Hydrogen Plants: Key Insights and Future Directions
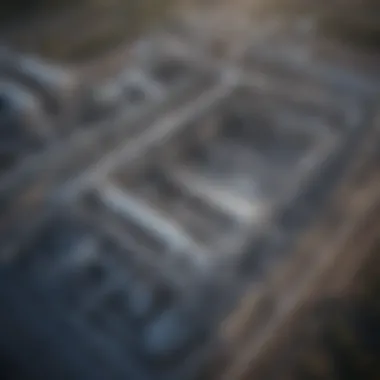
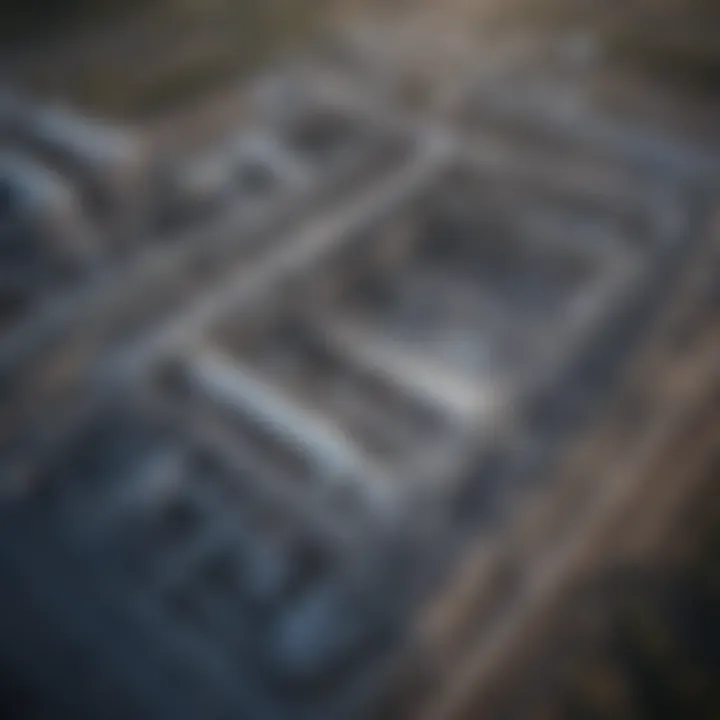
Intro
In recent years, hydrogen has emerged as a pivotal player in the global energy landscape. As nations race toward achieving carbon neutrality, hydrogen plants present a tantalizing solution, serving not only to generate power but also to catalyze an entire hydrogen economy. This sector is gaining ground for its capacity to decarbonize various industries, thus yielding a significant environmental impact.
The importance of understanding hydrogen plants cannot be overstated. They are at the heart of numerous sustainable energy initiatives, aiming to transform how we harness and utilize energy. Yet, many people are still somewhat in the dark about their foundational principles and practical applications.
In this article, we will explore the nuts and bolts of hydrogen plants, shining a light on their development, current methodologies for hydrogen production, and a future that looks increasingly hydrogen-centric. We will delve into the backbone of hydrogen plants, examining the operational mechanics and discussing how they stand up against traditional energy solutions.
Research Overview
Summary of Key Findings
Recent studies reveal that hydrogen produced via renewable sources holds more potential for sustainable energy dynamics than those derived from fossil fuels. The key findings suggest that:
- Hydrogen plays a critical role in energy storage and transportation.
- Current production methods, including electrolysis and steam methane reforming, show varying levels of environmental impact.
- Emerging technologies like green hydrogen production could reshape industry standards in the coming years.
"Hydrogen could be the fuel of the future, but its path is riddled with both opportunities and challenges."
Methodologies Employed
To assess the operational prowess of hydrogen plants, a combination of quantitative and qualitative methodologies have been utilized:
- Empirical Studies: Research focusing on actual production metrics from existing plants.
- Comparative Analysis: Evaluating the environmental impact of various hydrogen production techniques.
- Case Studies: In-depth investigations of successful hydrogen projects worldwide, including those leveraging advanced technologies.
In-Depth Analysis
Detailed Examination of Results
With growing traction towards sustainable energy, hydrogen plants are rapidly advancing. Analyzing operational efficiency and emissions can paint a clearer picture of the hydrogen sector's viability. For instance, data indicates that hydrogen produced by electrolysis powered by renewable energy sources has a markedly lower carbon footprint than traditional methods.
Comparison with Previous Studies
When juxtaposed with earlier research, the advancements in hydrogen production reflect a significant leap in technology and methodology. Historically, steam methane reforming has dominated production, but newer approaches, particularly those focusing on electrolysis and renewable inputs, are rapidly gaining favor. Not only does this shift enhance sustainability, but it also meets governmental and societal demands for cleaner energy solutions.
