Exploring the RT-PCR Test: Principles and Applications
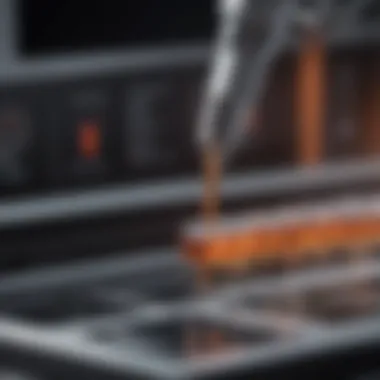
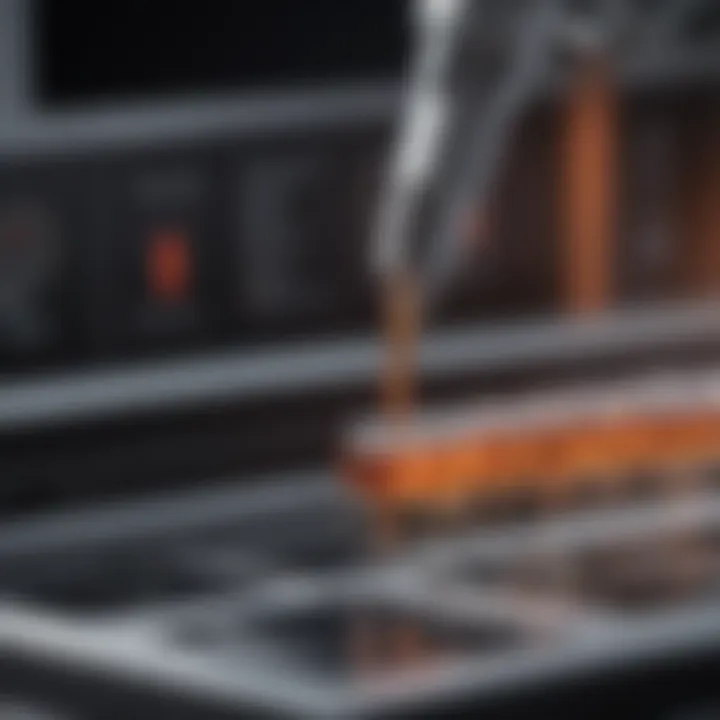
Intro
The RT-PCR test plays a pivotal role in the realm of molecular biology, especially when it comes to diagnosing infectious diseases. Understanding how this technique operates not only sheds light on its significance in current health crises but also uncovers the complexities behind its applications. This introduction sets the stage for a deeper exploration of RT-PCR, breaking down its mechanics, importance in science, and future implications for research and diagnostics.
Diving into the very essence of RT-PCR, it becomes clear that this test is more than just a laboratory procedure. It is an advanced method that assists in identifying and quantifying specific nucleic acid sequences. With its growth in popularity, especially during outbreaks like COVID-19, RT-PCR has transcended its initial academic foundations. Researchers, clinicians, and even educators have found themselves needing a robust understanding of this technique for practical applications.
The upcoming sections will delve into the nuts and bolts of RT-PCR, starting from a comprehensive overview of its research implications, then transitioning into its in-depth analysis.
Research Overview
Understanding the broader landscape of research involving RT-PCR is essential for grasping its impact. This section highlights several key points, ensuring we lay a solid groundwork for the detailed examination that follows.
Summary of Key Findings
RT-PCR has proven to be an invaluable tool across various domains, particularly in diagnostic medicine. Some key findings include:
- Rapid Detection: The ability to quickly identify pathogens has been vital during health emergencies.
- High Sensitivity and Specificity: This test significantly reduces the chances of false results, making it indispensable for accurate diagnostics.
- Versatility: Beyond infectious diseases, RT-PCR is also useful in cancer research, genetic testing, and more.
Methodologies Employed
The methodologies behind RT-PCR are intricate yet organized, ensuring reliability in results. Here are some crucial components of these methodologies:
- Sample Collection: The first step often involves collecting samples from patients, usually through nasal swabs or blood draws.
- RNA Extraction: Once obtained, the RNA is isolated to prepare for amplification.
- Reverse Transcription: This process involves converting RNA into complementary DNA (cDNA), forming the basis for further analysis.
- PCR Amplification: Finally, the cDNA is amplified using specific primers to detect the target sequences.
