Microbes and Their Impact on Biotechnology
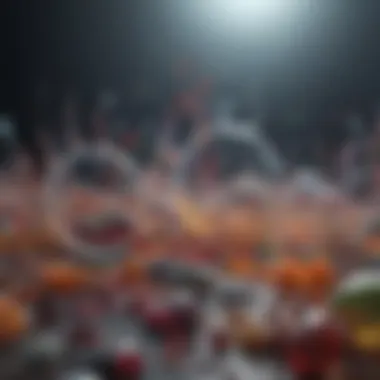
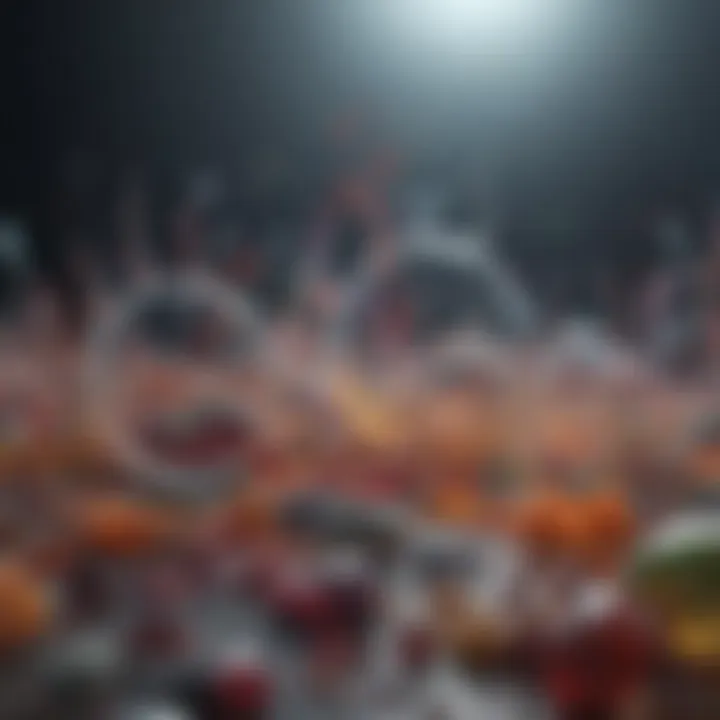
Intro
In recent decades, the interplay between biotechnology and microbiology has captured the attention of scientists and innovators alike. The small, often unseen world of microbes holds the key to various biotechnological advancements that promise to address pressing global challenges, from food security to environmental sustainability. Each type of microbe, whether bacterium, fungus, or virus, contributes uniquely to the larger tapestry of biotechnological application.
Microbes are more than just agents of disease; they can be harnessed to drive processes that yield vast benefits. For instance, fermentation, a process as old as civilization itself, relies on bacteria and yeasts to transform raw materials into essential products like bread, beer, and biofuels. Similarly, the potential of bioremediation showcases how specific microbes can clean up environmental pollutants, turning waste into resources. Notably, advancements in microbial biotechnology have paved the way for genetically engineered microbes, elevating the conversation around ethical concerns and safety.
The significance of exploring these microbial contributions cannot be overstatedβit is a journey into a world where innovation meets biology, providing insights that help humanity tackle the ever-evolving landscape of ecological and societal challenges. This discussion will unfold through a detailed examination of the following areas:
- Key roles of bacteria, fungi, and viruses
- Methodologies employed in current research
- In-depth analysis of microbial applications in biotechnology
- Ethical implications and future research directions
As we venture deeper, we will uncover the critical roles these microorganisms play in the ever-expanding field of biotechnology.
Prologue to Microbial Biotechnology
Microbial biotechnology stands at the forefront of modern science, bridging the realms of biology, chemistry, and engineering, and revealing how much we can learn from our microscopic friends. Itβs like having a toolbox filled with different tools - each microbe offers unique properties that can be harnessed for numerous applications, ranging from sustainable agriculture to the development of biofuels. In this article, we will explore the significance of microbial biotechnolgoy and how it reshapes various industries.
