Exploring Activated Carbon: Properties and Applications
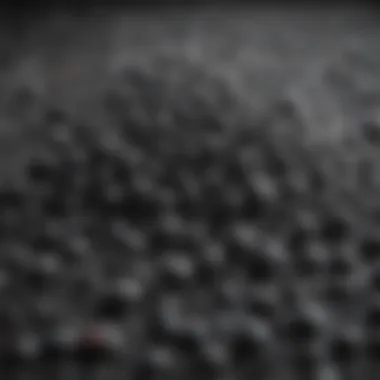
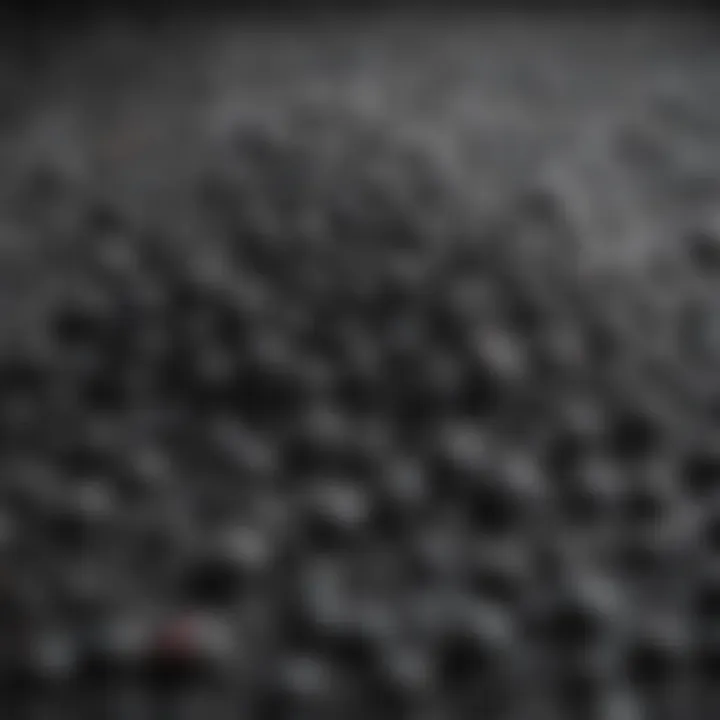
Intro
Activated carbon is gaining prominence in numerous fields due to its versatility as an adsorbent. This material's unique structure enhances its ability to attract and hold molecules from gases and liquids. Over the years, researchers have been exploring its properties, applications, and the future of activated carbon, leading to significant advancements that broaden its relevance.
In this article, we will delve into the defining characteristics of activated carbon, analyze its various applications across different industries, and discuss the factors influencing its effectiveness as an adsorbent. As we navigate through these topics, it becomes clear how important activated carbon is in tackling contemporary challenges, particularly those relating to environmental sustainability.
Research Overview
Understanding activated carbon begins with examining its properties. This section outlines key findings from recent studies while summarizing the methodologies employed in these investigations.
Summary of Key Findings
Research indicates that activated carbon possesses high surface area and porosity, which are critical for its adsorptive capabilities. These properties allow activated carbon to effectively remove impurities or harmful substances from air or water, making it invaluable in water treatment plants, air purification systems, and even in medical applications for toxin absorption.
Methodologies Employed
Various methodologies are used in studying activated carbon. Common techniques include adsorption isotherms and kinetic studies.
- Adsorption Isotherms: This examines how different concentrations of contaminant affect the adsorption process at equilibrium.
- Kinetic Studies: These investigate how quickly activated carbon can adsorb substances over time.
Such approaches provide comprehensive insights into activated carbon's behavior under various conditions, making it easier to tailor its applications.
In-Depth Analysis
An extensive evaluation of activated carbon involves analyzing specific results of recent studies and comparing them with prior findings. The following sections detail this analysis.
Detailed Examination of Results
Recent studies show that the effectiveness of activated carbon can be influenced by factors like temperature, pH, and the presence of competing contaminants. For instance, at elevated temperatures, some carbon structures can lose their adsorptive capacity.
Moreover, innovations in activated carbon production, including chemical activation and steam activation, have been shown to improve surface area and pore size significantly.
Comparison with Previous Studies
When comparing new data to older research, it is evident that the enhancements in production techniques have led to significant advancements. Earlier studies often suggested limitations on the use of activated carbon due to poor adsorption rates with certain molecules. However, newer techniques have yielded improved outcomes, thereby making activated carbon more versatile.
