DNA Sample Storage: Methods, Challenges, and Innovations
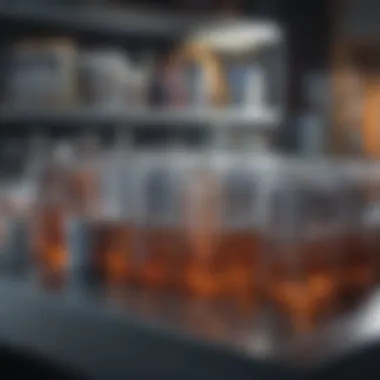
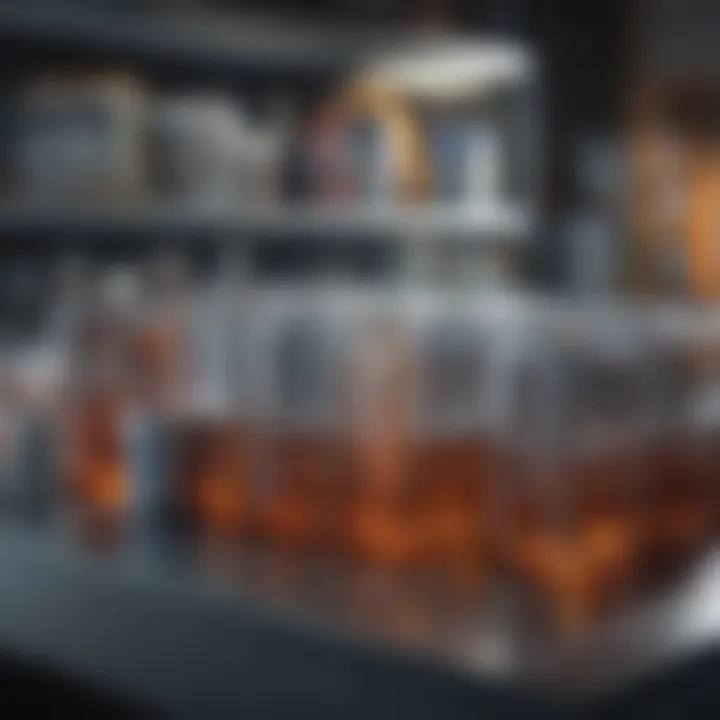
Intro
The preservation of DNA samples is pivotal in numerous fields, be it for forensic analysis, genetic research, or ancestral studies. As we stand on the cusp of significant advancements in genetic technology, understanding the protocols involved in storing DNA is of utmost importance for professionals, researchers, educators, and students alike. For the effective usage of such samples, knowing the best practices in storage can be a game changer.
In this article, we will delve into the intricacies of DNA storage methods, explore the challenges faced, and look ahead at potential future directions in the field. We will discuss optimal preservation conditions, delve into material considerations, and underscore the importance of maintaining sample integrity. Furthermore, we will touch upon the regulatory aspects that govern DNA storage, allowing readers to grasp the full landscape of this critical area in biological research.
Research Overview
Summary of Key Findings
Throughout our exploration, several key findings emerge on the efficacy of DNA storage:
- Temperature Control: Cold storage is indispensable. Freezing at -20°C or -80°C ensures sample stability and longevity.
- Storage Medium: The choice of storing medium plays a role. High-quality tubes or cryovials contribute significantly to preventing contamination and degradation.
- Humidity Levels: Moisture can pose a serious threat to DNA integrity, making desiccation protocols critical during storage.
- Documentation: Proper labeling and meticulous record-keeping cannot be overstated. They are essential to avoid errors in retrieval.
Methodologies Employed
In gathering this information, various methodologies were put into practice, including a detailed review of literature, laboratory experiments, and interviews with experts in the field. The literature review provided a foundation, detailing the traditional and innovative techniques employed in DNA storage. The experimental procedures involved observing the long-term effects of different storage conditions on DNA samples. Expert interviews shed light on practical challenges and advancements in storage technology.
In-Depth Analysis
Detailed Examination of Results
By employing varied storage techniques, it became clear that certain methods surpass others concerning sample integrity. For instance, DNA stored under optimal freezing conditions showed fewer signs of degradation over time. Conversely, samples that faced fluctuations in temperature and humidity often resulted in compromised integrity. Ensuring consistent conditions proved crucial. The analysis revealed that utilizing specific plastics for containers can also serve an important purpose; using materials like polypropylene can minimize the risk of DNA-binding issues that may arise from other materials.
Comparison with Previous Studies
When juxtaposed with previous studies, our findings affirm long-held beliefs while also challenging some outdated practices. For instance, earlier research suggested that room temperature storage might be viable for short durations. Our examination, however, indicates this risks significant sample degradation. Comparatively, the protection offered by extreme cold storage environments is consistent across multiple studies, reinforcing the traditional view that stability is paramount.
"In nucleotide preservation, even the slightest oversight can yield unforeseen consequences, underscoring the importance of rigorous storage protocols."
