Exploring Direct Air Capture: Mechanisms and Applications
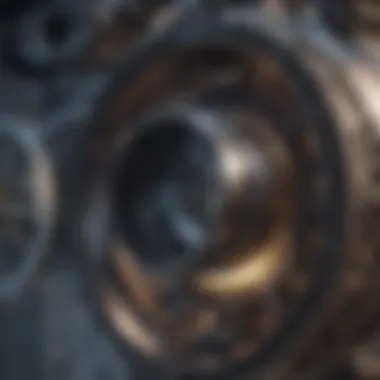
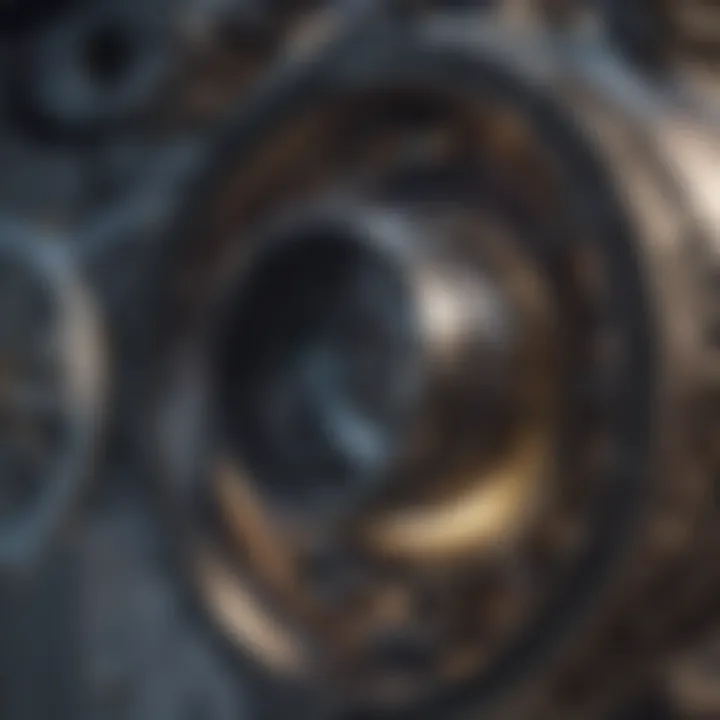
Intro
As the effects of climate change become increasingly palpable, innovative solutions are imperative for tackling the growing concentrations of greenhouse gases in our atmosphere. One such promising technology garnering attention is Direct Air Capture (DAC). This process involves filtering carbon dioxide directly from the air, presenting a method to mitigate the adverse impacts of climate change. While conversations about renewable energy sources often steal the spotlight, the role of DAC cannot be understated, as it offers unique opportunities to reduce atmospheric CO2 levels.
DAC technology operates on intricate mechanisms that allow for the effective extraction of carbon dioxide (CO2). However, it is not merely about pulling the gas out of the air. The implications of this technology stretch far beyond just reduction figures; they also encompass economic feasibility, environmental impact, and potential scalability in climate action strategies.
In light of its importance, it becomes essential to unpack the various facets of DAC. This article aims to offer a thorough exploration of the mechanisms behind DAC, the applications it serves, and the broader implications it carries for our planet. By examining the operational methodologies and assessing the economic and environmental ramifications, a clearer picture emerges as to how DAC technology can play a pivotal role in future climate initiatives and sustainable development.
Prolusion to Direct Air Capture
Direct Air Capture (DAC) stands at the forefront of climate technology, aiming to address one of the most pressing challenges of our timeβclimate change. The importance of this topic canβt be overstated; as emissions from industrial activities continue to rise, finding effective solutions for carbon removal is paramount. DAC offers a tangible method for pulling carbon dioxide directly from the air, thereby mitigating the adverse effects of greenhouse gas emissions.
Not only does DAC have the potential to directly curb atmospheric CO2 levels, it can also play a crucial role in achieving international climate targets. The technologyβs versatility allows for integration into various sectors, including energy, transportation, and manufacturing. Given the growing urgency for scalable, effective carbon removal solutions, understanding DACβs mechanisms, applications, and implications becomes vital.
Defining Direct Air Capture
Direct Air Capture refers to a set of processes that capture carbon dioxide from ambient air. Unlike traditional carbon capture techniques, which typically focus on emissions from specific sources like power plants, DAC directly interacts with the atmosphere. The captured CO2 can be stored underground or utilized in various industrial processes, making it a flexible tool in the fight against climate change.