Understanding these methodologies provides a clearer picture of the workflow and technical skill involved in RT-PCR tests.
"RT-PCR is like having a high-powered magnifying glass. With it, you can see not just what's there, but also what could be hiding out of sight."
In-Depth Analysis
As we move forward, let's break down the findings further to truly appreciate the current state of RT-PCR and how it stacks up against prior research.
Detailed Examination of Results
Diving deeper into the results garnered from RT-PCR tests can illustrate its effectiveness and reliability. Noteworthy aspects include:
- Diagnostic Accuracy: Studies often report success rates above 95%, highlighting its prominence in clinical settings.
- Role in Infectious Disease Management: RT-PCR has altered how outbreaks are managed, enabling swift public health responses.
Comparison with Previous Studies
When juxtaposing RT-PCR results against earlier methods such as traditional cultures or serological tests, several contrasts arise:
- Speed: Unlike conventional methods that can take days to yield results, RT-PCR typically delivers findings within hours.
- Range of Applicability: Previous methods may struggle with certain types of pathogens, whereas RT-PCR has demonstrated adaptability across numerous viral and bacterial species.
Intro to RT-PCR
The realm of molecular biology has witnessed an astonishing evolution, and RT-PCR stands as a cornerstone in this development. This section lays the groundwork for understanding what RT-PCR is, why it matters, and the foundational technology that paved the way for it. From clinical diagnostics to research applications, the importance of RT-PCR cannot be overstated. It serves as a crucial tool, providing insights into the genetic material of organisms, particularly viruses.
Background of PCR Technology
To appreciate the complexity of Reverse Transcription Polymerase Chain Reaction (RT-PCR), one needs to first grasp the basics of its predecessor, the Polymerase Chain Reaction (PCR). First developed in the 1980s by Kary Mullis, PCR revolutionized molecular biology by enabling scientists to amplify specific DNA segments exponentially. The method is like a photocopier for genes; it allows a tiny amount of DNA to be replicated into millions of copies, making analysis feasible.
Essentially, PCR harnesses the natural process of DNA replication, employing cycles of heating and cooling to separate strands and then synthesize new strands using the enzyme DNA polymerase. With PCR as its backbone, RT-PCR takes this innovation a step further by integrating reverse transcription, which transforms RNA into complementary DNA (cDNA). Thus, understanding PCR technology not only lays the foundation but also illustrates the ingenuity of molecular techniques.
Definition of RT-PCR
RT-PCR can be concisely defined as a variation of the standard PCR technique that is specially tailored for analyzing RNA instead of DNA. While PCR focuses on replicating DNA sequences, RT-PCR begins its process with RNA. This difference is of paramount importance, especially when working with organisms like viruses, which may only exist as RNA.
In a typical RT-PCR procedure, the first step involves reverse transcription, where an enzyme called reverse transcriptase synthesizes cDNA from the given RNA template. Once cDNA is formed, standard PCR techniques amplify specific segments of this complementary DNA, enabling detailed study or diagnostic testing. This ability to detect RNA specifically has placed RT-PCR at the forefront of diagnosing diseases like COVID-19, where quick and accurate detection of viral RNA is crucial for effective response and treatment.
"RT-PCR not only amplifies genetic material, but also provides a lifeline in the fight against infectious diseases by allowing rapid diagnosis and monitoring of viral loads."
The implications of RT-PCR extend far beyond just diagnostics. Its versatility allows researchers to dive deeper, facilitating studies in gene expression, viral load monitoring, and even genetic mutations. With the advances made in this technology, RT-PCR remains an invaluable asset in both clinical and research labs, combining precision with the breadth needed to tackle pressing health concerns.