Preface to Hydrogen Plants
Hydrogen plants are quickly becoming essential components in the evolving landscape of energy production, drawing significant attention from researchers, policymakers, and industry leaders alike. At a time when the world grapples with issues like climate change and the need for cleaner sources of energy, understanding these facilities and their functions is paramount.
The importance of hydrogen plants lies primarily in their capacity to produce hydrogen, a versatile energy carrier that can play multiple roles in decarbonizing various sectors, from transportation to power generation. Furthermore, the growing interest in hydrogen stems from its potential to not just reduce carbon emissions but also to be integrated with renewable energy resources. By effectively harnessing hydrogen, we might just unlock a pathway towards a more sustainable energy future.
Definition and Purpose
Hydrogen plants, in simple terms, are facilities designed to produce hydrogen at scale. The purpose of these plants encompasses different production methods, including steam methane reforming, electrolysis, and other emerging technologies. Ultimately, these facilities serve a critical function in not only generating hydrogen fuel but also in supporting wider initiatives aimed at reducing greenhouse gas emissions.
These plants can be seen as bridges to a more sustainable energy system, paving the way for innovations in storage, distribution, and end-use applications of hydrogen. They play a crucial role in producing green hydrogen and implementing effective measures that advance both industrial and domestic applications of this crucial resource. The focus tends to be on ensuring efficiency while minimizing environmental impact, addressing both economic viability and sustainability concerns.
Historical Development of Hydrogen Production
The journey of hydrogen production dates back to the discovery of hydrogen itself, marked by significant milestones along the path to modern production methods. Initially, hydrogen was viewed merely as a laboratory curiosity or an industrial gas, primarily utilized in the manufacturing of ammonia, one of the key ingredients for fertilizers.
Fast forward to the 20th century and we can see a gradual shift in perception as hydrogen began to gain recognition as an energy source. The oil crises of the 1970s acted as a catalyst, pushing nations to explore alternative fuels, including hydrogen. Fast-forwarding to today, researchers and companies worldwide are striving to improve production techniques, driven largely by impressive advancements in technology and growing environmental awareness.
The emergence of renewable energy sources has also redefined hydrogen’s role: it’s no longer just a supplement to traditional fuels but a potential cornerstone of an entirely new energy paradigm.
The historical development of hydrogen production illustrates not only the evolution of technology but also the shifts in societal attitudes towards sustainable energy solutions.
From a scientific perspective, the evolution in hydrogen production has led to increasingly sophisticated processes capable of yielding reliable outcomes. This evolution is not merely chronological; it reflects the persistent efforts to achieve higher efficiency, lesser environmental footprints, and enhanced safety in handling hydrogen as an energy resource. In doing so, hydrogen plants must keep their eyes on the road ahead, ensuring their continued relevance as stakeholders work toward an energy future deeply rooted in sustainability.
Fundamentals of Hydrogen Production
The importance of understanding the fundamentals of hydrogen production cannot be overstated in the current energy discourse. As we transition from traditional fossil fuels to more sustainable alternatives, hydrogen stands out as a versatile energy source. In this section, we will examine various methods of hydrogen production, emphasizing their técnica and applicability in today’s energy landscape. By mastering these fundamentals, stakeholders can make informed decisions that align with broader sustainability goals.
Methods of Hydrogen Production
Hydrogen can be produced through several methods, each showcasing its unique attributes, benefits, and challenges. Let’s explore three dominant methods in detail:
Steam Methane Reforming
Steam Methane Reforming (SMR) is a widely used process that converts natural gas into hydrogen. This method involves reacting methane with steam over a catalyst, generating hydrogen and carbon dioxide. One of the key characteristics of SMR is its cost-effectiveness; natural gas is plentiful and relatively cheap, making this process highly economical.