The importance of this field lies in its potential to leverage microorganisms to tackle pressing issues, such as waste reduction, food security, and energy shortages. When it comes to environmental concerns, microbes are not just passive players; they actively break down pollutants and contribute to nutrient cycling. So, the potential benefits are manifold and far-reaching, ultimately impacting societal wellbeing and ecological sustainability.
Definition of Microbial Biotechnology
Microbial biotechnology refers to the utilization of microorganisms to develop and manufacture products that can improve quality of life, health, and the environment. This encompasses an extensive range of processes including fermentation, bio-remediation, and genetic manipulation. In simpler terms, itβs about using tiny life forms to make things better for us in various ways.
These microbes can be bacteria, fungi, algae, or even viruses. Each type plays a specific role in producing valuable substances or enzymes, or in breaking down harmful compounds. For instance, bacteria such as Escherichia coli serve as building blocks in gene cloning techniques, while fungi like Aspergillus contribute to fermentation processes in food production. The interplay of these tiny beings and their biochemical mechanisms is what makes microbial biotechnology such an exciting field to delve into.
Historical Context and Evolution
To truly appreciate microbial biotechnology, one should understand its roots. The journey began long ago, even before the term itself was coined. Traditional fermentation techniques date back thousands of years; our ancestors used mold and yeast for bread, beer, and wine production long before any scientific framework was established.
Fast forward to the 19th century, when Louis Pasteur pioneered the study of microorganisms and their roles in fermentation. His studies laid the groundwork for the eventual development of modern microbiology. With the discovery of penicillin by Alexander Fleming in 1928, humanity witnessed a major turning point, showcasing how microbes could be harnessed for medical advancements.
The late 20th century ushered in a new era of genetic engineering, allowing us to manipulate microbial genomes and enhance their capabilities. Techniques such as recombinant DNA technology opened avenues for creating genetically modified organisms optimized for specific biotechnological applications. Over the years, the evolution of microbial biotechnology has been nothing short of revolutionary, leading us to today's landscape where microbes are integral to innovation across various sectors.
"Microbial biotechnology represents a pivotal shift in scientific thought, where tiny entities hold the key to addressing some of the largest biotic challenges we face."
Types of Microbes in Biotechnology
Understanding the types of microbes involved in biotechnology is essential. Microbes are not just tiny organisms; they are potent tools in various industries. Each group of microbes, such as bacteria, fungi, viruses, and algae, brings unique strengths to the table. Their distinctive metabolic pathways and ability to adapt to different environments make them invaluable in biotechnological applications.
In this section, we dive into the characteristics and contributions of these microbial groups, providing insight into how they can be harnessed for innovative solutions in fields like healthcare, agriculture, and environmental management.
Bacteria
Bacteria represent a significant microbial contingent in biotechnology. Their rapid growth rates and versatile metabolic abilities make them suitable for various applications. For instance, the bacterium Escherichia coli is extensively utilized in genetic engineering. Researchers modify this bacteria to produce insulin, a hormone critical for diabetes management. Similarly, certain bacteria are adept at breaking down complex organic compounds in waste materials, aiding in environmental cleanup efforts.
Their uncomplicated cellular structure allows for easier manipulation in laboratories. Furthermore, bacteria's natural ability to thrive in diverse environmentsβfrom deep-sea vents to extreme acid poolsβopens doors for developing bioprocesses in various conditions.
