditional plastics, which often persist in the environment for centuries.
However, the level of biodegradability depends on several factors, including temperature, humidity, and microbial activity. Notably, PLA does not degrade effectively in marine environments, highlighting the need for careful waste management.
Another related consideration is the environmental impact during production. PLA is derived from renewable resources like corn starch or sugarcane, presenting a lower carbon footprint compared to petroleum-based plastics. However, concerns about land use for biofuels persist, as they can compete with food production.
In summary, while PLA offers several environmental advantages, responsible sourcing and comprehensive waste management strategies are essential to minimize unintended consequences.
Synthesis of Poly Lactic Acid
The synthesis of poly L lactic acid is crucial in understanding its utility and value in various applications. This process not only defines the polymer's characteristics but also influences its performance in real-world conditions. Effective synthesis methods determine the properties and potential applications of PLA, making this section particularly important for students, researchers, educators, and professionals engaged in material science and sustainable technologies.
Lactic Acid Fermentation
Lactic acid fermentation is a biological process that converts sugars into lactic acid using microorganisms such as bacteria. This method is vital for producing the lactic acid needed to synthesize poly L lactic acid. There are several key advantages to this fermentation process:
- Renewable Source: The utilization of carbohydrates from plant sources like corn, sugarcane, or beets ensures that the feedstock is renewable.
- Lower Environmental Impact: Compared to petrochemical processes, lactic acid fermentation has a reduced carbon footprint.
- Biological Control: The fermentation process involves specific microorganisms, allowing precise control over the production quality and yield.
To achieve PLA, the fermentation process can be split into several stages:
- Preparation of the Substrate: Feedstock is often pre-treated to extract fermentable sugars.
- Fermentation Process: The fermentation is carried out under controlled conditions, optimizing parameters like pH, temperature, and oxygen availability.
- Lactic Acid Recovery: After fermentation, lactic acid is extracted and purified for further processing.
Polymerization Methods
Once lactic acid is produced, the next step in synthesizing poly L lactic acid is polymerization. This process involves converting lactic acid monomers into a polymer chain, resulting in PLA. Several polymerization techniques can be utilized, each influencing the characteristics of the final product:
- Ring-Opening Polymerization (ROP): This method involves opening lactide, the cyclic dimer of lactic acid, to form a polymer. ROP typically offers a higher molecular weight PLA, which translates to better mechanical properties and thermal resistance.
- Condensation Polymerization: In this technique, lactic acid molecules react to form PLA while releasing water as a byproduct. While simpler, it often results in lower molecular weight polymers and can take longer, requiring careful control of reaction conditions.
The choice of polymerization method will have significant implications for PLA's performance in applications ranging from medical devices to packaging solutions. Understanding the intricacies of these techniques is fundamental for ongoing research and development in the field.
The synthesis of PLA through lactic acid fermentation and polymerization reflects both innovation and sustainability, with far-reaching implications for material science and environmental impact.
Applications of Poly Lactic Acid
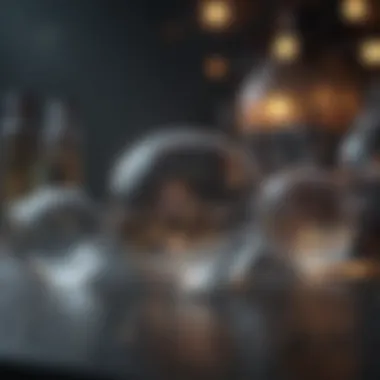
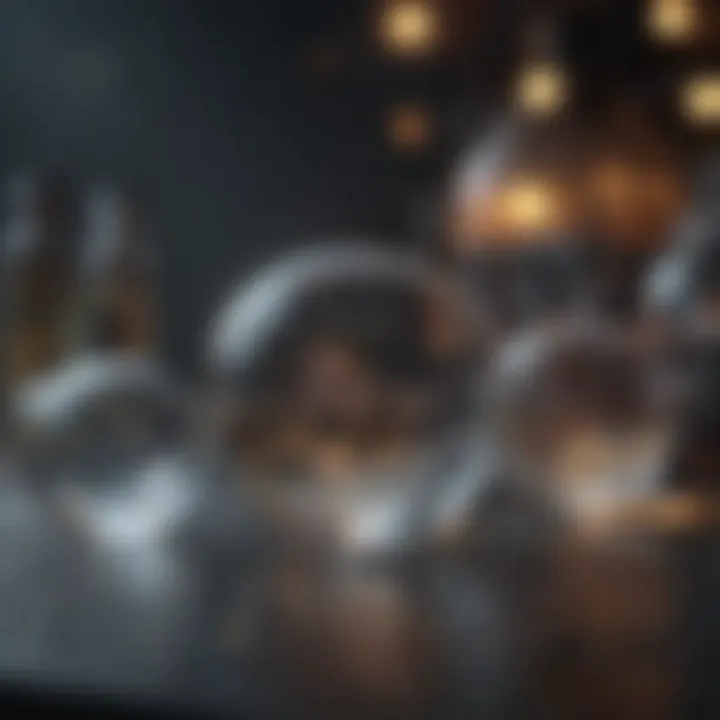
The applications of poly L lactic acid (PLA) highlight its significance in modern materials science. PLA serves diverse roles across industries, driven by its advantageous properties such as biodegradability and compatibility with various applications. This section delves into the key applications of PLA, providing insight into its uses and the benefits it brings.