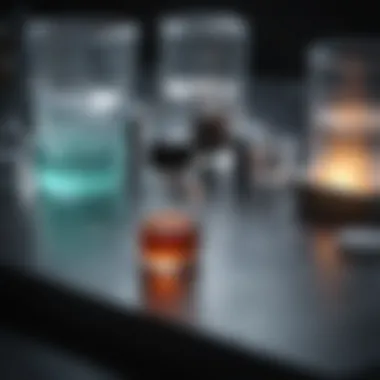
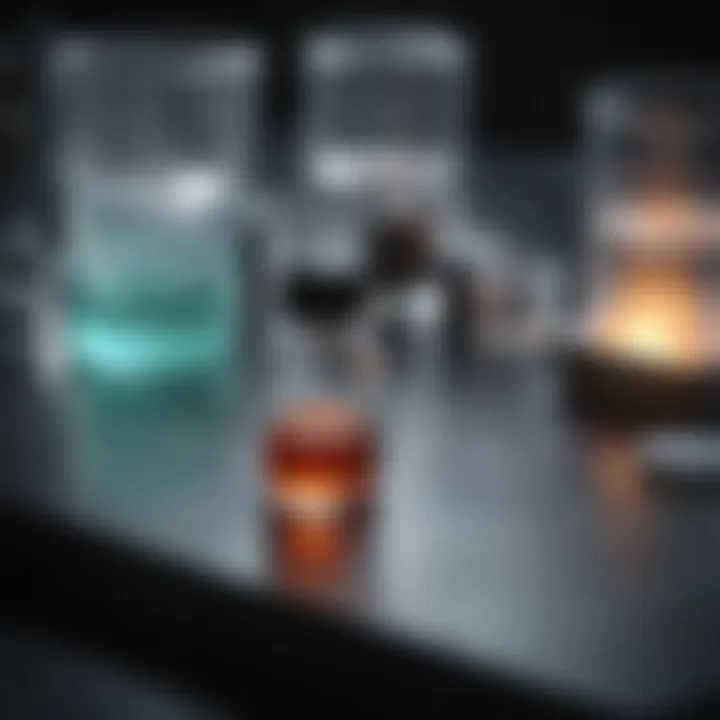
Principles of RT-PCR
RT-PCR stands as a cornerstone in molecular biology, earning its reputation due to the meticulous principles that govern its operation. Understanding these principles not only illuminates the intricacies of the test but also highlights its significance in various fields, notably clinical diagnostics and research. The principles encompass crucial concepts such as reverse transcription, PCR amplification, and the pivotal role of primers and probes. Each of these elements weaves into the fabric of RT-PCR, contributing to its overall effectiveness. Comprehending these principles enables students, researchers, and practitioners to appreciate why RT-PCR is often first in line when it comes to identifying genetic material, particularly in viral infections.
Mechanism of Reverse Transcription
At the heart of the RT-PCR process lies the mechanism of reverse transcription. This step converts RNA into complementary DNA, or cDNA. The process begins when an enzyme known as reverse transcriptase synthesizes cDNA using the RNA template. This step is not just a minor detail; it is integral to ensuring that genetic information from RNA viruses, like SARS-CoV-2, can be analyzed.
Notably, reverse transcription involves several key stages:
- The reverse transcriptase binds to the RNA template.
- It synthesizes the complementary DNA strand by adding nucleotides that pair with the RNA.
- After synthesis, RNase H, another enzyme, degrades the RNA strand, which enables the formation of double-stranded cDNA.
This mechanism is remarkable because it creates a stable DNA product that can then be amplified in subsequent steps of the RT-PCR process. This ability to convert short-lived RNA into stable cDNA opens countless doors for study and diagnosis.
Amplification Process in PCR
Once cDNA has been formed, the process transitions into the amplification phase, essential for generating sufficient quantities of DNA for analysis. In this stage, standard PCR techniques are employed. The cycle begins with denaturation, where the double-stranded cDNA is heated, effectively separating the strands. Following this, the temperature is lowered to allow for annealing of specific primers designed to target regions of interest within the cDNA.
The amplfication occurs through a series of cycles, typically around 25 to 40, where polymerase enzymes then synthesize new strands by extending from these primers, doubling the amount of DNA with each cycle. The beauty of this amplification lies in its exponential nature: a single copy of cDNA can yield millions in just a short span of time.
Role of Primers and Probes
Primers and probes play fundamental roles in the RT-PCR process, ensuring both specificity and sensitivity. Primers are short sequences of nucleotides that serve as starting points for DNA synthesis. They are crucial because they must be designed to perfectly match the target sequence, providing accuracy in amplification.
Probes, on the other hand, add another layer of specificity. Often labeled with fluorescent dyes, these probes hybridize to the target DNA, allowing for real-time monitoring of the amplification process. This labeling not only confirms the presence of the target sequence but also quantifies it, providing information on how much of the target is present in the original sample.
The right design of primers and probes is often the difference between obtaining clear results and facing ambiguous or invalid data. Without this precision, the entire testing process can yield misleading conclusions.