There are primarily two methods of DAC:
- Chemical absorption, which removes CO2 using chemical reactions with special solvents.
- Physical adsorption, where CO2 molecules adhere to solid materials without reacting chemically.
These methods showcase the innovative approaches DAC employs, illustrating how technology can harness chemical principles to achieve environmental goals.
Historical Context of Carbon Removal Technologies
The history of carbon removal technologies provides a backdrop for understanding the evolution of DAC. In the early 20th century, scientists began to explore the concept of removing pollutants from the air, but serious advancements came later. The development of carbon capture technology was largely influenced by the need to combat industrial emissions.
In the 1970s, the oil crisis prompted more research into alternative energy sources and carbon management, laying the groundwork for future DAC innovations. By the 2000s, as concerns about climate change reached a boiling point, DAC began to gain traction as a viable countermeasure.
βThe history of DAC illustrates a growing recognition of the need to take action against carbon emissions, reflecting changing societal attitudes towards environmental responsibility.β
Today, countries around the globe are investing in DAC research and pilot projects, showing a collective will to utilize cutting-edge technology to shape a sustainable future. This historical context is essential to appreciate DAC's current landscape and its implications for tomorrow.
Mechanisms of DAC Technology
Understanding the mechanisms of Direct Air Capture (DAC) technology is crucial for grasping how it can contribute to mitigating climate change. This section breaks down the essential processes involved in the capturing of carbon dioxide from the atmosphere. Itβs not just a matter of removing CO2; itβs about selecting the right method to ensure efficiency, scalability, and economic viability. By analyzing these mechanisms, we aim to highlight their respective benefits and challenges, which are vital for anyone invested in sustainable technologies.
Chemical Absorption Methods
Chemical absorption methods rely on the interaction between a gas and a liquid solvent to capture CO2 from the air. Generally, amine solutions are the most commonly used solvents due to their high reactivity with carbon dioxide, forming a weak bond. This process typically involves two stages: absorption and regeneration. In the absorption phase, ambient air is brought into contact with the solvent in a scrubber, where CO2 is captured. Later, in the regeneration stage, the solvent is heated or depressurized to release the trapped CO2, making the solvent reusable.
The advantages of chemical absorption methods include:
- High selectivity towards CO2, enabling effective capture even at low concentrations.
- The ability to operate in diverse environmental conditions.
However, there are challenges to consider. The regeneration process is energy-intensive, often requiring significant heat, which can reduce the overall efficiency. Additionally, the degradation of solvents over time can lead to increased costs and maintenance issues.
Physical Adsorption Techniques
Physical adsorption, unlike chemical absorption, relies on physical forces, such as van der Waals forces, to capture CO2 on the surface of solid materials known as adsorbents. In this mechanism, materials such as zeolites, activated carbon, or metal-organic frameworks (MOFs) serve as the adsorbents, offering a high surface area for gas interaction. When air passes through a bed of these materials, CO2 molecules stick to the adsorbent. This method can be reversed by simply applying heat or a vacuum to release the captured CO2.
Some notable benefits of physical adsorption techniques include:
- Lower energy requirements for desorption compared to chemical absorption, leading to reduced operational costs.
- The potential for using abundant materials in adsorbents, making the technology more accessible.
However, adsorption is less effective at lower CO2 concentrations found in the ambient atmosphere. This limitation means that additional innovations are often needed to optimize the process further.