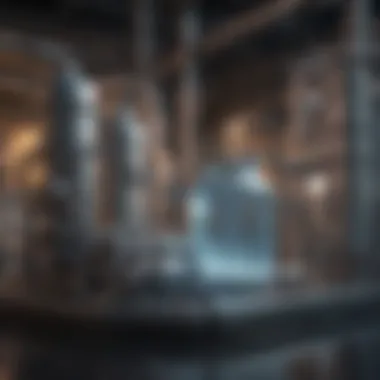
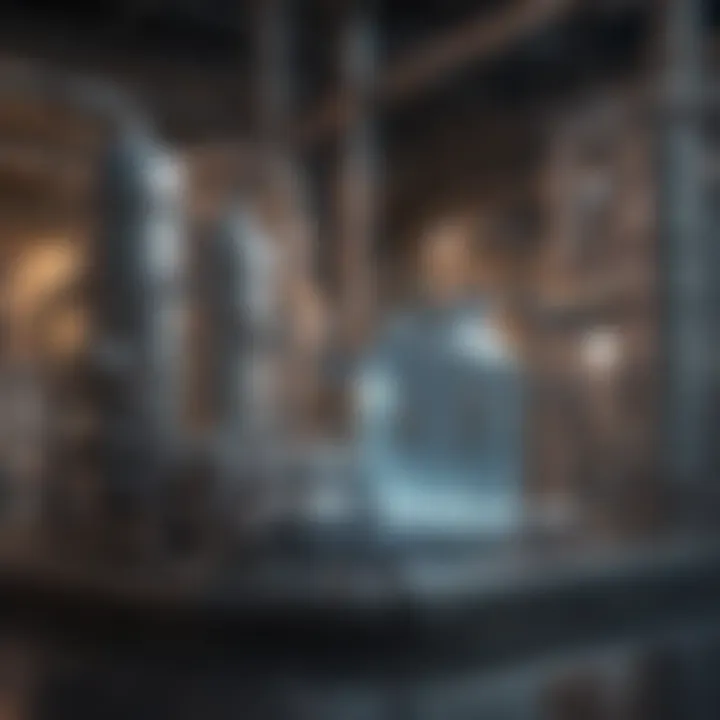
However, despite its affordability, SMR’s major drawback lies in its carbon emissions, which contradicts the sustainability goals of the hydrogen economy. The unique feature of this method is its large-scale applicability, catering to existing industrial demands. Still, as nations aim to reduce their carbon footprints, integrating carbon capture technologies alongside SMR could be a way forward.
Electrolysis
Electrolysis is an appealing method of hydrogen production as it splits water into hydrogen and oxygen through electricity. The key characteristic of electrolysis that makes it beneficial is its potential to utilize renewable energy sources, like solar and wind power. This method generates green hydrogen, contributing significantly to a low-carbon future.
While innovations continue to reduce costs, the unique feature of electrolysis lies in its ability to be deployed in various sizes, catering to both small-scale and large-scale operations. However, the main disadvantage is that it still requires substantial electrical input, which can be expensive, depending on energy prices.
Thermochemical Water Splitting
Thermochemical Water Splitting leverages high temperatures to produce hydrogen from water using thermochemical cycles. This method is garnering attention due to its high efficiency and low carbon footprint. A significant aspect of this method is that it can be powered by solar energy, presenting a harmonious relationship with renewable sources.
The distinctive feature of thermochemical water splitting is its capacity to operate at high thermodynamic efficiency. However, the technology is still in the experimental phase, facing challenges regarding material durability and system complexity. Despite these challenges, its potential contribution to a sustainable hydrogen economy is promising.
Material Requirements for Production
For effective hydrogen production, certain materials and conditions are paramount. Here we dive into two critical aspects: feedstocks and energy inputs.
Feedstocks
Feedstocks are essential for hydrogen production, determining both the efficiency and environmental impact of manufacturing methods. In the context of hydrogen production, methane is a common feedstock in SMR, while water is pivotal for electrolysis. The key feature here is the versatility of feedstocks, which can range from fossil fuels to biomass and water.
The unique characteristic of utilizing various feedstocks lies in its adaptability to local resources and infrastructure. On the downside, reliance on fossil-based feedstocks for methods like SMR may result in carbon emissions, thus challenging the sustainability aspect of hydrogen production.
Energy Inputs
Energy inputs are crucial in hydrogen production's efficiency and sustainability. Each production method requires distinct energy levels, which directly influences operating costs. For instance, electrolysis needs a significant electrical input, potentially making it less attractive from an economic standpoint without established renewable energy sources.
A key feature of energy inputs is their impact on the lifecycle and sustainability of the hydrogen produced. Higher reliance on renewable energy is essential to enhance the eco-friendliness of the process. However, challenges remain, particularly concerning the accessibility and cost of renewable energy solutions.
Understanding the fundamentals of hydrogen production is key to unlocking its potential in a future powered by sustainable energy systems.
In summary, comprehending the fundamentals equips industry stakeholders with a deeper insight into hydrogen production’s varied methodologies and material requirements. This foundational understanding serves as a springboard for exploring advanced topics in hydrogen technologies and future developments.
Technological Innovations in Hydrogen Plants
In the rapidly evolving field of energy production, hydrogen plants have come to the forefront due to their promising potential for sustainable energy solutions. This section focuses on the technological innovations within these plants, emphasizing their significance not only for efficiency but also for ecological sustainability. As the world transitions to greener energy sources, advancements in hydrogen production become crucial. These innovations hold the key to overcoming existing barriers in hydrogen production, making it a viable alternative to fossil fuels.