In summary, the principles underlying RT-PCR are essential for its functionality. Each component, from reverse transcription through the amplification process to the roles of primers and probes, must be meticulously understood to utilize RT-PCR effectively in diagnostics and research. Knowledge of these principles empowers professionals in the field to leverage RT-PCR's capabilities fully.
Applications of RT-PCR
The landscape of molecular biology is vast, and among the myriad techniques available, RT-PCR shines brightly as a pivotal tool, particularly in clinical diagnostics and research. Its versatility allows for a host of applications, making it a valuable asset across various fields. This section will unravel the significance of RT-PCR, bringing to light its crucial roles and contributions in understanding diseases and advancing scientific research.
Clinical Diagnostics
In the realm of clinical diagnostics, RT-PCR has carved out a niche that often seems irreplaceable. It is the go-to method for detecting viral infections, allowing for rapid and reliable results. One of the most commendable features of RT-PCR in this context is its ability to specifically identify genetic material from viruses.
Viral Infections
Take, for instance, COVID-19 testing; RT-PCR quickly became synonymous with diagnostics during the pandemic. This method enables laboratories to pinpoint the presence of the SARS-CoV-2 virus within samples, such as nasopharyngeal swabs. The key characteristic that makes RT-PCR particularly suitable for viral infections is its sensitivity; it can detect even minute amounts of viral RNA, which is essential in early diagnosis and managing outbreaks.
However, the unique feature of detecting multiple strains contributes to its popularity. It's streamlined for various viruses like influenza and HIV, providing results that are not only accurate but timely. Yet, it's worth noting that while RT-PCR is reliable, the process requires meticulous handling to avoid contamination, which could lead to false positives—a crucial consideration given the high stakes of viral diagnostics.
Genetic Disorders
On another front, RT-PCR makes significant headway in diagnosing genetic disorders. By identifying specific mutations in genes, this technique assists in detecting conditions like cystic fibrosis or certain types of muscular dystrophy. The advantage of using RT-PCR in this scenario lies in its precision—individuals can receive targeted insights regarding their genetic health.
A remarkable aspect is its capability to analyze multiple genes simultaneously, which saves time and resources. Yet, the intricacies of interpreting these results demand a level of expertise that isn’t always readily available—a drawback that cannot be overlooked. Moreover, the emotional weight of genetic testing often creates a profound impact on individuals and families, necessitating sensitive handling of the information obtained.
Research Applications
Beyond diagnostics, RT-PCR serves as an invaluable tool in various research applications. Its capacity to assess gene expression and contribute to vaccine development is proof of its adaptability and relevance in modern science.
Gene Expression Analysis
Delving into gene expression analysis, RT-PCR allows researchers to quantify the mRNA levels of specific genes, shedding light on various biological processes and disease mechanisms. This analysis is pivotal in areas like cancer research, where scientists can study what genes are overactive or underactive. The ability to derive this data quickly is a key benefit, facilitating swift adaptations in experimental designs based on preliminary results.
One of the unique features of RT-PCR in this regard is its efficacy in quantifying gene expressions in a range of samples, whether from tissue, blood, or cultured cells. Yet, it is not without limitations. The challenge often lies in the normalization of data and ensuring that results are comparable across different experiments—a task requiring diligence and precision.
Vaccine Development
As the world has witnessed, vaccine development has surged into the spotlight, and RT-PCR plays a vital role. It helps in the assessment of immune responses to candidate vaccines, elucidating if they sufficiently stimulate the desired immune pathways. During the development of vaccines for diseases like Zika and, more recently, COVID-19, RT-PCR has been crucial in evaluating how well a vaccine works in eliciting an immune response.
The primary advantage in vaccine research is RT-PCR's ability to deliver rapid feedback on the immunogenicity of vaccine candidates, thereby guiding adjustments in formulations if needed. However, the integration of this technology into the broader context of vaccine efficacy requires careful consideration of various factors, including population diversity and emerging variants, which can complicate interpretations.