Hybrid Systems for Enhanced Efficiency
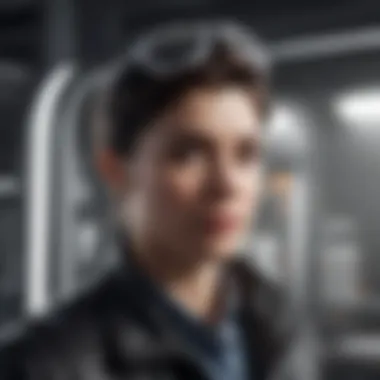
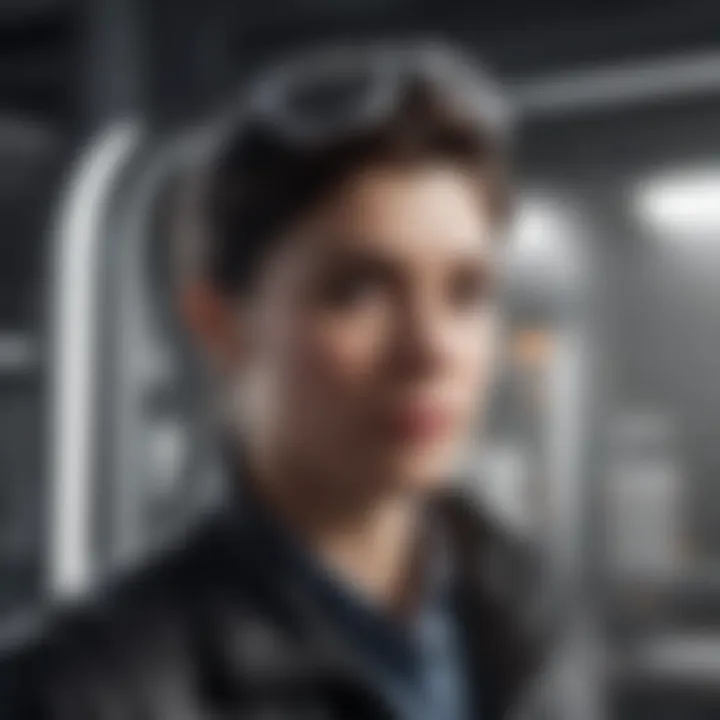
Hybrid systems combine aspects of both chemical and physical processes to improve the overall carbon capture efficiency. These systems can utilize the high capacity of chemical absorption to capture larger amounts of carbon dioxide, followed by physical adsorption to enhance the regeneration efficiency. By doing this, hybrid systems can mitigate the energy costs often associated with traditional chemical absorption and capitalize on the unique benefits of each method.
For example, one hybrid approach utilizes a chemical solvent to capture CO2 and then switches to a physical adsorbent to facilitate the release of CO2 with lower energy input.
Key advantages of hybrid systems include:
- Flexible adaptability based on operational conditions and desired outputs.
- Reduction in total energy consumption while increasing the quantity of CO2 removed.
Types of DAC Technologies
Understanding the different types of Direct Air Capture (DAC) technologies is crucial for grasping how they contribute to climate change mitigation efforts. Various systems exist, each tailored to specific operational contexts and outcomes. Their relative merits, efficiencies, and potential challenges demand careful consideration as we navigate the path toward a more sustainable future.
Engineered Systems and Their Functionality
Engineered systems represent the more technologically advanced category of DAC methods. They typically rely on mechanical processes to effectively capture carbon dioxide from the air, utilizing robust hardware configured for this precise task. One of the leading models is the Climeworks system, which employs a chemical process to absorb COβ, subsequently releasing it through a heating mechanism. This closed-loop operation is vital, as it ensures the captured carbon can be stored practically and sustainably.
These systems' functionality often hinges on their design, including the materials used in filters that can adsorb COβ molecules selectively. High-capacity sorbents, such as amines, facilitate the efficient collection of COβ. From a performance perspective, engineered systems generally exhibit high capture rates, making them suitable for large-scale deployment.
However, the intricacies of implementing such technologies are not without their own set of challenges.
- High initial costs: Setting up engineered systems requires considerable capital investment.
- Energy requirements: The energy costs associated with capturing and processing COβ can be substantial, necessitating a reliable source of renewable energy.
- Land considerations: The necessary space for these systems must be factored into any planning, requiring analysis of potential sites to optimize efficiency.
In recent years, several governments and private investors have begun channeling resources into refining these technologies. Proponents argue that continued advancements could reduce costs and increase efficiencies, allowing DAC to become a viable component of broader carbon neutrality strategies.
Natural and Bio-Based Approaches
In contrast, natural and bio-based approaches have their roots much deeper in ecological processes. These systems utilize natural mechanisms, such as photosynthesis, to absorb COβ. The most common examples include afforestation and soil carbon sequestration.
- Afforestation involves planting trees which act as natural carbon sinks β the more trees, the more COβ absorbed.
- Soil carbon sequestration improves soil health, enhancing its capacity to store carbon effectively. Practices like regenerative agriculture play a pivotal role in these initiatives.
These approaches boast several benefits:
- Lower implementation costs: Typically, natural strategies require lesser capital compared to engineered systems.
- Biodiversity enhancement: Implementing these methods supports ecosystem restoration and can lead to increased biodiversity.