Fungi
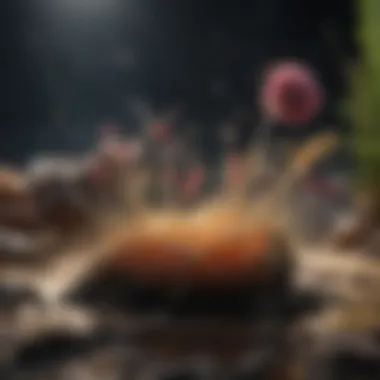
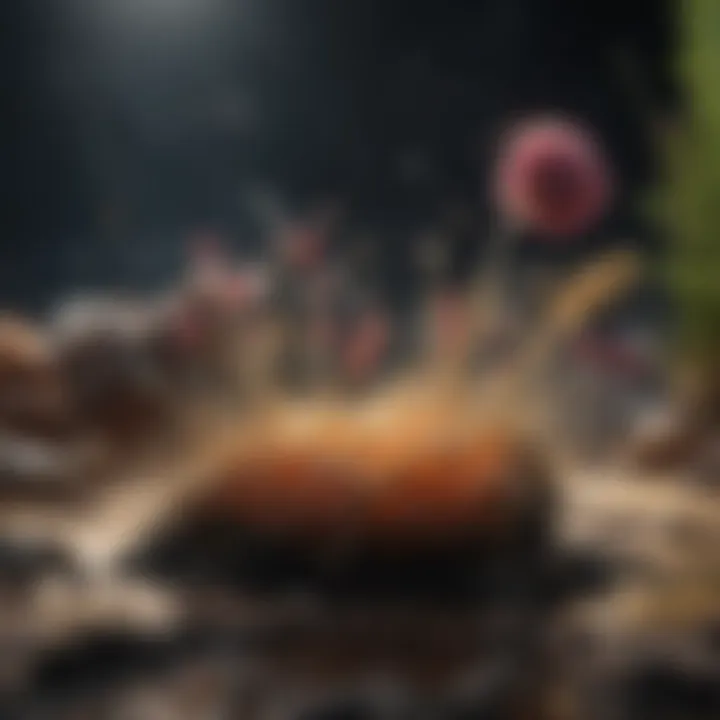
Fungi play a vital role in the fermentation industry. Yeasts, particularly the species Saccharomyces cerevisiae, have been utilized in baking, brewing, and winemaking for centuries. Their capabilities extend beyond traditional usesβscientists harness their sophisticated enzyme systems for biocatalysis in industrial syntheses. These enzymes help in breaking down starches into sugars, which can later be fermented for ethanol production.
In addition to food production, fungi can contribute to sustainable practices by decomposing agricultural residues and converting them into valuable bioproducts. This not only mitigates waste but also supports nutrient cycling within ecosystems.
Viruses
Viruses, despite their reputation as pathogens, have practical applications in biotechnology. They can be tailored for gene delivery, making them potent tools in genetic engineering. For instance, viral vectors are used in developing vaccines and treating genetic disorders by introducing healthy genes into patients' cells.
Moreover, bacteriophages, viruses that specifically target bacteria, are gaining traction as alternatives to antibiotics in treating bacterial infections. This approach, known as phage therapy, capitalizes on the specificity of bacteriophages, allowing for targeted treatments without harming beneficial bacteria in the microbiome.
Algae
Algae are unique organisms with incredible potential in biotechnological applications, especially in biofuel production. Microalgae, in particular, can produce oils that can be converted into biodiesel. They grow rapidly and require minimal resources compared to traditional crops, making them a sustainable choice for energy generation. Algae also absorb large amounts of CO2 during their growth, contributing to climate change mitigation.
Additionally, algae are rich in proteins and vitamins, and research is delving into their use as food supplements and animal feed. Their capacity to bioaccumulate substances opens possibilities in wastewater treatment, where they can remove contaminants while yielding valuable biomass.
"Through leveraging the diverse capacities of microbes, we can pave the way for innovative solutions to pressing global challenges."
Microbial Metabolism and Biochemical Pathways
Microbial metabolism refers to all biochemical processes that occur within a microbe, enabling it to survive and reproduce. Understanding these metabolic pathways is crucial in the context of biotechnology. These pathways allow microbes to convert various substrates into energy, biomass, and byproducts that may be harnessed for industrial purposes. This area of knowledge not only provides insights into the capabilities of microbes but also opens avenues for innovative applications in various biotechnological fields.
Anaerobic vs. Aerobic Metabolism
The distinction between anaerobic and aerobic metabolism is key to understanding how different microbes function in varied environments.