Prelude to DNA Sample Storage
Storing DNA samples is a critical aspect of modern science, bridging the gap between biological inquiry and practical application. As our understanding of genetics advances, so does the need for effective storage methods that can safeguard these samples for long-term use. This introduction outlines why proficient DNA storage protocols are indispensable in both research and medical contexts
*
Importance of Storing DNA Samples
The value of biobanking, notably DNA sample storage, cannot be overstated. For researchers, the ability to access high-quality samples is what helps fuel new discoveries in genomic medicine and evolutionary biology. Each sample is a key that can unlock important insights into diseases, heredity, and biological diversity.
Moreover, DNA samples serve as a foundation for various personal applications, such as genetic testing, paternity testing, and forensic investigations. In legal contexts, the integrity of DNA evidence plays a vital role in criminal justice proceedings. A mishandled sample could mean the difference between acquittal and conviction, showing that storing DNA samples isn’t just a matter of efficiency but one of ethical responsibility.
"In the world of research, the smallest oversight in sample storage can mean significant setbacks in time and resources."
From environmental studies to agriculture, as well, DNA storage enables ongoing research into biodiversity, plant genetics, and potential conservation efforts. Without adequate storage measures, important biological materials could go to waste.
*
Historical Context
The practice of preserving biological materials has a deep-rooted history. As far back as the early 20th century, scientists like Thomas Morgan used Drosophila, the fruit fly, to study inheritance patterns. Those early days laid the groundwork for what would blossom into today’s sophisticated DNA storage methods.
By the 1950s, with the double helix structure of DNA discovered by Watson and Crick, it became apparent that samples could be leveraged for more than just understanding genetic inheritance. Research moved from purely observational to actively manipulating and analyzing DNA.
Fast forward to the late 20th century, and the rise of biotechnology saw the establishment of biorepositories. These facilities were designed specifically to store and manage biological samples, including DNA, under optimized conditions. Today, advancements in technology and a deeper understanding of DNA degradation mechanisms have prompted ongoing refinement of storage techniques. As these methodologies evolve, the significance of effective DNA sample storage continues to rise, reflecting a symbiotic relationship between scientific progress and methodology.
Fundamental Principles of DNA Preservation
The preservation of DNA is a cornerstone of genetics, biotechnology, and forensic science. Understanding the fundamental principles of DNA preservation is paramount for any laboratory or research facility aiming to maintain the integrity of their specimens over time. These principles form the bedrock of successful storage protocols, ensuring that genetic information can be reliably accessed for future analysis.
One of the central tenets in DNA preservation is molecular stability. DNA's structure can be highly sensitive to external conditions; it can degrade if not stored correctly. Factors like temperature fluctuations, humidity, and light exposure can significantly impact the molecular structure of DNA. Thus, appropriate use of storage conditions can protect against decomposition, ensuring that samples remain viable for future studies.
Another crucial aspect is the environmental conditions in which DNA is kept. Variations in temperature, humidity, and exposure to light can either enhance or undermine molecular stability. Each of these variables brings its own set of characteristics that can be both advantageous and detrimental depending on the specific storage scenario.
Understanding these principles allows for better design of storage facilities and strategies, ensuring the greatest possible longevity for DNA samples.
Molecular Stability
Molecular stability refers to the ability of the DNA structure to remain intact over time. This stability is of utmost importance because even slight alterations can lead to severe impacts on research outcomes. When DNA is subjected to unfavorable conditions, it can undergo hydrolytic damage, oxidative stress, or physical fragmentation. This degradation compromises the DNA’s usability for sequencing, cloning, or forensic analysis.
In this context, employing practices that shield DNA from stressors is beneficial. For instance, the use of protective solutions or carriers can enhance the resilience of samples against degradation processes. This proactive approach can help keep samples in optimal conditions while extending their life span.