- Community involvement: Initiatives can engage local communities in carbon reduction efforts, fostering a sense of stewardship toward environmental sustainability.
However, it's important to recognize that while these methodologies offer valuable solutions, they are not without limitations. For instance,
- Scalability issues: It might be challenging to implement these solutions on a scale that significantly impacts atmospheric COβ levels.
- Time for effectiveness: It often takes years before afforested areas reach their peak carbon absorption capacity.
The synergy between engineered systems and natural approaches proposes an intriguing avenue for research. The potential for hybrid systemsβwhere both strategies are integratedβmight yield the most effective solutions for carbon capture, leveraging the strengths of both methodologies.
As DAC technologies continue to evolve, the dialogue surrounding their diverse applications highlights the necessity of exploring every avenue available for effective climate action.
Applications of Direct Air Capture
Understanding the applications of Direct Air Capture (DAC) technology is essential, not just for grasping its operational potential but also for recognizing its strategic importance in combating climate change. DAC offers a multifaceted approach to mitigating atmospheric carbon dioxide levels, and its relevance extends across various sectors, from renewable energy to industrial processes. The integration of DAC into ongoing and upcoming projects marks a significant advancement in both environmental sustainability and carbon neutrality efforts.
Integration with Renewable Energy Sources
The relationship between DAC and renewable energy is particularly noteworthy. As the world shifts towards wind, solar, and hydropower, the question arises: how can DAC complement these energy sources? By harnessing excess energy generated by renewables, DAC systems can operate more efficiently and cost-effectively. This synergy is crucial since DAC requires significant amounts of energy to function.
Consider a solar farm that generates surplus electricity during peak hours. If this energy could feed into a nearby DAC facility, the captured CO2 could be utilized in various ways, such as in greenhouse agriculture or transformed into fuels through chemical processes. Itβs a win-win situation, allowing for a more continuous energy flow and creating a sustainable cycle of energy use and carbon reduction.
"Integrating DAC with renewable energy could be the game-changer we need in lowering global carbon emissions."
DAC in Carbon Neutrality Plans
Another critical aspect of DAC's application is its role in achieving carbon neutrality. Many countries have set ambitious targets to become carbon neutral by 2050 or sooner. To meet these goals, reducing carbon emissions through conventional means is often not enough. This is where DAC comes into play, acting as a pivotal technology to offset emissions that are difficult to eliminate entirely.
For instance, in scenarios where industries still depend on fossil fuels, DAC could effectively capture emitted CO2, pushing the organization closer to their neutrality targets. Several governments and enterprises are already crafting frameworks that incorporate DAC into their long-term strategies. These plans often involve policy adjustments and investment in DAC technology as an essential avenue for sustainable development.
Implementation in Industrial Processes
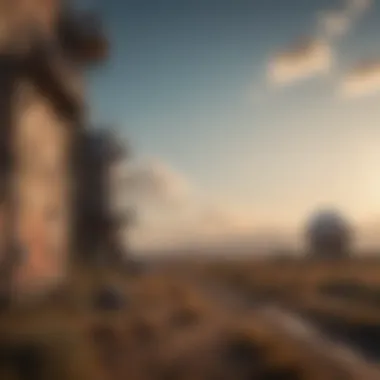
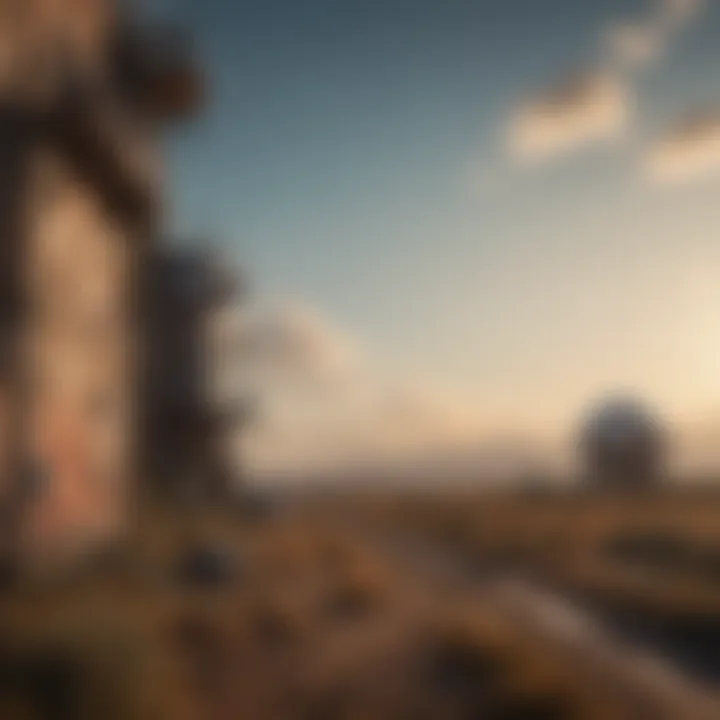
The applications of DAC technology also expand into traditional industrial processes. Industries like cement, steel, and chemical manufacturing are among the largest sources of greenhouse gas emissions. By integrating DAC systems within these sectors, businesses can take proactive steps toward the decarbonization of their operations.