Advancements in Electrolysis Technology
Electrolysis technology plays a vital role in hydrogen production. Essentially, it is the process of using electricity to split water into hydrogen and oxygen. Recent advancements in this area are paving the way for more efficient systems. For instance, next-generation electrolyzers, like proton exchange membrane (PEM) and anion exchange membrane (AEM) electrolyzers, have shown increased efficiency and durability.
Additionally, improvements in catalyst materials have resulted in lower energy requirements for splitting molecules. By employing non-precious metals, researchers are reducing costs significantly. The output of these innovations is, therefore, a more economically feasible method for generating hydrogen, making it attractive for large-scale applications.
- Benefits of Advancements in Electrolysis Technology:
- Increased efficiency
- Reduced operational costs
- Enhanced durability of systems
These improvements underscore an exciting era for electrolysis technology, enhancing its potential to contribute significantly to hydrogen production.
Integration with Renewable Energy Sources
Integrating hydrogen production with renewable energy sources presents a promising pathway toward sustainability. This synergy allows for the utilization of surplus energy generated by wind, solar, or hydropower. During low-demand periods, excess energy can be leveraged to produce hydrogen, which can then be stored and utilized during peak times.
For example, Germany has made strides in combining wind energy with hydrogen production, showcasing how renewable resources can effectively contribute to a hydrogen economy. By converting surplus electricity into hydrogen, there is a dual benefit: it stabilizes the electrical grid and provides a clean fuel option.
"Integrating hydrogen production with renewable energy is like having your cake and eating it too; it maximizes resources and minimizes waste."
- Key Considerations for Integration:
- Energy storage for grid stability
- Efficient use of surplus renewable energy
- Development of infrastructure to support this integration
This innovation not only underscores a mature approach to energy management but also cultivates a cleaner future.
Role of Hydrogen Storage Technologies
Hydrogen storage solutions are paramount in addressing one of the significant challenges in the hydrogen ecosystem. As hydrogen production scales, the need for safe, efficient storage technologies becomes increasingly important. Accurate storage systems ensure that hydrogen can be transported and utilized without significant losses or hazardous situations.
Recent innovations have focused on enhancing storage methods, primarily in three areas: compressed hydrogen, liquid hydrogen, and metal hydrides.
- Compressed Hydrogen:
- Liquid Hydrogen:
- Metal Hydrides:
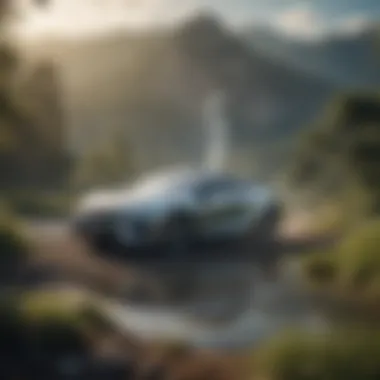
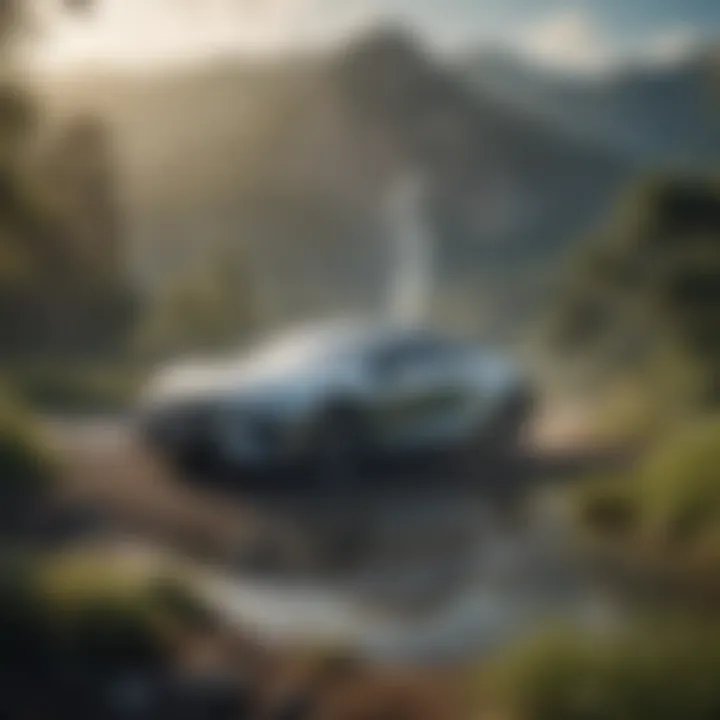
- High-pressure tanks allow for considerable volume storage
- Energy-efficient in terms of transport
- Enables larger scale storage options
- Requires cryogenic technologies
- Absorb hydrogen at lower pressures
- Provides safety through solid-state storage
The development of cutting-edge hydrogen storage technologies can dictate the future viability of hydrogen as an energy carrier.