Environmental Conditions
Temperature
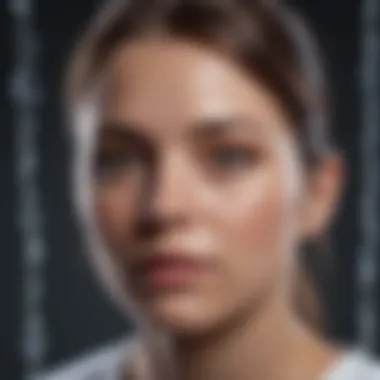
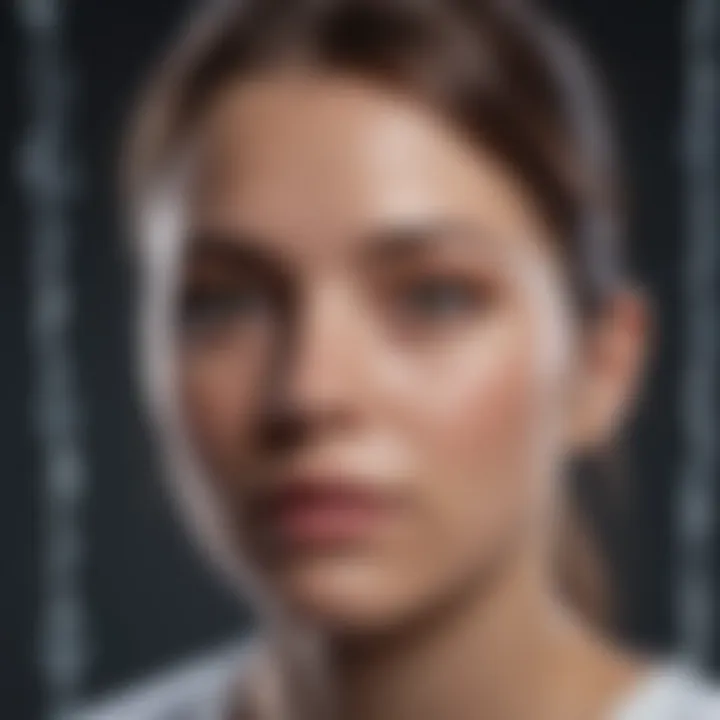
Temperature plays a pivotal role in preserving DNA integrity. Keeping samples at a consistently low temperature helps minimize the molecular motion of DNA, reducing the likelihood of reactions that lead to degradation. Typically, ultra-low temperatures around -80°C are optimal for long-term storage. This cold environment is favored because it essentially halts biochemical processes that could otherwise contribute to sample deterioration.
However, there are unique features to be aware of regarding temperature management. While ultra-low temperatures are beneficial, transitioning samples back to room temperature can introduce risks, such as potential condensation within storage containers which may introduce moisture. Moreover, short-term storage at temperatures around -20°C is often used for immediate use applications but is not suitable for extended periods.
Humidity
Another environmental factor, humidity, is equally critical in the storage of DNA samples. High humidity can promote hydrolysis, leading to degradation of nucleic acids. On the other hand, overly dry conditions can also be detrimental, causing the samples to become brittle. Thus, maintaining an optimal range between 30% to 50% relative humidity can be beneficial.
The key characteristic of humidity control is ensuring a balanced environment within storage facilities. By utilizing desiccation agents or specialized containers, researchers can optimize the conditions conducive to preserving DNA integrity over time.
Light Exposure
Light exposure is an often-overlooked factor in DNA storage. UV light, in particular, can induce damage by causing photochemical reactions within the DNA molecule. Such interactions may lead to mutations and fragmentation, undermining the quality of samples when retrieving them for analysis.
The primary attribute to consider here is the necessity of dark or opaque storage solutions. Protecting DNA samples from light is particularly beneficial in setting up protocols that promote long-term preservation. For instance, dark-colored storage containers can prevent light exposure and mitigate potential risks associated with degradation.
Common Methods for Storing DNA Samples
When it comes to preserving the integrity of DNA samples, the methodologies used can vastly influence outcomes in both research and forensic applications. Understanding these common methods is vital, as efficient preservation techniques can drastically enhance sample longevity and usability. Here, we delve into three primary methods: cryopreservation, ethanol storage, and liquid nitrogen storage. Each method offers unique benefits and constraints, demanding thoughtful consideration based on the intended use of the samples.
Cryopreservation Techniques
Cryopreservation is a method widely favoured in biological research for its efficiency in halting biological activity at ultra-low temperatures. This technique is particularly critical when dealing with live cells or more complex specimens, such as those that may involve cell lines or primary cell cultures.
The fundamental principle behind cryopreservation involves cooling samples to temperatures below -130 degrees Celsius, often achieved using liquid nitrogen or specialized freezers. Here are a few key components:
- Controlled Cooling: Slow cooling rates are necessary to avoid ice crystal formation within the cells, which can cause rupture.
- Cryoprotectants: Substances like dimethyl sulfoxide (DMSO) or glycerol are often mixed with samples to protect the cells from damage during the freezing process.
- Thawing Protocols: Proper thawing is as crucial as freezing. Rapid thawing helps avoid osmotic shock from ice crystal melting, ensuring sample viability.
Utilizing cryopreservation can prolong the lifespan of DNA samples significantly, making it a cornerstone technique in many research environments.
Storage in Ethanol
Using ethanol for DNA storage represents a less complex but still effective approach. Ethanol has been historically utilized due to its ability to precipitate DNA, separating it from contaminants. This method typically involves the following steps:
- Precipitation: DNA is mixed with ethanol to precipitate the nucleic acids, allowing for easy collection by centrifugation.
- Washing: The DNA pellet is usually washed with 70% ethanol to remove any remaining salts and impurities.
- Drying: Proper drying of the DNA is essential to avoid degradation.
- Rehydration: When ready for use, the DNA can be rehydrated in an appropriate buffer.
While this method is straightforward and cost-effective, it comes with its own challenges. Samples stored in ethanol can sometimes be sensitive to temperature variations and light exposure, requiring careful handling to maintain sample integrity.