For example, imagine a cement plant that incorporates a DAC unit into its emissions management system. This facility could capture a significant portion of its process emissions, drastically reducing its overall carbon footprint. Furthermore, the captured CO2 could be recycled for use in product manufacturing or geologic storage, leading to innovative solutions that also generate economic benefits.
Environmental Impact of DAC Technology
The significance of understanding the environmental impact of Direct Air Capture (DAC) technology cannot be overstated. As we grapple with the escalating levels of atmospheric carbon dioxide, the quest for effective solutions draws attention to the role of DAC. This technology holds the promise to not merely mitigate CO2 emissions but to actively remove it from the air we breathe. Thus, examining its environmental implications helps to shed light on a key avenue to combat climate change.
Lifecycle Analysis of DAC Systems
Lifecycle analysis (LCA) of DAC systems is pivotal in evaluating their sustainability. This approach involves a thorough examination from raw material extraction to the energy consumed during operation and finally, the end-of-life implications. Often, people tend to overlook how energy-intensive these systems can be. For instance, the production of components used in DAC can generate greenhouse gas emissions. So, an astute analysis of the entire lifecycle is critical, not just the final product.
Furthermore, consider the inputs. Capturing CO2 often requires significant energy, which can vary greatly in environmental impact based on the energy sources used. If powered by fossil fuels, the net benefit may dwindle, whereas coupling DAC with renewable energy sources substantially boosts its environmental standing.
A few aspects generally included in an LCA of DAC systems are:
- Raw Material Sourcing: How materials for equipment are sourced can have significant environmental implications. Sustainable sourcing options exist but are not universally applied.
- Operational Energy Use: The energy required to operate DAC systems is an important consideration. An evaluation of energy sources can help clarify the overall carbon footprint.
- Emissions During Transport: Movement of materials and captured CO2 can introduce additional emissions. Efficient logistics play a role in minimizing this impact.
- End-of-Life Management: The fate of DAC systems at the end of their utility must be planned to ensure minimal environmental harm.
Through lifecycle analysis, researchers and stakeholders can identify pain points, streamline processes, and improve DAC systems. Thus, thereβs a path toward reducing the overall negative environmental impacts that could offset gains from CO2 capture.
Potential Reductions in Atmospheric CO2 Levels
Another chief consideration surrounding DAC is its capacity to reduce atmospheric CO2 levels. This not only has implications for climate change mitigation but also for ecological balance. Every ton of CO2 removed directly contributes to lower global temperatures, a promising step toward staving off climate calamities.
Research indicates that if DAC systems are scaled properly, they could remove billions of tons of CO2 from the atmosphere annually. To illustrate:
- The Global Carbon Project highlights that current global emissions are approximately 36 billion tons per year. Billions of tons removed by DAC could help shift that balance.
- Several studies advocate for the potential of DAC technology being integrated into broader climate strategies. This includes restoration of degraded ecosystems, which combined efforts could synergistically combat climate issues.
βDirect Air Capture offers a tangible route to not just slow but potentially reverse some of the damage caused to our atmosphere.β
The capacity of DAC to directly impact atmospheric conditions is contingent on several factors:
- Technological Advancement: The more efficient DAC systems become, the greater their potential to sequester CO2.
- Investment and Policy Support: Sustained funding and sound policies are critical to drive innovations and expand kapacity.
- Social Acceptance: Gaining public trust and understanding can pave way for smoother implementation.