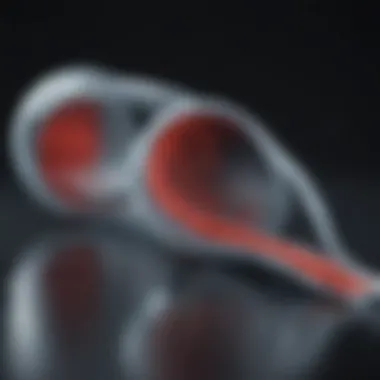
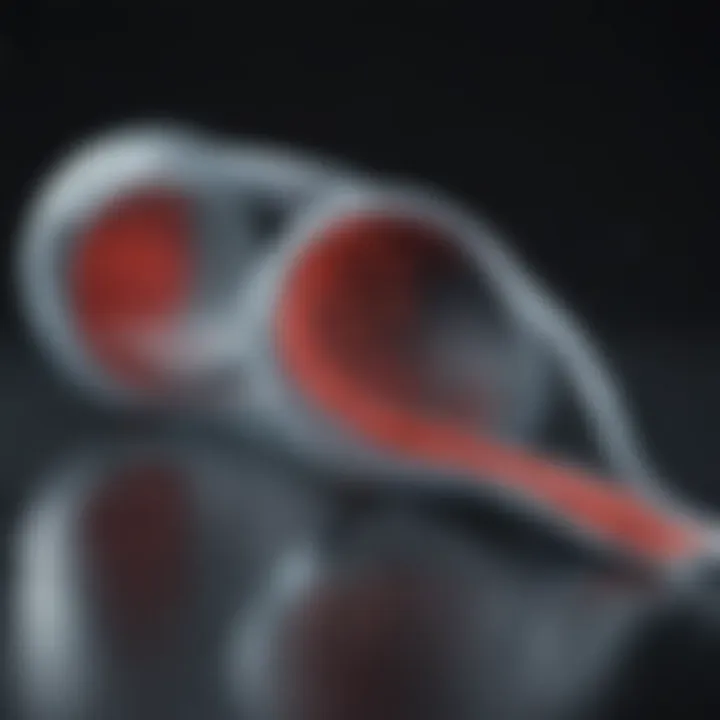
In essence, the applications of RT-PCR encapsulate not just a method of testing but a gateway to understanding and treating diseases better, making it an indispensable component of modern molecular biology.
RT-PCR, with its dynamic applications in clinical and research settings, underscores the significance of this technique in navigating both the challenges of infectious diseases and the complexities of genetic disorders. It showcases the potential for advancing knowledge and improving patient outcomes across the board.
Process of Conducting RT-PCR Tests
The process of conducting RT-PCR tests is a cornerstone in molecular diagnostics. It's not merely a protocol but a carefully orchestrated sequence of events that involve critical choices at every step. These steps ensure that the resulting data is not only reliable but also robust enough to withstand scrutiny from the scientific community and beyond. Here, we delve into the intricate process, discussing the importance, benefits, and essential considerations surrounding this pivotal technique.
Sample Collection Techniques
Effective sample collection is pivotal; if this step is flawed, it can throw everything else off-kilter. A well-collected sample lays the groundwork for accurate results.
Different methods can be employed based on the type of testing needed and the virus or organism in question. Some common techniques include:
- Nasal Swabs: Commonly used for respiratory viruses, where swabs are inserted into the nasal passages.
- Throat Swabs: Useful for testing pathogens, particularly in cases of throat infections.
- Sputum Samples: Collected for respiratory infections, these yield material coughed up from the lungs.
Proper training in collection techniques is crucial to avoid contamination, which can compromise validity. Observing protocols and using sterilized equipment is non-negotiable. Moreover, timestamping and tracking samples enhance traceability, which is utmost in clinical setups.
Preparation of Reagents
Once the samples are collected, attention shifts toward the preparation of reagents. This is where the magic begins; high-quality reagents are the backbone of reliable tests. Each component plays a unique role, from enzymes that facilitate the reaction to buffers that maintain pH levels.
Key reagents include:
- Reverse Transcriptase: Converts RNA into complementary DNA (cDNA), a fundamental step in the RT-PCR process.
- DNA Polymerase: Amplifies the cDNA, leading to the generation of multiple copies of the target region.
- Primers: Short sequences that provide starting points for the DNA synthesis. The design and specificity of primers directly influence test outcomes; they must match the target sequence closely to guarantee fidelity.
Attention to detail during the preparation phase is essential. Even small variations in concentrations can have cascading effects, leading to erroneous results. Standard operating procedures must be diligently followed to maintain consistency.
Thermal Cycling Conditions
Thermal cycling is where the real excitement happens, transitioning samples through various temperatures to encourage both reverse transcription and DNA amplification. The conditions set here can make or break test results.