Investments in these technologies are critical, enabling the successful maneuvering of hydrogen within a larger energy system.
Environmental Impact and Sustainability
The increasing global focus on renewable energy has shifted attention to the environmental impact and sustainability of hydrogen production. Understanding how hydrogen plants can operate sustainably is paramount in discussions surrounding energy transitions and climate action. As the world grapples with issues like climate change, the assessment of hydrogen’s environmental footprint is essential. Hydrogen production methods have differing impacts, and electing cleaner production techniques can greatly enhance sustainability in the energy sector.
Carbon Footprint of Hydrogen Production Methods
The carbon footprint associated with hydrogen production varies significantly depending on the method used. For instance, steam methane reforming, a commonly utilized technique, involves extracting hydrogen from natural gas but releases carbon dioxide during the process. This has raised concerns about long-term environmental impacts, especially as nations strive to meet stringent emission targets set forth in climate agreements.
Conversely, methods like electrolysis present a much cleaner alternative when powered by renewable energy sources. If powered by solar or wind energy, the carbon emissions from electrolysis approach zero, making it a vastly superior option from an environmental perspective.
Some key points to consider regarding the carbon footprint of hydrogen production methods include:
- Method Efficiency: The efficiency with which each method converts feedstock into hydrogen directly impacts the carbon output.
- Energy Source: Utilizing renewable energy dramatically reduces the overall carbon emissions in hydrogen production.
- Byproducts Management: Strategies for managing byproducts from production processes can influence environmental impact based on the method used.
Lifecycle Analysis of Hydrogen Systems
A lifecycle analysis (LCA) assesses the environmental impacts associated with all stages of a hydrogen system’s life—from cradle to grave. When considering hydrogen plants, it's essential to analyze not just the production phase but also the transportation, storage, and eventual end use of hydrogen. Each phase contributes to the overall environmental impact and deserves careful evaluation.
Key elements in the LCA of hydrogen systems include:
- Production: Considering emissions from producing hydrogen, including energy use and feedstock sourcing.
- Transportation: Analyzing the environmental impact of transporting hydrogen, which depends on the distance and method of transport.
- Storage: Evaluating the environmental costs involved in storing hydrogen, which can vary based on the technology used.
- Usage: Understanding how hydrogen is utilized impacts the total emissions, especially in applications like fuel cells versus combustion.
Ultimately, a comprehensive lifecycle analysis not only helps identify areas for improvement but also enables stakeholders to make informed decisions about adopting hydrogen technologies. It sheds light on how strategic development and innovation in hydrogen plants can lead to better outcomes for both energy production and the environment.
Economic Considerations
When discussing hydrogen plants, the economic landscape plays a pivotal role in shaping their feasibility and future landscape. Understanding the economic considerations is essential not just for stakeholders, but also for assessing the viability of hydrogen as a sustainable energy source. Factors such as production costs, market dynamics, and overall investment impacts determine how hydrogen can compete in the energy market.
The economic viability of hydrogen projects hinges on various elements, from the capital outlay for building plants to operating costs and potential returns on investment. Balancing these factors can either amplify or hinder hydrogen’s role in the global energy transition.
Cost Analysis of Hydrogen Production Techniques
The production cost of hydrogen varies significantly among different methodologies. Each technique boasts its own set of financial metrics, affected by factors such as technology maturity, input costs, and scale of production.
- Steam Methane Reforming (SMR) usually dominates the market, offering competitive prices due to its established nature. However, its reliance on fossil fuels comes with its own set of environmental implications.
- Electrolysis, while cleaner, remains largely constrained by its higher costs. As renewable energy sources become cheaper, the costs associated with electrolysis might see a dip, making it a more attractive option in the long run.
- Thermochemical Water Splitting is less common but presents intriguing potential if capital and operational costs can be effectively managed.
In summary, while the SMR process tends to lead in affordability, ongoing technological advancements are essential to enhance the economic attractiveness of cleaner methods.
Market Dynamics and Future Pricing
Market dynamics around hydrogen production are in a state of flux. The energy transition, influenced by climate policies and technological advancements, is shifting the demand side of hydrogen. Factors contributing to the future pricing of hydrogen include:
- Regulatory Frameworks: Governments worldwide are implementing incentives and regulations aimed at reducing carbon emissions, often favoring hydrogen solutions. This push can elevate hydrogen's market status.