In summary, the ongoing research regarding activated carbon demonstrates its potential in various applications, helping tackle pressing environmental and health issues. The outlook for future innovations suggests further enhancements in its efficacy and range of uses, solidifying its place as a vital material.
Prelims to Activated Carbon
Activated carbon serves as a pivotal substance within various scientific domains, particularly in the realm of adsorption. This section will delve into its significance, defining what activated carbon is, exploring its composition, and tracing its historical roots. Understanding activated carbon is essential, as it has broad applications that span environmental, industrial, and medical fields.
Definition and Composition
Activated carbon is a form of carbon that has been processed to create a vast network of pores. This unique structure grants it an exceptionally high surface area, which can reach up to 3000 square meters per gram. Such characteristics make it ideal for various adsorption processes. The material can be derived from several sources, including coconut shells, wood, and coal.
The composition of activated carbon primarily includes carbon, but it may also contain trace elements, such as hydrogen, oxygen, and nitrogen, depending on the precursor material and activation method used. The pores within activated carbon can vary in size, which allows it to adsorb a wide range of molecules, making it versatile and effective for specific applications.
History of Activated Carbon Usage
The utilization of activated carbon can be traced back several centuries. Early records suggest that ancient Egyptians used forms of carbon to purify water. However, it was not until the late 19th century that scientists began to explore the material systematically.
During the industrial revolution, activated carbon gained significant recognition for its ability to remove impurities from air and water. Its adoption in medical applications occurred with advances in research, leading to its use in treating poisonings and drug formulations. Today, the importance of activated carbon continues to evolve with emerging environmental issues and technological advancements, marking its journey from a historical curiosity to a cornerstone of modern adsorption technologies.
"Activated carbon's journey from ancient Egypt to modern science represents not just a material evolution but also a testament to human ingenuity in solving complex problems."
In summary, activated carbon's definition, composition, and historical evolution establish a solid foundation for understanding its critical role in various applications. The subsequent sections will elaborate on its physical and chemical properties, mechanisms of adsorption, and the diversity of its applications.
Physical and Chemical Properties
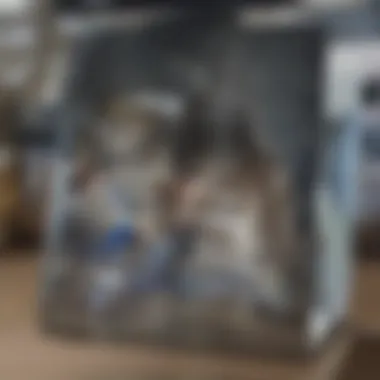
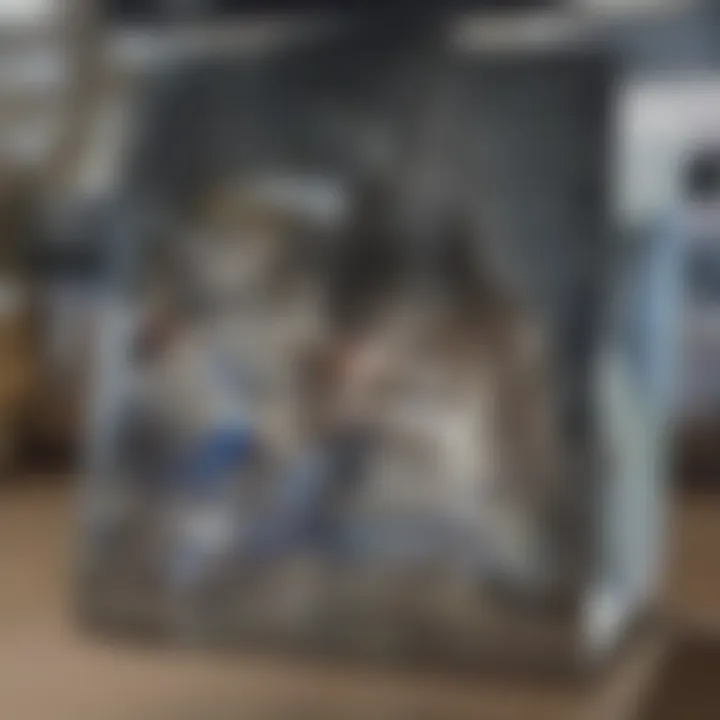
The physical and chemical properties of activated carbon are fundamental to its role as an adsorbent. These properties directly influence its efficacy in various applications, from environmental mitigation to industrial processes. Understanding the nuances of these characteristics helps to elucidate why activated carbon continues to be a preferred material in adsorption technologies.