In sum, the potential for DAC to significantly curtail atmospheric CO2 levels represents a beacon of hope in climate discussions. Maintaining a sharp focus on environmental impacts, alongside technological and operational efficiency, is paramount for the promise of DAC technology to be realized.
Economic Considerations of DAC
The economic landscape surrounding Direct Air Capture (DAC) is pivotal as the success of this technology will heavily rely on its financial viability. Understanding the costs, investment trends, and overall market dynamics is crucial for stakeholders aiming to implement DAC solutions in either public or private spheres. A sound grasp of these elements helps in shaping policies, securing funding, and ultimately determining the role of DAC in future climate agendas.
Cost Analysis of Deployment
The costs associated with deploying DAC technologies can vary widely based on several factors. Primarily, the specifics of the technology chosen, location, and scale of the deployment play decisive roles. Different approaches such as chemical absorption and physical adsorption can incur vastly different costs.
- Capital Expenditures: Initial investment can be hefty. From constructing facilities to purchasing necessary equipment, start-up costs can run into the millions. For instance, estimates suggest that producing carbon removal through DAC can be as expensive as $600 per ton of CO2.
- Operational Expenses: Once the system is in place, ongoing operational and maintenance costs also come into play. Utilities like electricity and water required for operation can affect overall expenses. It's essential to consider that as technology advances, these costs may decrease, leading to potential sustainability in various implementations.
- Economies of Scale: Expanding the deployment scale can lead to cost reductions. Larger facilities or industrial applications may benefit from reduced per-unit costs, making DAC more economically attractive in the long run. Navigating this delicate balance between scale and cost-efficiency will be instrumental in refining DAC technology viability.
Investment Trends in DAC Technologies
Investment trends within the DAC sector indicate a growing confidence in its potential. More venture capital firms and institutional investors are beginning to recognize the viability of carbon capture as a necessary solution to climate challenges.
- Increasing Funding: Several high-profile investments signal a robust belief in DAC's future. Companies like Carbon Engineering and Climeworks have attracted significant financial backing, allowing them to innovate and scale their operations.
- Government Interest: Several governments have allocated funding to research and develop DAC technologies as part of broader climate action plans. Policies favoring tax incentives for carbon capture initiatives can catalyze a surge in investments.
- Emerging Partnerships: Collaborations between private companies, research institutions, and government bodies have begun to flourish. These partnerships are essential for accelerating technology development and reducing costs, creating a fertile ground for future investment.
Market Dynamics and Potential for Growth
As DAC technologies mature, several factors are influencing market dynamics:
- Public Awareness: Heightened awareness of climate change has driven consumer demand for more sustainable solutions. As public sentiment increasingly favors carbon offset initiatives, companies utilizing DAC technology may find themselves better positioned in the market.
- Carbon Pricing Mechanisms: The introduction of carbon pricing in various regions enhances the economic advantages of DAC, making carbon capture more lucrative. As countries enforce stricter emissions regulations, the market for DAC could expand significantly.
- Global Climate Policy: International climate accords often encourage investment in new technologies aimed at reducing greenhouse gas emissions. The role of DAC in meeting targets under these accords could lead to enhanced market prospects and greater support.
"The pathway to mass adoption of DAC hinges significantly on its economic viability, coupled with collaborative efforts to enhance technology's accessibility and affordability."