Medical Use Cases
Surgical sutures
Surgical sutures made from PLA play an important role in medical procedures. These sutures are designed to be absorbed by the body over time. This characteristic reduces the need for a second surgery to remove traditional stitches. A key advantage of PLA sutures is their biocompatibility. The body generally accepts them well, leading to minimal inflammation.
The unique feature of PLA surgical sutures is their gradual degradation. As the sutures dissolve, they provide continuous support to the healing tissue. However, this gradual loss of strength must be considered. While they are beneficial for many surgical applications, certain procedures may still require non-degradable options.
Drug delivery systems
Drug delivery systems utilizing PLA showcase another significant application. They enable controlled release of medication over an extended period. This controlled delivery can improve treatment efficacy and patient compliance. The main characteristic of PLA in this context is its ability to be engineered for specific release rates based on the drug and desired outcome.
A unique aspect of PLA drug delivery systems is their versatility. They can be formulated into various shapes and sizes, such as microspheres or films, to match different therapeutic needs. One challenge, however, is to ensure stability during storage, as certain drugs may degrade in the presence of moisture or heat.
Packaging Solutions
Food packaging
PLA has become increasingly popular in food packaging. It offers a sustainable alternative to traditional plastics. Its key characteristic is that it is derived from renewable resources, making it appealing to eco-conscious consumers. Additionally, PLA provides a barrier against moisture and oxygen, helping to prolong the freshness of food products.
One unique feature of PLA food packaging is its compostability. Unlike conventional plastics, it can break down in industrial composting facilities. This makes it an excellent choice for products that prioritize environmental impact. However, its heat resistance is limited, which might restrict its use in certain applications requiring higher temperatures.
Compostable materials
As a biodegradable polymer, PLA is also integral in producing compostable materials. These materials can decompose under the right conditions, contributing to reduced landfill waste. The primary reason for its popularity in this area lies in its environmental benefits. PLA serves industries looking to enhance their sustainability efforts, particularly in packaging and single-use items.
The unique feature of PLA compostable materials is their ability to break down in composting environments. This contrasts sharply with traditional plastics that can persist for hundreds of years. One consideration, however, is that proper composting facilities are necessary for efficient degradation. Without these conditions, PLA may not break down as intended, emphasizing the need for proper disposal practices.
Textile and Fiber Applications
PLA is increasingly utilized in textiles and fiber applications. Its biodegradable properties offer a sustainable option for fashion and garment manufacturing. The fibers produced from PLA can mimic conventional polyester in both feel and function. They are used in clothing, upholstery, and other textile products.
The sustainability narrative surrounding PLA is vital, as the textile industry seeks to reduce its environmental footprint. One notable aspect of PLA fibers is that they can be dyed and processed similar to traditional textiles. However, durability compared to synthetic fibers remains a consideration. Ensuring that PLA textiles meet the demands of consumers is essential for broader adoption.
Poly L lactic acid continues to showcase its versatility across numerous applications, cementing its role in sustainable practices.
Advantages of Poly Lactic Acid
Poly L lactic acid (PLA) has emerged as a vital player in the realm of biodegradable materials, and its advantages make it a compelling choice for many applications across diverse industries. Understanding these advantages is crucial for students, researchers, educators, and professionals interested in sustainable materials. This section delves into specific elements of PLA that set it apart from traditional polymers, focusing particularly on its renewability and biocompatibility.
Renewable Resource Base
One of the most significant advantages of PLA is its renewable resource base. Unlike petroleum-based plastics, PLA is derived from natural resources such as corn starch or sugarcane. This aspect emphasizes sustainability, as the production of PLA not only uses resources that can be replenished but also aligns with the growing global push towards reducing reliance on fossil fuels.
Moreover, the agricultural processes to cultivate such renewable resources can often utilize techniques that are environmentally conscious. For instance, farmers can implement crop rotation to maintain soil health. By choosing PLA, industries can shift from a linear economy to a more circular model. This encourages the use of materials that can be broken down and reused, ultimately reducing waste.