Surface Area and Porosity
The surface area of activated carbon is critical. With a high surface area that can reach over 1000 mΒ²/g, activated carbon provides ample space for adsorption. The porosity of the material allows for the accommodation of various molecules, making it suitable for adsorbing a wide range of contaminants. Porous structure is not just about having holes; it determines how effectively activated carbon can trap substances. Smaller pores tend to adsorb smaller molecules, while larger pores can accommodate bulkier substances. Thus, porosity can be tailored for specific applications, enhancing the operational efficiency.
Chemical Composition and Structure
The chemical composition of activated carbon varies based on its source material and the activation process. Primarily, it consists of carbon, which forms the backbone of its structure. The arrangement of carbon atoms leads to the development of functional groups on the surface. These groups can interact with different chemicals, allowing activated carbon to bind a variety of contaminants. This versatility is one reason why activated carbon is so successful in various fields such as environmental remediation and pharmaceuticals.
Impact of Activation Processes
Physical Activation
Physical activation involves the use of heat in a gaseous atmosphere, commonly steam. This method creates porosity through the thermal decomposition of the precursor material. A key characteristic of physical activation is the retention of most of the carbon structure while enhancing porosity. This makes it a beneficial choice for applications requiring high adsorption capacity. However, one disadvantage can be the time-consuming nature of this process compared to chemical activation, which may limit its scalability in some cases.
Chemical Activation
Chemical activation involves treating the precursor material with chemicals, such as potassium hydroxide or phosphoric acid, at relatively lower temperatures. This method not only enhances the pore structure but also allows for finer control over the surface chemistry of the carbon. A significant advantage of chemical activation is that it produces activated carbon with a high surface area in a shorter time frame than physical activation. However, it does come with the downside that residual chemicals might affect safety and regulatory compliance in certain applications.
Understanding these physical and chemical properties is essential for optimizing activated carbon usage in various applications, paving the way for more effective technologies.
The interplay of these factors determines the specific uses of activated carbon in various sectors, contributing to its enduring significance and potential for future innovations.
Mechanisms of Adsorption
Understanding the mechanisms of adsorption is essential for comprehending why activated carbon is so effective as an adsorbent. This section explores the specific processes involved in adsorption, namely physisorption and chemisorption, as well as the factors that influence the efficiency of these mechanisms. The insights gained here provide significant context for the applications of activated carbon across various fields.
Types of Adsorption Processes
Physisorption
Physisorption refers to the adsorption process that occurs through weak van der Waals forces. In this process, the interaction between the adsorbate and the adsorbent is dominated by physical forces rather than chemical bonds. One key characteristic of physisorption is its reversibility, meaning that the adsorbate can detach from the adsorbent without requiring significant energy input. This property makes physisorption favorable in applications where temporary capture of contaminants is needed, like in air and water filtration.
The unique feature of physisorption is its relatively low energy requirement for both adsorption and desorption compared to other processes. However, despite its beneficial aspects, the drawbacks include a generally lower capacity for higher contaminant concentrations, which limits its use in certain scenarios.
Chemisorption
Chemisorption involves the formation of strong chemical bonds between the adsorbent and the adsorbate. This process typically entails a higher energy requirement since chemical bonds are stronger than the van der Waals interactions at play in physisorption. A key characteristic of chemisorption is that it usually results in more significant and often irreversible adhesion, making it suitable for applications requiring more permanent capture of pollutants.
One unique feature of chemisorption is its ability to facilitate specific interactions based on the chemical structure of both the adsorbent and adsorbate. However, the main disadvantages lie in the energy costs associated with breaking these strong bonds during the desorption phase. Thus, while chemisorption is effective for select applications, it may not always be practical for scenarios needing flexibility.