Liquid Nitrogen Storage
Storage in liquid nitrogen is often viewed as the gold standard for DNA preservation. The sub-arctic temperatures (around -196 degrees Celsius) effectively halt biological activity and prevent degradation processes. This method offers several advantages:
- Long-Term Viability: DNA samples stored in liquid nitrogen can remain viable for decades, with little risk of degradation.
- Minimal Contamination Risk: With proper protocols, contamination can be significantly reduced, thus maintaining the purity of samples.
- Versatility: This method can be applied to a wide variety of biological materials, including tissues, cells, and even complete organisms.
Nevertheless, challenges persist. The need for specialized storage tanks and monitoring systems adds a layer of complexity and cost. Furthermore, the potential for human error during handling can lead to catastrophic results if not properly managed.
In summation, while cryopreservation, ethanol storage, and liquid nitrogen methods each have distinct advantages, the choice depends on the specific needs of the research or application. Understanding their nuances proves essential for any scientist intent on optimal DNA sample management.
"The choice of storage method can mean the difference between a successful experiment and one that is doomed from the start."
Ultimately, careful attention to the methods employed will pave the way for more reliable results in the diverse fields of genetics and biotechnology.
Challenges in DNA Sample Storage
Storing DNA samples comes with its own set of challenges that can significantly affect the integrity and usability of the samples. Handling these challenges is crucial in ensuring that research outcomes are not compromised. Understanding the risks associated with storage methods is essential for scientists and professionals involved in genetic research, forensic studies, and medical applications.
Contamination Risks
Contamination poses a serious threat to the quality of stored DNA samples. When foreign biological or chemical materials mix with the DNA, it can lead to inaccurate results in analyses and experiments. The two principal forms of contamination are biological and chemical, each with its own implications.
Biological Contamination
Biological contamination typically arises from enzymes, microbes, or contaminants from the surrounding environment. One of the vital aspects of biological contamination is its unpredictability. Even trace amounts of foreign DNA can skew results during genetic analysis, leading to a domino effect of errors in downstream applications.
Biological contamination can be insidious, often unnoticed until it's too late. This is particularly concerning in forensic contexts where precision is crucial.
Characteristics that make biological contamination a significant issue include the ability of microbes to thrive in various conditions and their resilience against many sterilization techniques. As researchers handle samples—even in sterile environments—there’s always a risk of introducing DNA from their hands or instruments. The unique feature of biological contamination is that even when minimized, total eradication may not be feasible. The advantages of understanding these risks allow researchers to create protocols that mitigate sources of contamination and ensure outcomes that are as accurate as possible.
Chemical Contamination
Chemical contamination refers to the unfriendly interaction of DNA samples with various chemicals, including reagents used during storage or processing. The presence of contaminants from storage containers or reagents can lead to reactions that degrade or modify the DNA. This degradation can render the DNA unusable for precise analyses or experiments.
In terms of significance, chemical contamination raises alarms due to its potential to alter DNA integrity, making it challenging to achieve reliable results. One key characteristic of chemical contamination is that it’s often invisible. A researcher might think they're using perfectly clean equipment, yet residual chemicals from previous uses can silently wreak havoc.
The unique aspect of chemical contamination is its potential for widespread impact on research. Understanding this risk provides a rationale for using high-quality storage materials and implementing stringent cleaning protocols. The downsides of aspecting chemical contamination extend from the initial contamination to possible legal implications in forensic cases where evidence integrity is paramount.
Degradation Over Time
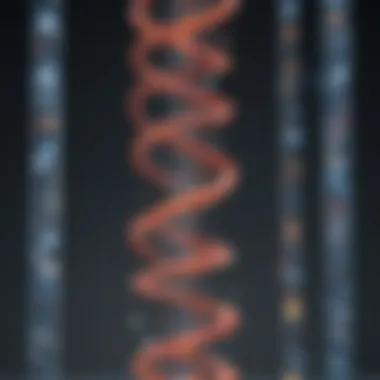
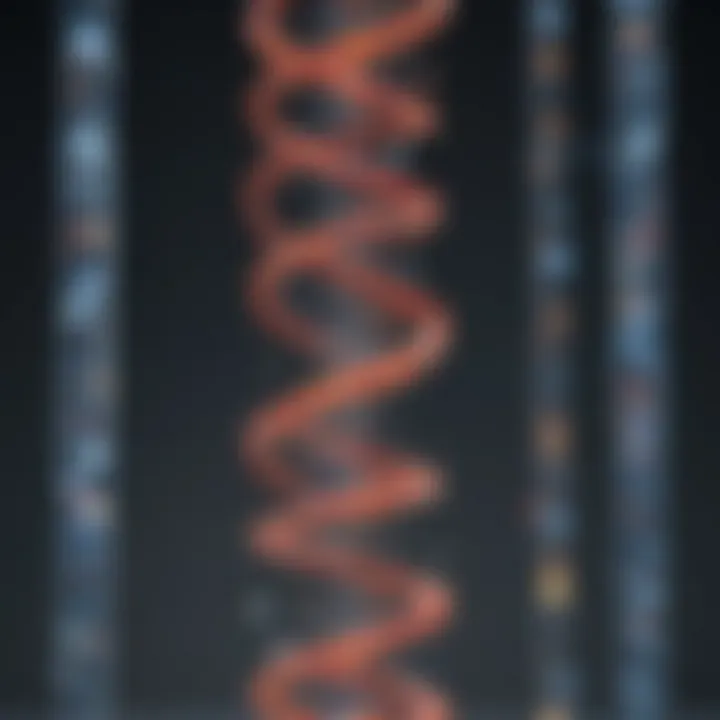
Another issue that merits attention is DNA degradation over time. Factors such as temperature oscillations, humidity, and exposure to light all play a role in how well DNA samples maintain their integrity. The process of degradation begins as soon as DNA is extracted from its biological source and can continue if the samples are not stored under optimal conditions.