Biocompatibility
Another pivotal advantage of PLA is its biocompatibility. PLA has gained significant attention in the medical field for applications such as surgical sutures and drug delivery systems. Its compatibility with the human body is largely due to its non-toxicity and ability to break down into benign by-products such as lactic acid, which is naturally present in the body. This makes PLA an appealing alternative to synthetic materials that can evoke adverse reactions.
- In surgical applications, PLA can minimize the risk of infections.
- In drug delivery, its controlled degradation rate can allow for sustained release of medications over time.
A growing body of research supports the notion that PLA's properties extend its applicability in the medical sector, indicating a promising future for this polymer.
"The use of PLA in medical applications exemplifies how integrating biodegradable materials can profoundly impact patient care and surgical practices."
Challenges and Limitations
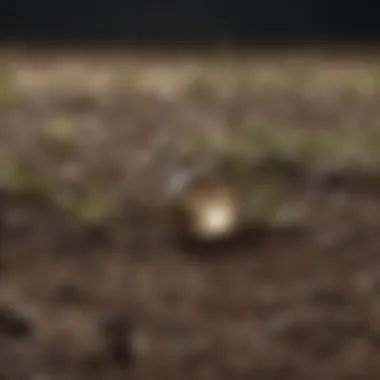
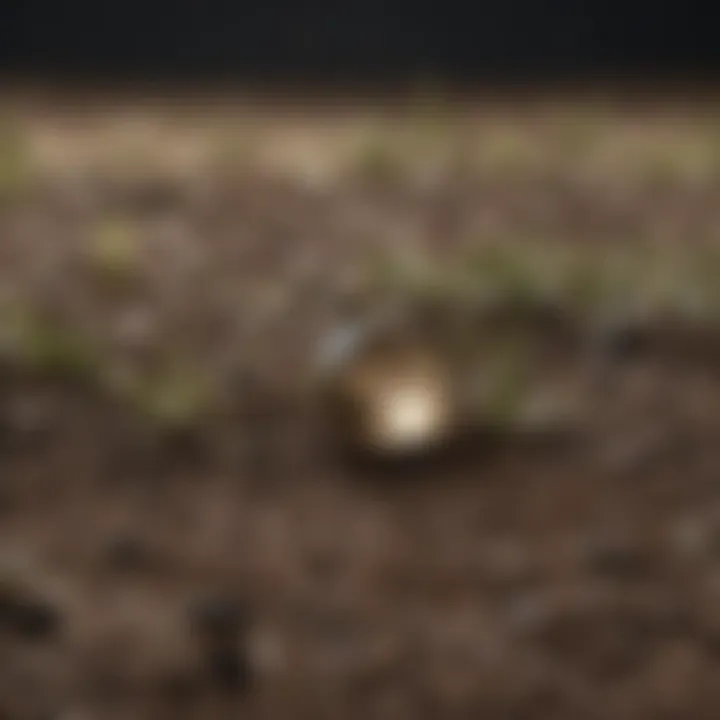
Understanding the challenges and limitations associated with poly L lactic acid (PLA) is essential for comprehending its viability as a sustainable material in various applications. While PLA has numerous advantages, these challenges can impact its feasibility. Addressing them is critical for researchers and industry professionals looking to optimize the use of PLA.
Cost of Production
One of the major drawbacks of PLA is its cost of production. The process to produce PLA is often more expensive compared to petroleum-based plastics. This stems from several factors:
- Raw material sourcing: PLA is derived from lactic acid, which is typically obtained from corn, sugarcane, or tapioca. The volatility in agricultural markets directly affects the cost of raw materials.
- Processing costs: The polymerization methods can be energy-intensive. The equipment and technology required for production may also add to the expenses.
- Economies of scale: PLA production, while growing, does not yet meet the scale of conventional plastic manufacturing, which can lead to higher unit costs.
These elements significantly challenge PLA's competitiveness in the market, particularly when comparing it with alternatives that have lower production costs.
Performance in Various Conditions
Another notable limitation of PLA is its performance across different environmental conditions. While PLA offers desirable properties such as rigidity and clarity, it also has certain weaknesses:
- Thermal stability: PLA has a relatively low melting point, around 160 Β°C. This can make it unsuitable for applications involving high temperatures, as the material may deform.
- Moisture sensitivity: PLA is sensitive to moisture, which can affect its mechanical properties. In humid environments, PLA can absorb water, leading to issues in structural integrity over time.
- Degradation speed: While PLA is biodegradable, its degradation can vary based on environmental conditions. Under composting conditions, PLA decomposes relatively quickly. However, in marine or landfill environments, the rate of breakdown can be much slower, which raises questions about its long-term environmental benefits.