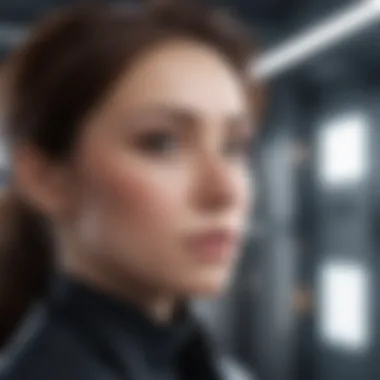
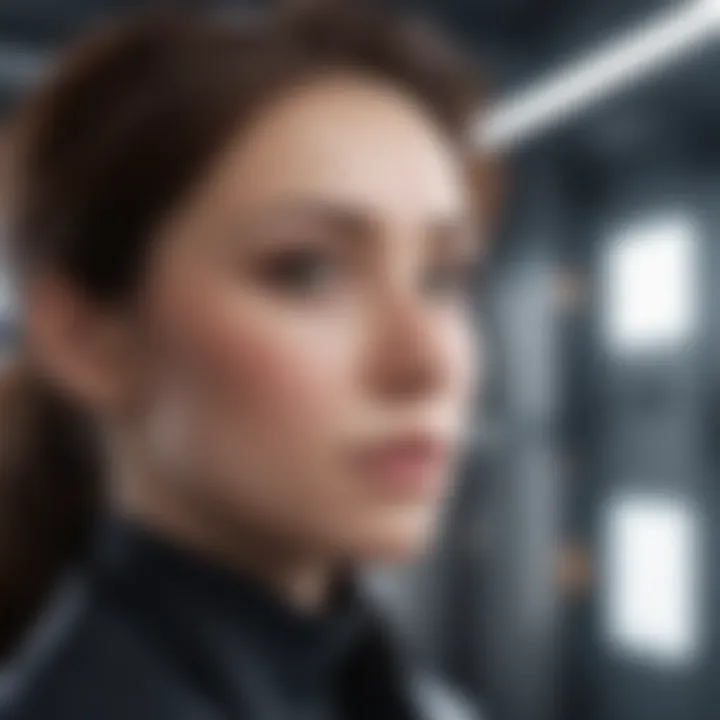
For further exploration on the economic implications of DAC technologies, visit Wikipedia or check out more insights on Britannica.
Challenges Facing DAC Technology
Understanding the challenges facing Direct Air Capture (DAC) technology is essential for grasping its role in combating climate change. While DAC holds great promise, its widespread adoption is impeded by a complex array of technical and regulatory barriers. Addressing these obstacles is vital to fully realize the potential benefits of DAC in a world grappling with ever-increasing levels of carbon dioxide.
Technical Barriers to Widespread Adoption
Deploying DAC technology on a large scale encounters several technical challenges that can hinder its success. The efficiency of capturing atmospheric CO2 is heavily influenced by the materials and methods employed in the extraction process. Many DAC systems utilize sorbents or solvents that can experience degradation over time, which can lead to reduced efficiency and higher operational costs.
Another significant hurdle is the energy requirement for carbon capture and subsequent processing. Most DAC systems need substantial energy inputs, often from fossil fuels, which could undermine their environmental benefits. Finding a balance between energy requirements and eco-friendly practices remains a daunting task.
Moreover, scalability poses a major concern. Small-scale DAC installations can show promising results in controlled environments, but replicating that success at an industrial scale while maintaining efficiency and cost-effectiveness is no small feat. Resolving these technical challenges is crucial for transforming DAC from a theoretical solution into a practical, widely utilized approach.
Regulatory and Policy Hurdles
The journey of DAC technology doesn't end at the technical challenges; it wades into the murky waters of regulation and policy, where clarity is often lacking. Regulatory frameworks for emerging technologies are frequently antiquated or non-existent, leading to confusion among potential investors and developers. For DAC to flourish, comprehensive guidelines and standards need to be established that both ensure safety and promote innovation.
Furthermore, government incentives for carbon capture and removal technologies can be inconsistent. In some regions, subsidies may be available, while others remain opaque or entirely absent. This inconsistency can discourage investments needed to drive the technology forward. In fact, navigating these policies can often feel like walking through a minefield; one wrong step might derail a project entirely.
"The single biggest barrier to scaling up DAC is not just technical; itβs the tangled web of regulatory frameworks that create uncertainty in the investment and deployment landscape."
Ultimately, the interplay between technology and policy is critical. For DAC to play a meaningful role in our climate strategy, both sectors must come together to address these hurdles, ensuring that innovations can become part of a holistic approach to reducing our carbon footprint.
While challenges abound, they also offer opportunities for advancement and collaboration. A concerted effort among scientists, policymakers, and industry stakeholders can help pave the way for a future where DAC technology not only survives but thrives in the ever-pressing battle against climate change.
Future Perspectives in Direct Air Capture
As we look ahead, the importance of Direct Air Capture (DAC) technology becomes increasingly evident in the fight against climate change. The urgency to reduce carbon emissions isnβt just a whisper among environmentalists; it's become a clarion call for action. High concentrations of carbon dioxide in our atmosphere are leading to worrisome climatic shifts, and DAC represents a viable path toward mitigating these impacts. Probing into the future of DAC enlightens discussions on innovations, strategic roles in global policies, and the collaborative efforts needed to ramp up this technology.