The typical thermal cycle includes:
- Denaturation: Heating the mixture to around 95°C to separate the DNA strands.
- Annealing: Dropping the temperature to about 55-65°C to allow primers to bind to the target sequences.
- Extension: Raising the temperature to roughly 72°C allows DNA polymerase to extend the new DNA strands.
It's crucial to set the right time and temperature for each phase. This is not a one-size-fits-all; optimization is often necessary based on the specific assay being run. Carefully calibrated equipment is paramount to ensure that temperature changes happen smoothly without overshooting or undershooting.
The finesse required in these procedures underscores the complexity inherent to RT-PCR tests. Ensuring quality at each step helps mitigate misinterpretations of data, thus maintaining confidence in the findings.
Interpretation of RT-PCR Results
Understanding how to interpret RT-PCR results is crucial in determining the presence or quantity of RNA in samples. This section explores what the results mean, their implications, and the decision-making processes that rely on these outcomes. The interpretation of results can significantly impact patient management in clinical settings and guide research directions.
Qualitative Results
Qualitative RT-PCR results provide a straightforward yes or no answer to whether a particular RNA sequence is present in the sample. This binary outcome is particularly beneficial in diagnosing infectious diseases, as it allows for rapid identification of pathogens. For example, in a hospital environment, discovering the presence of a virus like SARS-CoV-2 can inform immediate isolation and treatment protocols.
However, interpreting qualitative results isn't without its complexities. False positives may occur due to contamination or non-specific binding, leading to incorrect conclusions about a patient's health or the status of a sample. It's essential to cross-verify results with clinical symptoms and consider other diagnostic methods.
Researchers also utilize qualitative results, particularly in studying gene expression. By determining if a certain gene is active or inactive, scientists can glean crucial insights into biological processes.
"In RT-PCR, clearly defining positive and negative results can be the difference between clarity and confusion in diagnosis."
Quantitative Results
Quantitative RT-PCR stretches beyond merely stating whether a target RNA is present. It estimates how much of that RNA is in the sample, offering a richer dataset for analysis. This data can be particularly groundbreaking in oncology, where monitoring specific prognostic markers can indicate tumor load or the effectiveness of treatment.
For instance, high levels of viral RNA detected in a patient may signal a severe infection, while low levels could indicate a resolved case. The importance of quantitative results resonates within the research community too. By comparing RNA levels between different samples, researchers can discover trends and patterns that shed light on disease mechanisms.
Yet, one must exercise caution when interpreting quantitative data. Variability in sample quality, reagent efficiency, and run conditions can all affect the numbers derived from the test. Hence, establishing a robust standard curve and using a proper reference gene for normalization are vital processes in gaining accurate results.
By comprehensively understanding both qualitative and quantitative interpretations of RT-PCR results, professionals and researchers can draw more informed conclusions, leading to better clinical outcomes and more profound scientific discoveries.
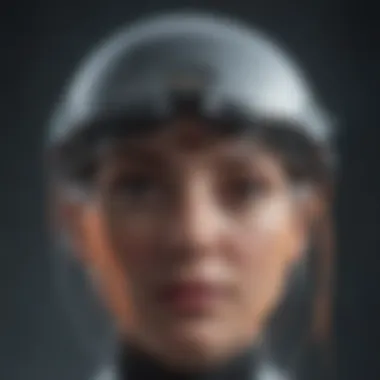
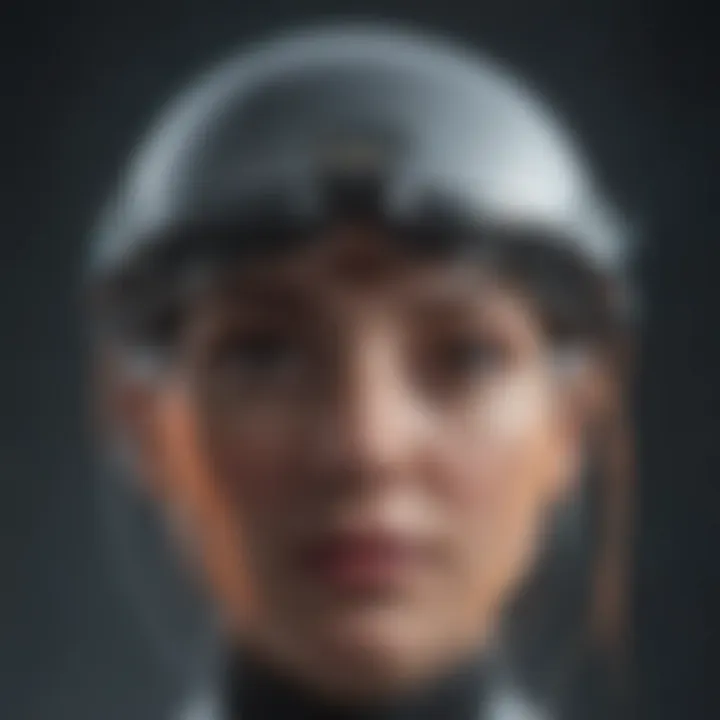
Limitations of RT-PCR
Though RT-PCR has undoubtedly revolutionized the field of molecular diagnostics, understanding its limitations is essential for anyone working in the domain of biology, healthcare, or research. The power of this technology doesn’t come without its challenges. Recognizing these limitations helps practitioners refine their methods and enhance the accuracy of their results, ultimately leading to better patient outcomes and research discoveries.