Degradation primarily affects the stability of the DNA molecule. It can lead to fragmentation, which is detrimental during analyses such as sequencing and amplification. Time is an enemy of DNA preservation if samples are not handled correctly. Each passing day can contribute to a further decline in sample quality, and ultimately lead to potential failure in achieving research objectives. Therefore, it becomes critical to prioritize effective storage methods that minimize degradation risks over extended periods.
Material Considerations for Storage
When it comes to storing DNA samples, the selection of appropriate materials plays a crucial role in maintaining sample integrity and ensuring long-term preservation. Choosing the right materials can help minimize contamination risks, reduce degradation, and ensure that samples remain viable for future analysis. This section will delve into the importance of material considerations, focusing on specific elements that influence DNA storage outcomes.
Selecting Collection Vessels
Picking the right collection vessel is not just a matter of convenience; it's an essential factor in the success of sample storage. The vessel must offer protection against environmental factors that could potentially harm the DNA. For example, using high-quality microcentrifuge tubes or cryovials is common practice, as these tend to have low permeability and provide a good barrier against contamination.
Several types of collection vessels are available:
- Plastic Tubes: They are lightweight and resistant to breakage, making them a popular choice in laboratories.
- Glass Vials: Known for their excellent chemical stability, glass can be useful when working with certain sensitive samples.
Each option brings its own pros and cons, so understanding the intended use and the specific storage conditions can guide the selection process effectively.
Impact of Material Composition
The composition of the materials used in storage vessels significantly affects DNA preservation. Choosing between plastic and glass involves careful evaluation of their characteristics.
Plastic vs. Glass
When comparing plastic to glass, one can appreciate the lightweight, unbreakable nature of plastic tubes like polypropylene. These vessels provide convenience for handling and storing multiple samples, especially in situations where spills might occur. However, plastic can exhibit leaching issues if not properly selected, potentially introducing contaminants into the samples.
On the other hand, glass is often viewed as a gold standard due to its inert nature. However, glass containers can be fragile, posing a breakage risk during transport or storage. If durability is prioritized, plastic wll help avoid accidents, but meticulous material choice should prevail to ensure sample quality.
Sterilization Effects
Sterilization methods applied to collection vessels must cater to preserving the integrity of DNA samples. It's critical that sterilization ensures the removal of both biological and chemical contaminants. Common sterilization methods include autoclaving and gamma radiation. Each approach has unique characteristics that can impact DNA preservation.
- Autoclaving: While effective, it can cause certain plastic materials to warp or degrade, potentially compromising their structural integrity.
- Gamma Radiation: This method is favored for its ability to sterilize without exposing materials to high heat, making it suitable for sensitive applications. However, it may not be feasible for all types of materials.
As the field of DNA storage evolves, the importance of selecting the right materials cannot be overstated. The choices we make today will resonate in the quality of research tomorrow.
Regulatory Aspects of DNA Storage
Regulatory aspects of DNA storage play a pivotal role in ensuring the safe and ethical handling of genetic material. In a world where genetic data can drive significant advancements in medical research and forensics, understanding these regulations is essential. Governments and organizations establish guidelines that dictate how DNA samples should be collected, stored, and disposed of to protect both individual rights and public health. Compliance with these regulations not only fosters trust among stakeholders but also enhances the credibility of research conducted using stored DNA samples.
Compliance and Standards
Compliance is a cornerstone in the framework of DNA storage. Various entities, including institutions and laboratories, must adhere to specific standards to ensure that their practices meet strict safety and quality requirements. These include:
- National Regulations: Countries have laws governing the use of biological samples. For instance, the U.S. Centers for Disease Control and Prevention (CDC) and the Food and Drug Administration (FDA) provide guidelines for clinical laboratories.
- International Guidelines: Organizations like the World Health Organization (WHO) set forth recommendations that span countries. They ensure that best practices are universally acknowledged and adhered to.