"Understanding these challenges is crucial for advancing the application of PLA in sustainable practices, ensuring its benefits do not become overshadowed by limitations."
Navigating these challenges requires ongoing research and development efforts to improve the production methods and enhance the material's performance. By addressing these issues, the potential of PLA can be fully realized.
Future Directions and Research
Research related to poly L lactic acid (PLA) is crucial as it highlights innovative strategies and practices that can foster sustainable solutions across industries. Moving forward, the emphasis should be on improving synthesis techniques and broadening the applications of PLA. This exploration can potentially lead to enhanced material properties, cost efficiency, and reduced environmental impact.
Innovations in Synthesis Techniques
Innovations in the synthesis of PLA are essential for advancing its feasibility in various sectors. Currently, the predominant methods include condensation polymerization and ring-opening polymerization. However, researchers are exploring more efficient and lower-energy alternatives. Techniques such as biocatalysis and flow chemistry are gaining attention due to their ability to simplify processes and improve yields.
- Biocatalysis reduces the need for harsh chemicals, and this leads to cleaner production. Using enzymes can enable reactions to occur under milder conditions.
- Flow chemistry allows for continuous production, enhancing scalability and reducing waste. This technique can lead to more stable and controlled processes, which can be very beneficial in achieving desired molecular weights.
In addition, the incorporation of renewable biobased feedstocks in PLA synthesis is an area of significant interest. By optimizing raw material choices, researchers aim to reduce dependency on petrochemical origins.
Expanding Applications in Sustainable Practices
The applications of PLA stretch far beyond its current utilization in medical and packaging markets. Expanding its use in sustainable practices represents a substantial opportunity for industry transformation. For instance, in the textile industry, PLA fibers can provide biodegradable alternatives to traditional synthetic fibers.
Engaging in sustainable agriculture through the use of PLA for mulch films can improve soil health while minimizing microplastics. These films can degrade after their lifecycle, contributing positively to soil properties.
Other domains include:
- 3D printing: PLA is already favored in this field due to its ease of use. Further development can enable more versatile and robust filament options.
- Consumer products: Enhancing PLA formulations can enable durable household goods, from utensils to electronics. This can further reduce our reliance on fossil fuel-derived materials.
As industries pivot towards environmentally responsible practices, PLA can play a significant role in helping achieve global sustainability goals.
"Innovation in PLA synthesis and application can redefine sustainability in modern materials and reduce our ecological footprint."
Closure
In summarizing the significance of poly L lactic acid (PLA), it is vital to highlight its multifunctional properties and broad applications. This article explores various dimensions of PLA, underpinning its role in pushing the boundaries of sustainable materials. Key points revolve around its renewable resource origin, its various applications in medical fields, packaging, and textiles, as well as ongoing research aimed at overcoming certain limitations.
PLA stands as a prime example of how modern science can foster eco-friendly alternatives to traditional plastics. The discussion on PLA extends beyond general applications; it delves into specific examples, such as the use of PLA in surgical sutures and in packaging solutions that contribute to waste reduction.
Importantly, the dialogue around PLA also addresses its production costs and performance under various conditions. Such considerations are pivotal for industries looking to adopt PLA in their practices while maintaining quality and efficiency. By understanding these aspects, researchers and industries can better navigate the transition toward more sustainable material alternatives.
"The integration of PLA into everyday products can play a crucial role in mitigating plastic waste issues while aligning with environmentally conscious practices."
Ultimately, concluding this comprehensive overview underscores not just the value of PLA today, but also its potential to shape the paradigms of material science moving forward.
Summary of Key Points
- Definition and Origin: PLA is a biodegradable polymer derived from renewable resources, primarily through fermentation processes.
- Versatile Applications: Its utility spans medical applications like drug delivery and surgical sutures, as well as sustainable packaging solutions.
- Advantages: The polymer exhibits strong biocompatibility and offers a renewable resource base, making it a desirable alternative.
- Challenges: Production costs and performance in extreme conditions present hurdles for wider adoption.
- Future Directions: Ongoing research is focused on innovating synthesis techniques and expanding applications in sustainable practices.
Final Thoughts on PLA
In reviewing PLA, it is clear that this material constitutes not merely a trend in biodegradable plastics but embodies a significant shift in how industries can approach environmental challenges. Its suitability in medical applications demonstrates its efficacy in critical fields while providing a lesser environmental footprint than traditional, non-biodegradable options.
As the world grapples with the consequences of plastic waste, embracing biopolymers like PLA could serve as a benchmark for innovation aimed at sustainability. The ongoing development of PLA-based products suggests that the future of material science may welcome a pivotal transition, encouraging a more responsible manufacturing ethos.