Aerobic metabolism involves oxygen, allowing microbes to utilize this gas for energy production. This process is highly efficient, as it uses oxygen as the final electron acceptor in the electron transport chain, producing a substantial amount of ATP, the energy currency of cells. Think of aerobic microbes as the high-performance engines, revving up energy output when oxygen is on hand. Common examples include species like Escherichia coli in favorable conditions.
On the other hand, anaerobic metabolism occurs in the absence of oxygen. Here, microbes utilize alternative electron acceptors, like nitrate or sulfate, or even organic compounds, leading to the fermentation process. Anaerobic pathways are generally less efficient than aerobic ones, but they grant survival in oxygen-deficient environments. Take the instance of Clostridium species; they thrive where oxygen is toxic, performing fermentation to generate energy from sugars without needing oxygen. Remarkably, some anaerobic processes can even lead to the production of biofuels, demonstrating their practicality in biotechnology.
"Understanding these metabolic differences not only enlightens us about microbe ecosystems but also guides us in designing biotechnological applications tailored to specific environmental conditions."
Fermentation Processes
Fermentation is undoubtedly one of the oldest biotechnological methods, linking microbes with food production and energy generation. This anaerobic process converts carbohydrates into acids, gases, or alcohol using microorganisms. Fermentation has wide-ranging applications in food industries, from yogurt to bread.
- Lactic Acid Fermentation: This is catalyzed by lactic acid bacteria, such as Lactobacillus, which convert lactose (found in milk) into lactic acid. This not only thickens dairy products but also extends their shelf life while giving them a tangy flavor.
- Ethanol Fermentation: Carried out primarily by yeast like Saccharomyces cerevisiae, this process converts sugars into ethanol and carbon dioxide. This is crucial in the production of alcoholic beverages and biofuels.
- Acetic Acid Fermentation: In this process, ethanol is oxidized to acetic acid by aerobic bacteria like Acetobacter, ideal in vinegar production.
Fermentation offers numerous benefits: it preserves food, enhances flavors, and produces valuable byproducts. However, inconsistencies can arise, influenced by factors like temperature, pH, and substrate concentration. Thus, there's a delicate balance to strike to optimize the fermentation process for industrial purposes. Overall, understanding fermentation processes enriches our toolkit in harnessing microbial capabilities for sustainable practices.
Applications of Microbial Biotechnology
The applications of microbial biotechnology are vast and diverse, showcasing the intricate relationship between microbes and various sectors of society. Understanding their roles in specific fields not only illuminates the potential of these tiny organisms but also underscores how they can drive innovation while addressing pressing global challenges. From transforming food production to cleaning up contaminated environments, the potential for microbes is nothing short of remarkable.
One of the standout applications is in the food industry. The fermentation process not only preserves food but also enhances flavors and nutritional value. As techniques evolve, new fermentation methods are being developed to create an array of products that appeal to changing consumer preferences. This aspect of microbial biotechnology serves as a lifeline, providing solutions that meet both health and taste demands.
- Sustainability: The use of microbes in food production is not just about improving taste; it's about making processes more sustainable. Fermentation reduces spoilage, extending shelf life and decreasing food waste, a critical factor in today's resource-constrained environment.
- Flavor and Nutrition Enhancement: Microbes are responsible for unique flavors in products like cheese, yogurt, and sauerkraut. They also boost probiotics, supporting health in ways that simple food items can't achieve alone.
Furthermore, microbial biotechnology presents significant opportunities in environmental restoration. Bioremediation utilizes microbes to clean up contaminated soils and water, breaking down pollutants into less harmful substances. This application underscores the natural ability of microbes to adapt and thrive in various environments, often emerging as heroes in situations where human intervention fails to yield effective results.