- Technological Advancements: New methods of production, particularly those integrated with renewable energy systems, may lower production costs over time.
- Global Competition: As more players enter the hydrogen market, competitive pressures could dictate pricing strategies.
The future of hydrogen pricing is tied to these evolving dynamics. As production techniques become more cost-effective and the market validates hydrogen’s role in a diversified energy portfolio, one could anticipate fluctuating prices influenced by both supply capabilities and demand characteristics.
"The road to a robust hydrogen economy is paved with economic insights as much as technological innovations."
Challenges Facing Hydrogen Plants
The topic of challenges facing hydrogen plants is not just a peripheral concern; it lies at the heart of the discourse surrounding hydrogen as a pivotal energy source for the future. Hydrogen plants must navigate a myriad of obstacles that can stall progress and impact their effectiveness. Addressing these challenges is crucial for optimizing the production, distribution, and utilization of hydrogen fuel, which ultimately influences the transition to renewable energy. Two primary challenges are infrastructure limitations and regulatory hurdles. Both pose significant barriers to the development of a robust hydrogen economy.
Infrastructure Limitations
Infrastructure limitations stand out as a significant hurdle for hydrogen plants. The current energy infrastructure is predominantly built around fossil fuels, leaving scant room for the integration of hydrogen technologies. This transition requires substantial investment and strategic planning. For instance, builiding networks for hydrogen distribution involves retrofitting existing pipelines or constructing completely new ones that can handle hydrogen's distinct properties.
Hydrogen is lighter than air and has the tendency to embrittle metals, demanding specialized materials for storage and transport. Adapting existing infrastructure can be more than simply patching new systems onto old ones; it often requires a complete redesign in many cases. Moreover, the availability of fuel stations equipped to dispense hydrogen for vehicles is minimal when compared to gas stations. This lack of accessibility limits consumer acceptance and can discourage industries from adopting hydrogen technologies.
To overcome these limitations, investments in research and development, as well as partnerships with private enterprises, could foster innovations in hydrogen infrastructure. For example, implementing pilot programs in urban areas could set a precedent for wider adoption. Ultimately, a sustained effort in deploying a comprehensive hydrogen infrastructure is vital for ensuring that hydrogen production aligns with broader energy goals.
Regulatory Hurdles
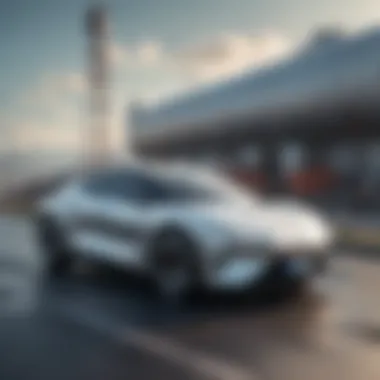
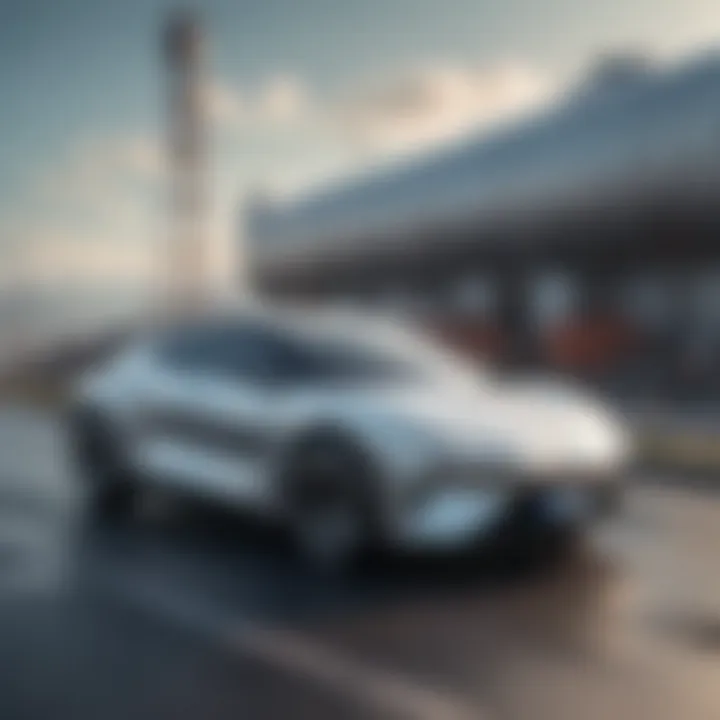
On the regulatory front, hydrogen has *often been somewhat of an afterthought. Unlike well-established energy sources, hydrogen lacks a clear regulatory framework. This gap can lead to inconsistent policies across different regions, creating uncertainty for businesses and investors. Each country has its standards and certifications related to hydrogen production, storage, and distribution, which can complicate international collaboration or scaling up technologies.