Factors Influencing Adsorption Efficiency
Temperature
Temperature plays a vital role in the adsorption process. As the temperature increases, the kinetic energy of the molecules improves, which can enhance adsorption under certain conditions. It is essential to note that for physisorption, higher temperatures may lead to reduced efficiency as the weaker interactions can be easily disrupted. Conversely, chemisorption may benefit from elevated temperatures as it can increase the reaction rates. Thus, temperature is an influential factor in choosing the appropriate adsorption method, depending on the specific application.
pH Level
The pH level of a solution can greatly affect adsorption efficiency, particularly in ionic contaminants. Many adsorbents, including activated carbon, exhibit varying surface charges based on the pH level. This characteristic affects the interaction between the adsorbate and adsorbent. A favorable pH can enhance the adsorption of charged species, while a less favorable pH may inhibit the process. Therefore, understanding the dependence of adsorption on pH is critical for applications in wastewater treatment and chemical processing.
Concentration of Contaminants
The concentration of contaminants in a solution is another significant factor influencing adsorption efficiency. Higher concentrations can enhance the driving force for adsorption, leading to better performance. However, this relationship can also lead to saturation of the adsorbent sites. Once these sites are occupied, further increases in concentration do not result in additional performance gains. Therefore, balancing the concentration of contaminants with the available adsorbent capacity is crucial for optimizing the use of activated carbon in various applications.
The effectiveness of adsorption processes is not merely determined by the properties of activated carbon, but significantly influenced by environmental conditions such as temperature, pH, and concentration of contaminants.
In summary, the mechanisms of adsorption play a fundamental role in the applications of activated carbon. Physisorption and chemisorption exhibit distinct characteristics that make them suitable for specific use cases. The factors influencing these processes highlight the need for careful consideration when implementing activated carbon in various domains.
Applications of Activated Carbon
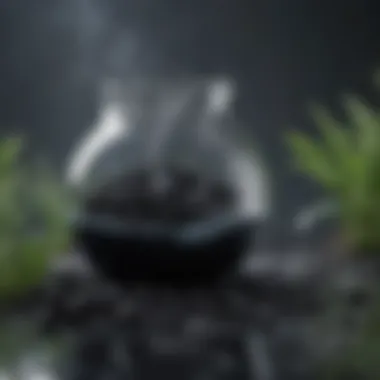
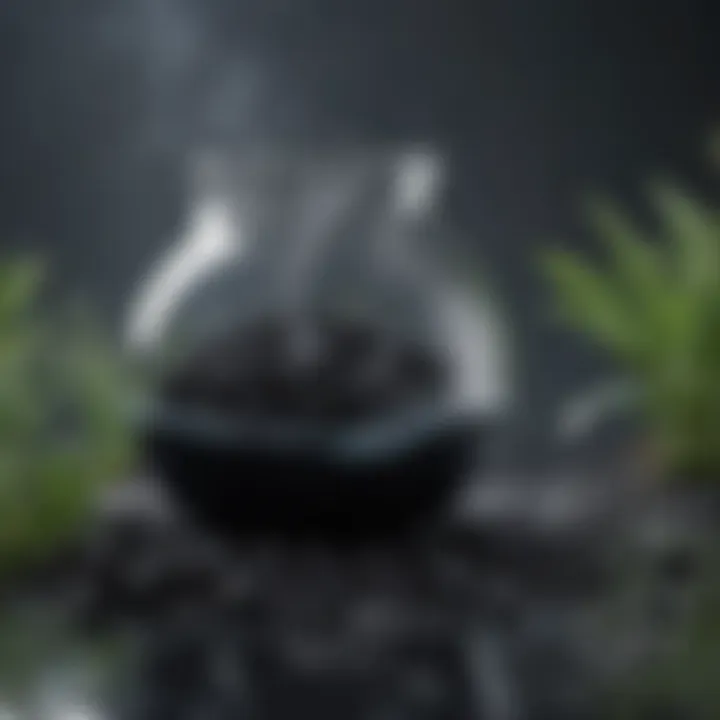
Activated carbon holds significant importance across various fields, primarily due to its unique ability to trap contaminants and impurities. Its application spans environmental, industrial, and medical domains, showcasing the versatility and efficacy of activated carbon as an adsorbent. Understanding its various applications helps in appreciating its value in addressing several real-world challenges. By examining the specific uses of activated carbon, we can identify the benefits and considerations that underline its relevance today.
Environmental Applications
Water Treatment
Water treatment is a critical application of activated carbon. It effectively removes organic compounds, chlorine, and suspended solids from water. The key characteristic of activated carbon in water treatment is its high surface area, allowing for extensive interaction with pollutants. This feature makes it a popular choice for municipal water systems, providing safe drinking water and protecting public health.