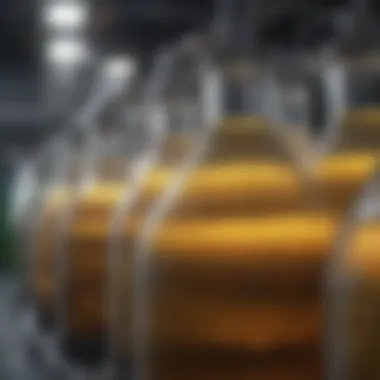
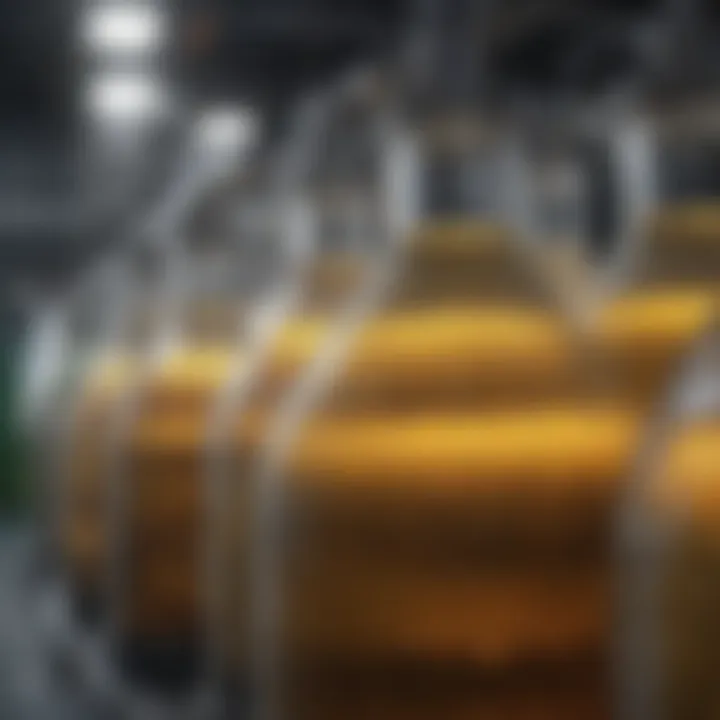
"Microbes have been nature's cleanup crew for millions of years. When leveraged correctly, their potential in bioremediation is unparalleled."
- Breakdown of Toxins: Specialized microbes can biodegrade plastics, oils, and heavy metals, showcasing their potential to combat pollution effectively.
- Cost-Effectiveness: Compared to traditional methods of cleaning contaminated sites, bioremediation could save time, resources, and money.
In terms of energy production, microbial biotechnology is a game-changer in biofuel production. Microbes are engineered to convert biomass or waste into biofuels, providing an alternative to fossil fuels. The ongoing research endeavors in this area indicate that as technology advances, the efficiency and sustainability of biofuels will continue to improve, ultimately playing a pivotal role in addressing energy crises.
- Lower Carbon Footprint: Biofuels produced from microbes emit fewer greenhouse gases than their fossil counterparts, contributing to more environmentally friendly energy sources.
- Waste Utilization: Utilizing food waste or agricultural residues for biofuel not only provides a renewable energy source but also helps divert waste from landfills.
On the pharmaceutical front, microbial biotechnology enables the production of vital medications and vaccines. Microbes have become essential tools in the pharmaceutical industry, being utilized to produce antibiotics, hormones, and even complex therapeutic agents. This avenue not only boosts medication availability but also opens the door for advancements in personalized medicine.
- Recombinant DNA Technology: This technique uses microbes to produce insulin and growth hormones, marking significant advancements in treating diabetes and hormonal disorders.
- Vaccine Development: The ability of microbes to express antigens can lead to rapid development of new vaccines, crucial in responses to emerging diseases.
In summary, the applications of microbial biotechnology span a broad spectrum, each driving innovation and sustainable practices. The exploration of these applications reveals how microbes can not only serve immediate needs but also pave the way for a more sustainable future. As our understanding of these organisms deepens, so does the potential for transformative change across various sectors.
Recent Advances in Microbial Biotechnology
Recent advances in microbial biotechnology are reshaping various sectors, including agriculture, healthcare, and energy. This area highlights innovative methods and technologies that harness microbial processes to solve pressing issues and improve efficiency in biotechnological applications. The importance of these advances cannot be understated, as they promise to address challenges like antibiotic resistance, pollution, and the quest for renewable energy sources. By understanding the latest developments in this field, it becomes clear that the potential benefits are immense, making it a topic of critical relevance in contemporary research and industry.