- Accreditation Standards: Laboratories may seek accreditation from recognized bodies, such as the College of American Pathologists, to validate their methods and protocols.
It’s crucial not only to follow existing regulations but also to stay informed of new developments. Regulatory frameworks are continually evolving, reflecting advances in technology and changing societal norms surrounding privacy and genetic data usage. Staying compliant can be a hefty undertaking, but it’s paramount for maintaining the integrity of research and protecting participant rights.
Ethical Considerations
Ethics in DNA sample storage is multifaceted. On one hand, it encompasses the moral obligations of researchers and institutions towards the individuals from whom samples are sourced. On the other hand, it involves broader societal implications, especially when considering the potential misuse of genetic information. Key ethical considerations include:
- Informed Consent: Ensuring that all participants are adequately informed about how their DNA will be used and stored is essential. Clear communication about the purpose of storage, potential risks, and the duration of storage helps in fostering informed choices.
- Privacy and Confidentiality: Maintaining participant anonymity is a fundamental ethical obligation. Genetic information is sensitive, and any breach can lead to discrimination or stigmatization.
- Equitable Access: As technology evolves, it’s essential to ensure that advancements benefit all populations fairly. Ethical oversight means considering how stored DNA can be used in ways that uplift marginalized communities rather than exploit them.
“Ethics in genetic research is not just about adhering to laws. It’s about fostering trust with communities and maintaining the dignity of participants.”
In summary, the regulatory landscape surrounding DNA storage is crucial for ethical research practices. Navigating these waters requires vigilance and dedication but ultimately serves to fortify public confidence in genetic science. With careful attention to compliance and ethical considerations, the potential of stored DNA samples can be fully realized without compromising individual rights or societal values.
Innovations in DNA Sample Storage
In the evolving landscape of biological research, innovations in DNA sample storage are not just important; they are pivotal. With the ever-growing need for efficient preservation methods due to the increasing volume of genetic material being collected, the approaches toward storing this vital data have had to become more sophisticated. These innovations promise not just extended shelf life but also enhanced integrity and usability of stored samples, making them central to both current practices and future developments in various fields such as genomics, personalized medicine, and forensics.
This section dives into some groundbreaking advancements, focusing specifically on cryogenic technologies and smart storage solutions, which provide vital benefits and raise important considerations regarding the preservation of DNA samples.
Advancements in Cryogenic Technologies
One of the most exciting developments in DNA preservation is the enhancement of cryogenic storage technologies. Traditionally, vital samples were stored at -80°C or even lower, utilizing liquid nitrogen. While effective, those methods have evolved significantly. Newer cryogenic systems offer programmable temperature control and real-time monitoring of environmental conditions. This advancement is crucial because the slightest fluctuation in temperature can lead to degradation of DNA, rendering samples unusable.
A noteworthy example involves the use of automated storage solutions that not only maintain consistent temperatures but also incorporate advanced data logging tools to track storage conditions over time. Such technologies enable researchers to create databases of thousands of samples efficiently without compromising the quality.
Additionally, new cryoprotectants are being developed which help prevent ice crystal formation during the freezing process. This innovation reduces mechanical damage to DNA strands, ensuring a higher recovery rate when samples are thawed. As a result, the integrity of the genetic material is maintained, making it available for critical analyses in future research.
"In modern biobanks, the ability to maintain sample integrity through advanced cryogenic technologies is no longer a luxury; it’s a necessity for credible research outcomes."
Smart Storage Solutions
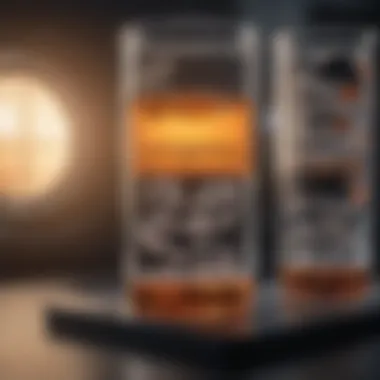
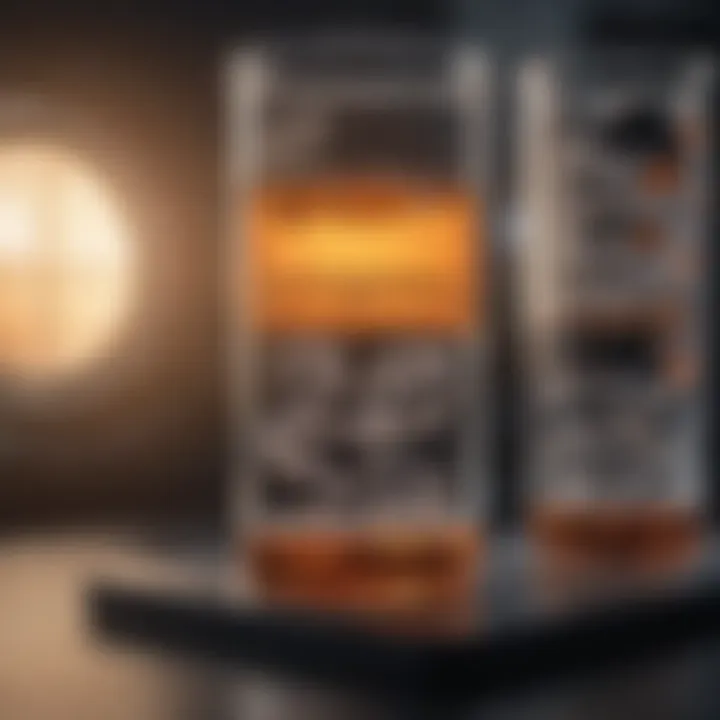
The rise of smart storage solutions signifies another leap forward in DNA sample storage. These systems integrate IoT technology to provide not only air-tight preservation but also intelligent monitoring. Smart units can track temperature, humidity, and even CO2 levels, alerting researchers to any anomalies via mobile notifications. Such features are essential for minimizing human error and ensuring lab conditions remain optimal for storing sensitive materials.