The unique adsorptive properties of activated carbon enable it to handle a wide range of contaminants. Its advantages include the ability to improve taste and odor as well as remove harmful substances like pesticides and volatile organic compounds. However, one must also consider its limitations, such as the need for periodic replacement or regeneration, which can incur additional costs and environmental impacts.
Air Purification
Air purification is another vital application. Activated carbon filters capture pollutants, volatile organic compounds, and odors in residential and industrial settings. The primary characteristic that highlights this technology is its efficacy in trapping harmful compounds without releasing them back into the air. This makes activated carbon a beneficial choice for maintaining indoor air quality.
A unique feature of air purification applications is the scope of use, from home air filters to large-scale industrial applications. The notable advantage is its reliability and relatively low maintenance. However, one disadvantage is that its effectiveness can be reduced once the carbon pores fill up, necessitating timely replacement.
Soil Remediation
Soil remediation involves the use of activated carbon to treat contaminated soils. This application focuses on the adsorption of pollutants such as heavy metals and organic contaminants. The key characteristic is its ability to immobilize hazardous substances, thereby protecting ecosystems and human health. Utilizing activated carbon in soil treatment is a proactive measure against environmental degradation.
Its unique ability to sequester contaminants provides a clear advantage in restoring degraded lands. Nevertheless, it also presents challenges. For example, the long-term effectiveness of activated carbon in soil can vary based on the type of contaminant, and its implementation may require additional interventions for full effectiveness.
Industrial Applications
Food and Beverage Processing
In the food and beverage industry, activated carbon is utilized for decolorizing, deodorizing, and purifying various products. The key characteristic is its ability to remove impurities while minimizing the impact on flavor and quality. This aspect makes it a popular choice for producers aiming to enhance product safety and quality.
The unique feature in food processing is its versatility to be used in different stages, from raw material preparation to final product refinement. The advantages include improving product integrity and consumer safety. However, there are disadvantages regarding cost and potential regulatory challenges that may arise based on local guidelines.
Pharmaceuticals Manufacturing
Activated carbon plays a vital role in pharmaceuticals manufacturing, mainly in the purification and recovery of active pharmaceutical ingredients. The primary characteristic here is its high adsorptive capacity, which is essential for ensuring the purity and safety of pharmaceuticals. This application is highly beneficial for pharmaceutical industries striving to meet stringent quality standards.
A unique feature is its ability to facilitate the removal of impurities without affecting the active ingredients. The advantages include cost-effectiveness in achieving high purity levels. However, the disadvantages might include the need for extensive testing and validation to confirm efficacy in specific applications, which can prolong development timelines.
Medical Applications
Drug Delivery Systems
In medical technology, activated carbon is emerging in drug delivery systems. It serves as an adsorbent for controlled release of medications. The key characteristic of using activated carbon in this context is its high surface area, which aids in capturing and gradually releasing drugs into the bloodstream. This feature is particularly beneficial in enhancing the efficacy of medications over time.
The unique advantage of utilizing activated carbon in drug delivery is its potential to optimize therapeutic outcomes with minimal side effects. However, there may be challenges regarding compatibility with specific drugs, necessitating extensive testing and research.
Poisoning Treatment
Activated carbon is widely recognized for its role in treating poisoning cases. It is effective in adsorbing a variety of toxic substances, thus preventing their absorption in the gastrointestinal tract. The key characteristic of activated carbon in these scenarios is its rapid action and effectiveness in emergency medical settings. This makes it a crucial component in first aid for poisoning incidents.
A unique feature is its ability to be administered intravenously or orally, providing flexibility in treatment protocols. However, potential disadvantages include limited effectiveness for certain poisons and the risk of aspiration if not administered cautiously. The need for medical supervision is critical in ensuring safe and effective treatment.
Activated carbon serves myriad applications, demonstrating its versatile role in enhancing health, safety, and environmental sustainability.
Environmental Impact and Sustainability
Activated carbon plays a crucial role in various applications, making it essential to scrutinize its environmental impact and sustainability. Understanding these elements allows for more informed choices regarding its use, addressing the growing concerns of ecological footprints and resource depletion that accompany the production and application of activated carbon.
The importance of focusing on environmental sustainability lies in the necessity to balance technological advancement and environmental preservation. Activated carbon is derived from carbonaceous materials such as wood, coal, or coconut shell. The extraction and processing of these materials can lead to deforestation, soil erosion, and resource depletion if not managed responsibly. Consequently, assessing the life cycle of activated carbon from raw materials to disposal is vital for evaluating its overall environmental footprint.