Sensitivity and Specificity Issues
Sensitivity and specificity are two cornerstones of any diagnostic test. In the context of RT-PCR, sensitivity refers to the test's ability to correctly identify positive cases, while specificity indicates its capacity to correctly recognize negative cases. The balance between these two is crucial for reliable results.
- Low Sensitivity: One major concern is that RT-PCR may sometimes yield false negatives—meaning that the test fails to detect the target RNA in a sample where it does exist. This can arise from several factors:
- Low Specificity: Conversely, low specificity can result in false positive results, wherein the test indicates the presence of RNA that isn’t actually there. This might be due to:
- Sample Quality: If the sample collected is not representative or is of poor quality, the RNA might degrade before it reaches the testing stage.
- Insufficient Reaction Conditions: Problems such as improper temperature settings during the thermal cycling can lead to suboptimal enzyme activation, impacting the amplification process.
- Cross-Reactivity: Primers designed for a specific pathogen may inadvertently bind to sequences from similar organisms, leading to inaccurate results.
- Contaminated Samples: Environmental contamination can introduce extraneous nucleic acids that manifest in the results, misleading healthcare providers into thinking that a viral replication is taking place.
The ramifications of these sensitivity and specificity issues cannot be understated, particularly in clinical settings where the stakes are high. Faulty results may lead to poor treatment decisions, affect public health responses, or even lead to worse disease management outcomes.
Contamination Risks
Contamination presents a significant risk in the RT-PCR workflow. The very nature of PCR relies on amplifying tiny amounts of DNA or RNA, making the process prone to contamination from external nucleic acids. This can stem from a variety of sources:
- Laboratory Environment: Open workspaces, improperly cleaned equipment, and insufficient personal protective equipment can all present contamination hazards.
- Carryover Contamination: Even the smallest residual RNA from prior RT-PCR experiments can contaminate new samples, setting off a chain reaction of false interpretations.
Effective measures to mitigate contamination risks involve stringent practices, such as:
- Using dedicated equipment for RT-PCR to avoid cross-contamination between samples.
- Implementing positive displacement pipettes which reduce the chance of aerosolization and, therefore, potential contamination.
- Maintaining a clearly demarcated workflow where pre-PCR and post-PCR activities are separated to minimize the risk of carrying contamination through the testing process.
"Contamination in RT-PCR is akin to a double-edged sword; it can cut through scientific integrity if not managed properly."
These limitations clarify why RT-PCR should always be interpreted with caution. Rigorous validation of results, coupled with an understanding of potential pitfalls, is essential. Thus, while RT-PCR remains a powerful tool in the arsenal of modern science, its execution and interpretation must be navigated with care.
Future Directions in RT-PCR Technology
The realm of molecular biology is continually evolving, and the future of RT-PCR technology is no exception. With the growing demands for rapid, accurate diagnostic tools, it’s imperative to explore how RT-PCR can be refined and enhanced. The advancements in technology not only bolster the effectiveness of existing RT-PCR protocols but also pave the way for innovative applications that can revolutionize both clinical and research fields.