Moreover, user-friendly interfaces incorporated into these systems allow scientists to manage sample inventories efficiently. This capability includes real-time data access, reducing the chances of sample mislabeling or contamination—a significant risk in traditional storage settings.
These innovations make the process of sample tracking easier, from sample collection to retrieval for research purposes. As a result, they enhance the overall workflow in labs, translating to better resource management and improved research outcomes.
In summary, as the biotechnological field continues to advance, the smart integration of cryogenic technologies and smart storage solutions demonstrates a critical shift toward more reliable and efficient methods for storing DNA samples. This not only safeguards the precious genetic data but also aligns with the broader trend of embracing technology in scientific research.
Applications of Stored DNA Samples
The proper storage of DNA samples is not just a laboratory protocol; it serves as the cornerstone for countless applications across various fields. Indeed, the importance of these applications cannot be overstated. From criminal investigations to groundbreaking medical research, the utility of stored DNA lies in its ability to provide vital information. As we move further into an age where genetic analysis plays a crucial role in understanding health, behavior, and identity, the relevance of these applications is ever-increasing.
Stored DNA samples represent a repository of potent information, essential not only for forensic and medical domains but also for various emerging research fields.
This section delves into two primary avenues for stored DNA usage: its functions in forensic science and its vital applications in medical and research contexts.
Forensic Science Uses
Forensic science thrives on the ability to analyze and interpret DNA evidence collected from crime scenes. Stored DNA samples play a pivotal role here, serving as a reference point for comparisons when evidence surfaces. The process often begins with the collection of DNA from a suspect or a crime scene. Once collected, these samples need meticulous handling and storage to maintain their integrity, ensuring they can withstand examination in a courtroom setting.
Several key benefits stem from utilizing stored DNA in forensics:
- Identifying Suspects: The primary function of DNA in forensics is to match genetic profiles to individuals, aiding in both confirming and exonerating suspects. This DNA data bank acts as a modern fingerprint system, enhancing law enforcement’s ability to solve cold cases.
- Cold Case Solvers: DNA samples stored from previous untested evidence can lead to breakthroughs in older cases that have long been stagnant. New advancements in DNA analysis allow forensic specialists to revisit these samples, giving law enforcement a second chance at justice.
- Investigative Leads: In instances where suspects are unknown, forensic DNA can help create profiles of unidentified perpetrators, leading to potential matches in their databases or similar cases.
Navigating the legal implications of using stored DNA in forensic science also is critical. Proper documentation, labeling, and adherence to compliance regulations ensure the integrity of the samples and respect for privacy concerns.
Medical and Research Applications
Beyond the realm of law enforcement, the medical field capitalizes on the information embedded in DNA samples to optimize patient care and advance scientific knowledge. Here are a few significant aspects:
- Personalized Medicine: Stored DNA allows healthcare providers to tailor treatments unique to an individual’s genetic makeup. This form of precision medicine can provide insights into how a patient may respond to certain drugs or therapies, indicating which treatments may hold the most promise.
- Disease Research: Understanding genetic variants linked to diseases fosters breakthroughs in diagnosing and treating conditions. Researchers often utilize stored samples in biobanks to study correlations between specific genes and diseases, paving the way for novel treatment modalities.
- Pharmacogenomics: This subfield allows for the use of DNA to ascertain how individuals metabolize medications, which can significantly improve prescription practices, preventing adverse drug reactions and enhancing therapeutic efficacy.
- Epidemiological Studies: Large-scale research often relies on archived DNA samples to identify genetic predispositions to diseases in diverse populations, leading to richer data sets that inform public health policies.
Overall, the applications of stored DNA samples stand at the intersection of science, law, and ethical considerations. Being aware of how deeply they influence various sectors can enhance our understanding of their significance, highlighting the necessity to maintain rigorous standards in their preservation.