Life Cycle Assessment of Activated Carbon
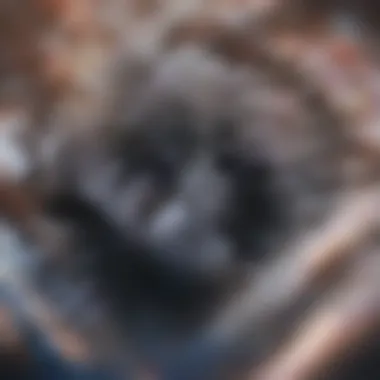
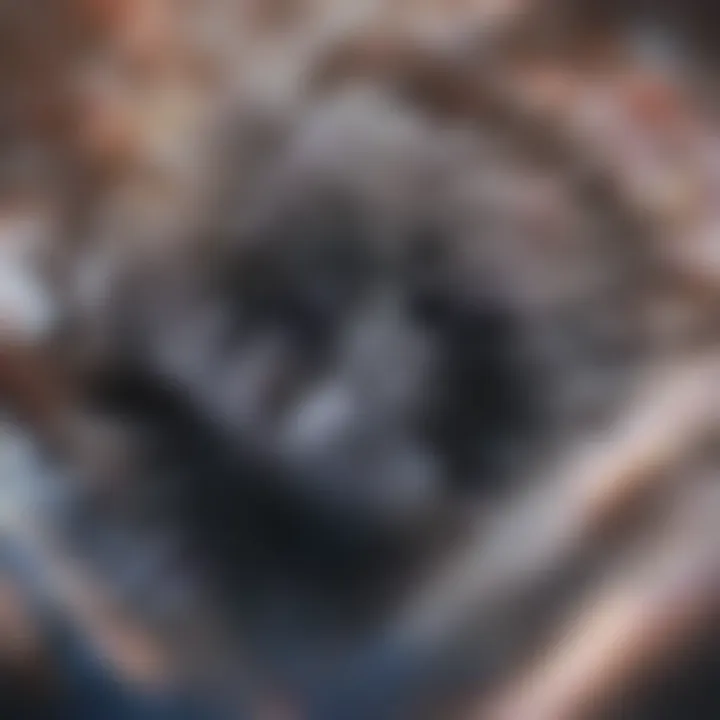
A Life Cycle Assessment (LCA) provides an analytical framework to evaluate the environmental impacts associated with all stages of a product's life, including extraction, production, use, and disposal. The LCA of activated carbon highlights several key considerations:
- Raw Material Extraction: The sourcing of materials can significantly affect ecosystems. Sustainable sourcing practices, such as utilizing agricultural waste, can mitigate adverse environmental effects.
- Manufacturing Processes: The production of activated carbon often involves significant energy consumption and greenhouse gas emissions. Employing more efficient production techniques can reduce these emissions.
- Utilization: Activated carbon can effectively reduce pollutants in air and water. However, it is essential to consider how effective its use is relative to alternative methods to determine its net environmental benefit.
- End-of-Life Management: Disposal options vary, with incineration and landfilling as common methods. Developing procedures for regeneration and recycling can lessen the environmental impact significantly.
Evaluating each stage of the activated carbon life cycle ensures that businesses and consumers can measure its sustainability effectively.
Sourcing and Regeneration
Sourcing strategies for activated carbon should focus on sustainability and minimal environmental disruption. Selecting renewable or waste-based feedstocks, such as agricultural residues or by-products from other industries, is a step in the right direction. This practice not only conserves natural resources but also promotes a circular economy.
Regeneration of activated carbon is another essential aspect of its sustainable use. This process involves cleaning and reactivating spent carbon, extending its lifespan, and reducing the demand for new materials. There are various methods for regeneration, including:
- Thermal Regeneration: Involves heating the spent carbon to remove adsorbed contaminants, restoring its adsorptive capacity.
- Chemical Regeneration: Utilizes chemicals to regenerate the adsorbent, which can often be more efficient for specific contaminants.
- Biological Regeneration: Emerging methods involve using biological agents to break down contaminants, promoting a more environmentally-friendly approach.
By implementing effective sourcing and regeneration, the environmental impact of activated carbon can be minimized. Companies that adopt these practices are more likely to achieve sustainable operations, reducing their overall ecological footprint.