Advancements in Detection Methods
Moving beyond conventional RT-PCR, new detection methods are emerging to improve sensitivity and efficiency. One of the standout developments in this area involves the application of digital PCR (dPCR). Unlike traditional approaches, dPCR quantifies target nucleic acids across numerous partitions, significantly reducing background noise and enhancing precision. This high-resolution technique is particularly useful in detecting low-abundance targets, such as rare mutations in cancer research or the early identification of viral infections.
Moreover, the introduction of advanced fluorescence detection methods promises to further streamline RT-PCR processes. Utilizing high-resolution imaging systems, researchers can expect real-time visualization of amplification events, which minimizes the time required for result interpretation. This means not only are we speeding up diagnostics but also boosting their accuracy.
Additionally, CRISPR technology is making waves in detection methods. By relying on CRISPR-mediated amplification, it opens up doors to highly specific and robust assays. These CRISPR-based RT-PCR tests are designed to target various pathogens with great specificity, thus broadening the application scope of RT-PCR in both infectious disease outbreaks and public health monitoring.
Integration with Other Technologies
The future of RT-PCR is not just in enhancing its own methods, but also in how it can be integrated with other innovative technologies. One significant avenue for integration lies in the combination of RT-PCR with next-generation sequencing (NGS). By pairing these technologies, researchers can achieve a more holistic view of genomic and transcriptomic landscapes. This amalgamation allows for comprehensive studies on gene expression, mutations, and pathogen diversity — valuable insights that can inform targeted interventions.
On a broader scale, coupling RT-PCR with artificial intelligence and machine learning could lead to a new wave of diagnostic tools. These technologies can analyze vast datasets from RT-PCR results, predicting trends, inferring potential outbreaks, or even suggesting personalized medicine approaches. In essence, leveraging AI could enhance the efficiency of diagnostic processes and result interpretation significantly.
Furthermore, mobile and point-of-care testing is also gaining traction. By integrating RT-PCR into portable devices, realtime diagnostics can be achieved, especially in resource-limited settings. This democratization of technology holds the potential to improve healthcare outcomes globally, allowing for timely interventions in critical situations.
"Emerging technologies like CRISPR and AI are set to change the game for RT-PCR, making it more precise and accessible than ever before."
As we look toward the horizon, the future directions of RT-PCR technology not only promise to address existing limitations but also open doors to entirely new potentials in diagnostics and research. The ongoing innovations will ultimately create tools that are faster, more accurate, and widely applicable in various fields, ensuring that RT-PCR remains at the forefront of molecular diagnostics.
The End
As we wrap up this exploration of the RT-PCR test, it’s clear that its significance cannot be overstated. RT-PCR sits at the intersection of diagnostics and research, providing a robust framework for understanding viral behavior and genetic expression. The reliability of the results generated by RT-PCR can directly influence patient treatment plans and public health responses, making the accuracy of its methodology essential.
Summary of Key Points
To recap what we've covered:
- We introduced the basic principles of RT-PCR, detailing its reliance on reverse transcription and amplification processes.
- The discussion also highlighted the test's multifaceted applications in both clinical diagnostics and research. Whether it’s diagnosing viral infections or analyzing gene expression, RT-PCR stands out for its versatility.
- We explored the procedural aspects of RT-PCR, examining each step from sample collection to result interpretation, emphasizing best practices that ensure integrity.
- Limitations, including sensitivity and contamination risks, were addressed, shedding light on potential pitfalls researchers and clinicians face.
- Lastly, we ventured into the future scope of RT-PCR technology, alluding to advancements and integrations that promise to enhance its capabilities.
Significance of RT-PCR in Science
RT-PCR has firmly established its place in the scientific community. It’s not merely a technique but a cornerstone of modern molecular biology. Researchers leverage its capabilities to delve into the minutiae of how different organisms respond to pathogens, which is crucial in today’s fight against infectious diseases.
Moreover, RT-PCR's quantitative aspect sheds light on gene expression levels, allowing scientists to draw connections between genetic processes and phenotypic outcomes. The proliferation of individualized medicine owes much to technologies like RT-PCR, which enables tailored treatment approaches based on specific genetic information. As we stand at the crossroads of innovation in molecular diagnostics, RT-PCR's role is set to expand, fostering breakthroughs in our understanding of biology and medicine.
Emphasizing the need for continual advancements, the future of RT-PCR looks promising not just in labs but in practical applications that could change the landscape of healthcare.