Synthetic Biology Approaches
Synthetic biology represents a frontier where engineering principles blend seamlessly with biological systems. This means creating new biological parts, devices, and systems or redesigning existing, natural biological systems for useful purposes. In microbial biotechnology, synthetic biology enables scientists to modify microorganisms, making them capable of performing tailored tasks that could not be achieved through traditional means. For instance, researchers now engineer bacteria to produce high-value compounds like insulin or biofuel from agricultural waste.
Some key techniques of synthetic biology include:
- Gene Editing: Altering gene sequences to enhance desired traits in microorganisms.
- Pathway Construction: Designing and assembling biochemical pathways to produce specific metabolites.
- Whole-cell Synthesis: Creating new organisms by assembling synthetic gene circuits within microbial hosts.
These strategies lead to increased productivity and the ability to design microbes that can respond to environmental stimuli. As such, synthetic biology has far-reaching implications for sustainable practices in agriculture, as well as efficient production processes in pharmaceutical industries.
CRISPR and Genetic Engineering of Microbes
The CRISPR-Cas9 system has radically transformed the landscape of genetic engineering. Originally discovered as a bacterial defense mechanism against viruses, this innovative tool allows for precise modifications of microbial genomes with unprecedented accuracy and efficiency. By utilizing CRISPR, researchers can knock out genes, insert new sequences, or modify existing ones, thus tailoring microbes for specific applications.
The applications of CRISPR in microbial biotechnology are varied and impactful. They include:
- Biofertilizer Production: Improving microbial strains that boost plant growth by enhancing their nitrogen-fixing abilities.
- Bioremediation: Engineering microbes to effectively break down environmental pollutants, such as heavy metals and plastics.
- Pharmaceutical Development: Creating microorganisms that can produce complex drugs that were once difficult or expensive to synthesize.
Moreover, with the increasing concerns regarding ethical implications in genetic manipulation, the discussions surrounding these advances have also become more pressing. Questions about safety, potential unintended consequences, and regulatory frameworks continue to shape the future of CRISPR applications in biotechnology.
"Recent breakthroughs not only advance our understanding of microbial capabilities but also challenge us to navigate the ethical landscape that accompanies such powerful tools."
Safety and Ethical Considerations
Understanding the safety and ethical considerations surrounding microbial biotechnology is not just a trivial footnote; itβs the bedrock of responsible scientific inquiry. The manipulation of microbes offers immense potential to address major societal challenges, but it also carries risks that should be carefully managed. This section will delve into the frameworks that govern microbial activities and examine the philosophical questions arising from their use, ensuring that scientific advancements do not come at the cost of ethical oversight or public safety.
Regulatory Frameworks
The role of regulatory frameworks in microbial biotechnology is akin to the rules of a road; they provide guidance to ensure that innovations do not lead to unintended consequences. In many countries, regulatory bodies such as the Environmental Protection Agency (EPA) and the Food and Drug Administration (FDA) set strict guidelines on how microbes can be developed and deployed. These regulations are designed to assess the safety of genetically modified organisms (GMOs), manage risks associated with biotechnological applications, and monitor environmental impacts.