Innovations on the Horizon
The landscape of DAC is rapidly evolving, with innovations emerging that hold promise for more efficient and cost-effective methods of carbon extraction. One of the significant advancements includes the integration of nanotechnology into DAC systems, which could lead to enhancements in the materials used for CO2 capture. New absorbent materials that are more efficient at lower costs and can operate under varying weather conditions are on the drawing board.
In addition to materials science, machine learning algorithms are being implemented to optimize the operation of DAC systems. Predictive maintenance, operational efficiency, and real-time monitoring could revolutionize how these systems function. Not to mention, advancements in renewable energy technologies can significantly lower the operational costs associated with running DAC systems. This interplay hints at a future where capturing carbon is not just feasible but economically viable.
"Innovation is the key to unlocking the full potential of Direct Air Capture technologies."
DAC's Role in Global Climate Strategies
DAC is increasingly counted among the essential tools in global climate strategies. As nations set ambitious goals for carbon neutrality, DAC presents a unique solution that addresses residual emissions, especially from difficult-to-decarbonize sectors like aviation and heavy industry. Its integration into national climate policies could facilitate achieving targets set forth in the Paris Agreement.
Moreover, DAC can serve a dual purpose as it has the potential not only to remove carbon but also to generate economic opportunities. For instance, carbon dioxide captured by DAC can be reused in various industries such as beverage production, plastics, and fuels, creating a circular economy. The significance of this role cannot be overstated; it will foster collective efforts among countries towards a sustainable future.
Collaboration Between Industries and Governments
The future of DAC technology hinges greatly on the collaboration between the private sector and governmental bodies. This partnership is underpinned by the need for substantial capital investments in research and development. Public-private partnerships can catalyze innovations and create frameworks conducive to the scale-up of DAC technologies.
Funding initiatives, tax incentives, and regulatory mechanisms spearheaded by governments could provide the necessary impetus for industries to adopt DAC systems. Furthermore, platforms for sharing best practices and lessons learned are critical to accelerating the maturity of DAC technologies. The coalition between various stakeholders can create synergy in tackling the overarching challenge of climate change, allowing DAC to realize its full potential.
Closure
As we pull the curtain down on the discussion around Direct Air Capture (DAC), it is clear that this technology serves as a crucial player in the complex theatre of climate change mitigation. The surrounding discourse illustrates not only the intricacies of how DAC operates but also positions it at the intersection of science and policy, shedding light on its multifaceted nature.
Summarizing the Importance of DAC
DAC emerged as a beacon of hope at a time when the perils of climate change are unmistakably looming large over the global landscape. Its significance lies in several key areas:
- Carbon Reduction: The primary aim of DAC is to directly target atmospheric CO2. This offers a vital pathway to attain net-zero emissions, particularly in sectors where decarbonization proves challenging.
- Complementary to Other Technologies: DAC doesnβt function in isolation. It works hand-in-hand with renewable energy sources and other carbon capture technologies, creating a synergistic effect that amplifies their collective impact.
- Adaptability: The various DAC methods, from chemical absorption to bio-based approaches, provide unique tools that can be tailored to specific environmental and economic scenarios.
- Policy Frameworks: As countries strategize around their commitments to climate agreements, DAC could foster a fresh dialogue on regulatory frameworks, advancing policies that support cleaner air and sustainable practices.
In synthesizing these elements, the importance of DAC becomes evident; it's not just a technical solution but a pivotal strategy that can facilitate a broader shift towards sustainability and climate resilience.
Call to Action for Further Research and Implementation
As the urgency to address climate challenges intensifies, thereβs no room for complacency. The call for additional research and implementation of DAC technology is not merely an academic suggestion; it is a necessary step towards securing a livable future. Here are some considerations that highlight this urgency:
- Investment in Research: The scientific community should allocate more resources towards understanding the long-term impacts of DAC. Research on materials that can lower the operational costs of DAC systems could yield breakthroughs in efficiency.
- Pilot Projects: Initiatives at a smaller scale can provide invaluable data. Supporting pilot projects across diverse geographic locales will deepen our understanding of how DAC technologies interact with local ecosystems and economies.
- Public Awareness: Raising awareness about DAC and its benefits should not be overlooked. A well-informed public can form a foundation for wider acceptance and pressure on policymakers to prioritize such technologies.
- Interdisciplinary Dialogue: Collaboration between technologists, environmentalists, and policymakers is crucial. Engaging varied stakeholders not only enriches the investigative process but also aligns DAC initiatives with community values and needs.