Future Directions in DNA Sample Storage
The journey of understanding and improving the storage of DNA samples is ongoing, and the future is bright. This section aims to illuminate the anticipated developments that can significantly impact the field of genetic research and biotechnology. As new technologies emerge, the way we store and manage DNA samples will evolve as well, pushing the boundaries of what is possible.
Trends in Biotechnology
As we look to the horizon, several trends in biotechnology hint at what we might see in DNA sample storage in the coming years. One notable trend is the increasing integration of automation and artificial intelligence in biobanking processes. This could streamline sample handling, reduce human error, and ultimately, enhance sample tracking and management.
- Automation: Automated systems for sample retrieval and storage can significantly reduce the time and labor costs involved in managing DNA samples. They can maintain consistent environmental conditions while ensuring quick access to samples for testing or research.
- Artificial Intelligence: The application of AI could revolutionize data analysis, allowing researchers to extract useful insights from large databases of DNA samples, thus playing a crucial role in identifying patterns and correlations.
- Advanced Cryopreservation: Innovations in cryoprotectants and protocols are also gaining momentum. These advancements could enable more efficient preservation of samples, reducing the chances of degradation and contamination.
Staying on the cutting edge of technology is paramount not only for quality control but also for compliance with evolving regulations surrounding DNA research.
Implications for Personalized Medicine
Personalized medicine is where DNA storage practices intersect directly with patient care. The ability to access well-preserved genetic samples informs the development of tailored treatments based on an individual’s specific genetic makeup. In the future, we can expect more robust systems for the storage, retrieval, and analysis of DNA samples, which will directly impact the effectiveness of personalized therapies.
- Targeted Therapies: Better storage techniques will facilitate larger and more diverse genomic databases, allowing researchers and clinicians to identify genetic markers for diseases more effectively.
- Real-time Access: With advances in biobanking technology, clinics may be able to access genetic information in real-time, enabling faster decisions regarding treatment plans.
- Ethical Considerations: As the use of personal genetic data becomes more prevalent, the need for ethical guidelines around data storage and access becomes increasingly critical. This will help build trust with patients, ensuring they understand how their data will be used and protected.
"As the future unfolds, the synergy between technological advances and personalized approaches could reshape healthcare fundamentally, allowing for not just reactive but proactive measures in disease treatment."
Overall, the direction in which DNA sample storage is heading holds considerable promise not only for biotechnological advancements but also for the broader field of medical practice. Enhancing our approach to storing DNA samples could very well translate into more effective treatments and better healthcare outcomes.
Closure
The conclusion serves as a vital synthesis of the key themes explored in the article regarding the storage of DNA samples. This section reiterates the significance of proper preservation methods, highlighting that the longevity and integrity of DNA samples are paramount in fields like forensic science and medical research. The findings underscore that, without reliable storage protocols, efforts to utilize genetic material in advancing health care or solving crimes can be severely compromised.
In summarizing the key points, it becomes evident that various factors influence effective DNA sample storage:
- Molecular stability remains crucial, ensuring that samples can be retrieved and analyzed without deterioration.
- Environmental conditions designed around temperature, humidity, and light exposure are non-negotiable.
- The choice of materials for collection and storage can significantly impact sample quality.
- Compliance with regulatory frameworks ensures ethical practices in DNA handling.
- Emerging technologies bring forth innovative solutions for long-term preservation, leading to a new era of possibilities in research and application.
This comprehensive understanding provides a framework for best practices, essential for students, researchers, educators, and professionals navigating the complex landscape of DNA sample preservation.
Summary of Key Findings
The article brought to light several critical findings about DNA storage:
- Optimal storage conditions are essential for maintaining sample integrity, involving carefully managed environments.
- Methods such as cryopreservation and liquid nitrogen storage stand out as effective techniques but require careful handling and research.
- Awareness of contamination risks and degradation factors is necessary for ensuring the quality of the samples over time.
- Regulatory and ethical implications must inform best practices, reflecting a broader understanding of the societal impact of DNA research.
The interplay of these elements provides a solid foundation for best practices in DNA storage, emphasizing the need for ongoing research and innovation in this field.
Final Thoughts on DNA Preservation
In reflecting on DNA preservation, it's crucial to recognize it as not merely a matter of storage but as a cornerstone of scientific advancement. The world is witnessing a rapid evolution in techniques that not only extend the viability of DNA samples but also enhance our ability to analyze them effectively. The future of DNA research will rely heavily on our commitment to responsible handling and pioneering methods of preservation.
In a world increasingly leaning on genetic information for specialized diagnoses and personalized treatments, the impact of proper DNA sample storage cannot be overstated. With every storage method polished to its peak efficiency, the opportunities push beyond mere research, reaching into real-world applications that benefit health, justice, and knowledge accumulation—driving forth a collective understanding of our biological heritage and potential.
"The preservation of DNA is not just about storing a biological sample; it’s about holding the key to future inquiry and discovery."
As the landscape of DNA storage evolves, let us prioritize innovation while fostering ethical practices that honor the digitally connected world we inhabit.