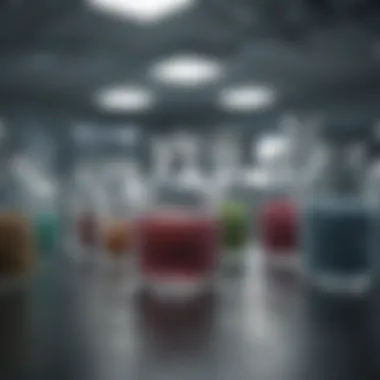
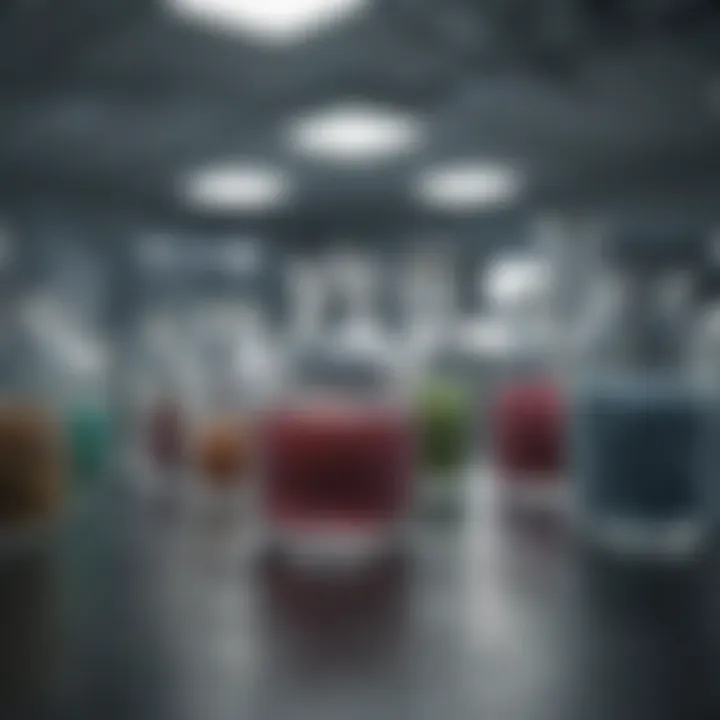
For instance, in the United States, the Coordinated Framework for the Regulation of Biotechnology outlines the responsibilities of different agencies in managing biotechnological products. This framework emphasizes a science-based approach that considers both benefits and risks. A few critical points include:
- Risk Assessment: Evaluating potential hazards associated with microbial products before they hit the market.
- Environmental Monitoring: Ongoing observation of microbial effects on ecosystems post-deployment.
- Public Involvement: Many regulatory processes invite public comment, acknowledging community concerns about safety.
The continuous evolution of these regulations demonstrates the commitment to adapt and respond to emerging challenges as science advances.
Ethical Implications of Microbial Manipulation
When we think about microbes, itβs easy to overlook their moral dimensions. Yet, as we push the boundaries of science, ethical implications must anchor our research and applications.
One of the primary ethical debates revolves around the concept of 'playing Godββthe notion that manipulating life at such a fundamental level is inappropriate. Such concerns are often tied to cultural and philosophical beliefs regarding nature and intervention. Moreover, there are deeper considerations regarding equity and accessibility: who will benefit from these biotechnological advancements?
β’ Equity in Access: As new microbial methods for agriculture or health emerge, understanding who has access to these innovations is crucial.
β’ Biodiversity: The artificial manipulation of microbes can inadvertently threaten native species, raising ethical concerns about the long-term impacts on ecosystems.
β’ Informed Consent: In the context of pharmaceuticals and gene therapies that involve microbial technologies, obtaining informed consent from patients is paramount.
"The true measure of any society can be found in how it treats its most vulnerable members." β Mahatma Gandhi.
Ultimately, engaging in ethical discussions surrounding microbial biotechnology requires a multidimensional approach. We must balance scientific progress with social responsibility, ensuring that our pursuit of knowledge does not outstrip our moral compass. Safety and ethical considerations are not just regulations; they are essential components of a thoughtful scientific community.
Future Directions and Research Opportunities
The role of microbes in biotechnology is constantly evolving, opening doors to new possibilities for application and understanding. Recognizing future directions and research opportunities is crucial, as it not only informs scientific inquiry but also guides practical applications in diverse fields. Microbial biotechnology holds the promise of addressing several pressing global challenges, such as resource scarcity, climate change, and public health concerns.
Innovative Application Areas
The landscape of microbial applications is broad and full of potential. Here are some noteworthy areas where microbes are making waves:
- Agricultural Biotechnology: Certain microbes can form symbiotic relationships with plants, enhancing nutrient uptake and disease resistance. Harnessing these relationships can lead to environmentally friendly alternatives to chemical fertilizers and pesticides.
- Wastewater Treatment: Natural processes driven by microbes are increasingly being deployed in wastewater management. Innovative systems, such as constructed wetlands and microbial fuel cells, use microbial activity to break down organic matter efficiently, transforming pollutants into harmless by-products or even energy sources.