The safety regulations surrounding hydrogen are particularly stringent. Given hydrogen's flammability and properties, compliance with rigorous safety standards is a must. However, these regulations can sometimes seem archaic, lagging behind technological advancements. Thus, navigating this regulatory maze can increase costs and delays, hampering projects that aim to capitalize on hydrogen's potential.
Additionally, securing permits for new hydrogen facilities can often take immense time and resources. Transparency in these processes is essential, along with a clear legislative framework that provides guidelines for safety without stifling innovation.
Future Directions of Hydrogen Technology
As we stand on the cusp of a significant energy transition, the importance of hydrogen technology cannot be overstated. Many nations are exploring hydrogen as a cornerstone for achieving sustainable energy goals. It has the potential to reduce greenhouse gas emissions, diversify energy sources, and enhance energy security. Understanding the future directions of hydrogen technology equips stakeholders with the knowledge they need to navigate this rapidly evolving landscape.
Innovations on the Horizon
The next few years promise a surge in innovations within the hydrogen sector. Several key areas are expected to contribute significantly to hydrogen technology advancements:
- Next-Generation Electrolyzers: As the push for green hydrogen intensifies, new electrolyzer designs are emerging that will increase efficiency and reduce costs. Innovations such as solid-state electrolysis or high-temperature electrolysis present possibilities that were previously thought impractical.
- Advanced Materials: Research into catalysts and membranes could enhance the production rate of hydrogen while lowering energy consumption. Using materials like graphene or novel alloys might lead to breakthroughs in efficiency and lifecycle longevity.
- Decentralized Hydrogen Production: With the rise of small-scale, modular hydrogen plants, it's possible to generate hydrogen closer to end-users, minimizing transport costs and energy losses. Local production would also allow for the integration of renewable sources more effectively.
- AI and Digital Twins: The incorporation of artificial intelligence in monitoring systems might optimize hydrogen production processes. By using digital twins—virtual models of physical systems—operators can simulate different scenarios, thus increasing efficiency and reducing downtime.
These innovations not only promise economic benefits but also pave the way for sustainable practices that will define the next phase of energy production.
"Innovation and technology are the engines of growth in the hydrogen economy."
Hydrogen's Role in Energy Transition
Hydrogen is increasingly recognized as a pivotal player in the transition toward sustainable energy. It is not just another fuel source; it possesses unique qualities that enable it to bridge various sectors:
- Interconnectivity with Renewables: Hydrogen can be generated using excess electricity from solar or wind farms, making it a dynamic partner for intermittent energy sources. This synergy promotes grid stability and optimizes energy use.
- Decarbonization of Industries: Sectors like steel production and chemical manufacturing are heavy polluters. Hydrogen can replace or supplement fossil fuels in these processes, supporting significant reductions in carbon emissions while facilitating the necessary industrial shifts.
- Transport Transformation: Hydrogen fuel cell vehicles are positioned to revolutionize the transport sector, particularly for heavy-duty and long-range applications where battery technology encounters limitations. The establishment of hydrogen refueling stations could enable a cleaner transportation system.
- Energy Storage Solution: Hydrogen serves as an effective means for storing energy. It can retain surplus energy produced during peak periods to be utilized during high-demand phases, addressing one of the biggest challenges with renewable energy.
In a world grappling with climate change and dwindling resources, hydrogen's versatility and potential for decarbonization positions it at the heart of future energy strategies. The importance of continued investment, research, and collaboration among industries and governments cannot be overlooked as we seek to tap into the full potential of hydrogen.
Case Studies in Global Hydrogen Initiatives
Examining real-world examples of hydrogen initiatives helps to illuminate the potential benefits and challenges inherent in hydrogen production. These case studies not only demonstrate practical implementations of hydrogen technology but also offer valuable insights for policymakers, investors, and researchers alike. Through the analysis of successful projects worldwide, we can glean broader lessons that shape the future of hydrogen in the energy landscape.
Successful Hydrogen Projects Worldwide
Several pioneering hydrogen projects have emerged globally, showcasing innovative approaches to the production, storage, and utilization of hydrogen. Here are a few noteworthy examples:
- Hyundai's Hydrogen Fuel Cell Technology: The South Korean automotive giant Hyundai has led the charge in hydrogen fuel cell vehicles with its NEXO model, which runs entirely on hydrogen, drawing considerable attention towards hydrogen as a sustainable transport option.