Sustainable practices in activated carbon sourcing and regeneration are crucial not only for environmental preservation but also for economic viability in the long term.
Through focused strategies in sourcing and a commitment to regeneration, the activated carbon industry can align itself with broader sustainability goals, making significant strides towards a more responsible and eco-friendly approach.
Future Directions in Activated Carbon Research
The future directions in activated carbon research represent a vital area of investigation. As environmental and health challenges become increasingly complex, activated carbon's role needs further exploration and innovation. Understanding this trajectory informs various stakeholders from policymakers to industry leaders and researchers.
Research is moving towards optimizing production techniques, which will enhance the efficiency and effectiveness of activated carbon as an adsorbent. Innovations in this area can lead to more sustainable practices, reducing waste and improving the material's performance in various applications.
Another key focus is the development of new, innovative uses for activated carbon. By exploring emerging technologies and applications, researchers can unlock new potential for tackling pressing issues such as air and water pollution, thereby expanding activated carbonβs impact on public health and environmental restoration.
"Continuous advancements in activated carbon research are essential for sustainable solutions in pollution control and resource management."
The interplay between these innovations and applications may contribute significantly to energy efficiency, economic viability, and environmental impact. Therefore, such directions in research are not just desirable; they are necessary for progress in various fields.
Innovations in Production Techniques
The methods for producing activated carbon are evolving. Traditional techniques often involve high-energy processes that can be wasteful. Therefore, researchers are now investigating alternative materials and processes that are more energy efficient.
For instance, using agricultural by-products, such as coconut shells and wood biomass, presents an opportunity. This not only utilizes waste materials but also provides a sustainable source of activated carbon. Techniques such as microwave-assisted activation are gaining attention for their potential to reduce energy consumption significantly.
Some emerging innovations in production techniques include:
- Modification of Activation Methods: Fine-tuning physical and chemical activation methods to enhance surface area and porosity.
- Nanotechnology Integration: Utilizing nanomaterials to create activated carbon with improved characteristics.
- Continuous Production Processes: Shifting from batch production to continuous systems for more consistent quality and efficiency.
These advancements can lead to higher quality activated carbon and reduce costs associated with production.
Emerging Applications and Technologies
The versatility of activated carbon is likely to expand with advancements in technology. Industries are discovering novel applications, moving beyond traditional uses in filtration and purification.
In the field of medicine, activated carbon is being explored for its role in drug delivery systems. Its porous structure can facilitate targeted delivery, improving the efficacy of treatments. Additionally, in the environmental sector, activated carbon is being utilized for capturing greenhouse gases, a crucial step in addressing climate change.
Some notable emerging applications include:
- Energy Storage: Activated carbon is increasingly seen as a viable material for supercapacitors, enabling efficient energy storage solutions.
- Contaminant Removal: New forms of activated carbon are designed for selective adsorption of specific pollutants, enhancing remediation efforts in water treatment.
- Air Quality Management: Enhanced activated carbon filters for indoor air purification, mitigating effects of volatile organic compounds and other harmful substances.
As research progresses, the interactions between activated carbon and advanced technologies such as artificial intelligence and big data will shape the future landscape of applications, refining its use in various sectors.
Each advancement in production techniques and applications not only emphasizes the adaptability of activated carbon but also its pivotal role in facilitating sustainable solutions to complex global challenges.
The End
The discussion surrounding activated carbon as an adsorbent is vital for understanding its multifaceted benefits and applications in various fields. Activated carbon's exceptional properties, including its high surface area and porous structure, enable it to effectively capture pollutants and toxins, making it an indispensable material in environmental management and industry.
Summing Up the Role of Activated Carbon
Activated carbon serves a crucial role in numerous applications, especially in water and air purification. Its effectiveness in adsorbing undesirable substances directly influences the quality of our environment and human health. In industrial settings, it helps ensure product purity while minimizing waste. The medical use of activated carbon for drug delivery and treatment of poisoning underscores its versatility and importance in protecting individuals against harmful substances.
As the demand for sustainable practices grows, the regenerative features of activated carbon present an opportunity for reducing waste and enhancing sustainability efforts. Further research focusing on innovative production techniques and exploring new applications will only reinforce the significance of activated carbon in addressing contemporary challenges in pollution and health. This conclusion encapsulates the critical role activated carbon plays, emphasizing that ongoing research and development will be essential for maximizing its potential in future applications.