- Biodegradable Plastics: The push towards sustainable materials has sparked interest in bioplastics derived from microbial sources. Microorganisms like Aliivibrio fischeri produce biopolymers, which can serve as the foundation for developing environmentally friendly materials that decompose naturally.
- Bioinformatics and Genomics: The integration of bioinformatics into microbial biotechnology is paving the way for revolutionary discoveries. By analyzing microbial genomes, researchers are uncovering genes that code for valuable enzymes and compounds. Such insights can be pivotal in biochemical product development.
"Microbial systems are agileβthey adapt quickly when faced with new challenges, offering us insights that can revolutionize how we approach biotechnological problem-solving.β
Challenges and Limitations in Current Research
Despite the exciting potential, several hurdles lie ahead for researchers in the field of microbial biotechnology:
- Regulatory Hurdles: The complexity of regulatory frameworks often stifles innovative microbial applications. Each country has its own rules governing genetically modified organisms (GMOs), impacting research and development timelines.
- Public Perception: The general public's perception of genetically engineered microbes can be fraught with skepticism. Efforts to enhance public understanding and acceptance of microbial technologies remain vital.
- Technical Limitations: While microbial systems can be incredibly efficient, scaling them up for industrial use presents challenges. Researchers must navigate the differences between lab-scale procedures and real-world applications, where variables can dramatically shift.
- Data Gaps and Knowledge Exchange: The field often suffers from gaps in data. Effective sharing of existing knowledge is vital for accelerating progress, yet proprietary research can inhibit collaboration and innovation.
Addressing these challenges requires a multifaceted approach, combining creativity, scientific rigor, and public engagement. Only through collaborative efforts and innovative thinking can the transformative potential of microbial biotechnology be fully realized.
Finale
The exploration of microbes in biotechnology is a vast and intertwined subject, crucial for the development of sustainable practices and innovative solutions across diverse industries. This article underscores the notable roles played by various microbial species, from bacteria to fungi, in processes that not only benefit humanity but also foster environmental balance. As we wrap up, it's essential to reflect on the key elements discussed throughout the sections and their implications for future advancements in biotechnology.
Recapitulation of Key Points
In our journey through microbial biotechnology, several vital points emerge:
- Importance of Microbes: Microorganisms like bacteria, fungi, and algae are indispensable in processes ranging from fermentation in food production to bioremediation efforts targeting environmental degradation.
- Recent Advances: Innovative techniques such as synthetic biology and CRISPR are reshaping how we manipulate and utilize these tiny powerhouses, broadening the horizon of potential applications.
- Safety and Ethics: As we harness the power of genetic engineering, a deep understanding of safety frameworks and the ethical implications becomes paramount to ensure responsible use of genetically modified microbes.
- Future Directions: The continued exploration into innovative application areas will pave the way for addressing challenges like food security, climate change, and public health issues.
The Importance of Continued Exploration
The landscape of microbial biotechnology is not static; it evolves rapidly as research progresses and new challenges arise. The need for ongoing inquiry into microbial applications cannot be overstated. Here are a few considerations:
- Addressing Global Challenges: Issues like climate change and population growth call for enhanced biotechnological solutions. Microbes could hold the key to biofuel production efficiency, pollution reduction, and even sustainable agriculture.
- Deepening Our Understanding: Each discovery unlocks doors to new applications and possibilities. As we explore the complexities of microbial interactions and metabolic capabilities, we stand to learn more about potential breakthroughs that could reshape industries.
- Collaboration Across Disciplines: Collaborative research between microbiology, chemistry, environmental science, and engineering will likely accelerate progress in microbial biotechnology. The merging of ideas and methods can lead to innovative solutions that may not be achievable in isolation.
In essence, the future of biotechnology brightly hinges upon our ability to remain curious, investigate, and adapt as we learn from the myriad behaviors of microbes. Engaging consistently with this corner of science is not just beneficial; it's imperative for both reaching new heights in technology and maintaining the delicate balance within our ecosystems.