- The Hydrogen Energy Supply Chain (HESC) Project: Taking place in Australia and Japan, this project aims to convert brown coal into hydrogen for transportation to Japan. This initiative strengthens international ties and showcases how hydrogen can be pivotal in economic exchanges.
- The Nort Project: In the Netherlands, this ambitious project revolves around producing green hydrogen from offshore wind farms. It's an exemplary model of integrating renewables with hydrogen production, setting a benchmark for sustainable energy initiatives around the globe.
Each of these programs reflects regional strengths and showcases how disparate environments can foster hydrogen technology development while nevertheless confronting unique challenges.
Lessons Learned from Hydrogen Initiatives
The analysis of established hydrogen initiatives reveals several crucial takeaways, encapsulating both success factors and areas for improvement:
- Cross-Industry Collaboration: Successful hydrogen projects often require cooperation among various stakeholders—government bodies, private sector enterprises, and research institutions. An illustration of this is how the Nort Project unites energy companies, the Dutch government, and academia.
- Importance of Policy Frameworks: Supportive regulations and incentives can significantly accelerate hydrogen projects' progress. Countries with favorable policies, like Japan's focus on hydrogen energy, have seen more rapid technological advancement.
- Scalability of Technology: Initiatives emphasize the necessity for scalable solutions. For instance, while the HESC Project showcases potential large-scale hydrogen production and distribution, scaling operations remains a critical concern, necessitating persistent innovation.
- Educating Stakeholders: Raising awareness and understanding of hydrogen-related technology among stakeholders is indispensable. Educational campaigns can help alleviate misconceptions and boost acceptance.
In summary, the cases highlighted illustrate the importance of not just technical innovation but also strategic planning and collaboration in establishing a sustainable hydrogen economy. Understanding the successes and failures of these initiatives provides a roadmap, both inspiring and cautionary, for others looking to engage in hydrogen projects in the future.
"Real-world examples serve as our guideposts—helping us discern effective strategies while cautioning us against repeating past mistakes."
Hydrogen's future remains bright, but it is through the diligent study of past initiatives that we can pave the way towards sustained and impactful advancements.
Finale: The Path Ahead for Hydrogen Plants
The journey of hydrogen technology is far from over, and the future appears promising yet complex. As global energy needs evolve, hydrogen plants will play a pivotal role in shaping sustainable energy landscapes. The critical aspects of this evolution revolve around reducing carbon footprints, improving production efficiency, and integrating with existing energy systems.
One of the core benefits of hydrogen energy lies in its versatility. As it stands, hydrogen can serve various purposes, from powering vehicles to feeding into the electricity grid. This adaptability enhances the resilience of energy infrastructures, making hydrogen a linchpin in the broad spectrum of energy strategies. Moreover, the potential for clean energy storage through hydrogen cannot be overstated. This capability means that hydrogen can help bridge the gaps in energy supply, especially when renewable sources like solar and wind are inconsistent.
In addition to these benefits, international collaborations and research initiatives are increasing. Engineers, scientists, and policymakers are working hand in hand, aiming to understand better and optimize hydrogen production technologies. Investing in hydrogen infrastructure paves the way for easing future transitions to greener energy solutions.
"The adaptation of hydrogen technology promises a transition to a cleaner energy future, but it requires careful planning and collective effort."
Summarizing the Key Points
- Hydrogen plants are increasingly essential in addressing climate change and meeting energy demands.
- These plants not only produce hydrogen but also offer advantages like flexible storage and integration with renewable sources.
- Continuous advancements and collaborations within the sector are crucial for overcoming existing challenges.
- Investment in infrastructure and technology will facilitate smoother adoption of hydrogen energy.
In summary, hydrogen's role in our energy future is significant. Its potential to contribute to cleaner energy solutions is being recognized more and more across various industries.
Final Thoughts on the Future of Hydrogen Energy
Looking ahead, the future of hydrogen energy hinges on several key elements. The enhancement of production methods such as electrolysis and biomass gasification will be instrumental. Future improvements must focus on driving down costs and increasing efficiency to make hydrogen a more competitive player in the energy market.
Additionally, the expansion of the hydrogen economy relies on public acceptance and regulatory frameworks that support innovation. Governments are tasked with laying down the groundwork for policies that incentivize investment and research. Without robust regulatory structures, the full potential of hydrogen cannot be realized.
Furthermore, it's critical to establish partnerships across sectors—from production to end-users. Collaboration among energy companies, manufacturing industries, and research institutions will drive the innovation needed to realize hydrogen's potential.
In closing, the path ahead for hydrogen plants emerges as a blend of challenge and opportunity. By strategically navigating this landscape, stakeholders can position hydrogen not just as an alternative energy source, but as a cornerstone for a sustainable future.