Exploring Cytodex Microcarriers in Cell Culture
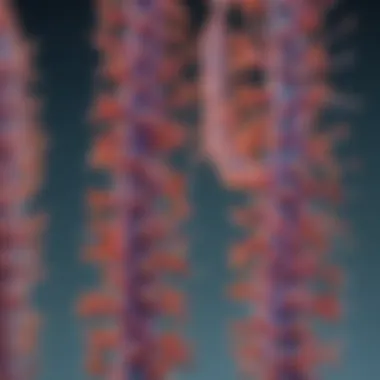
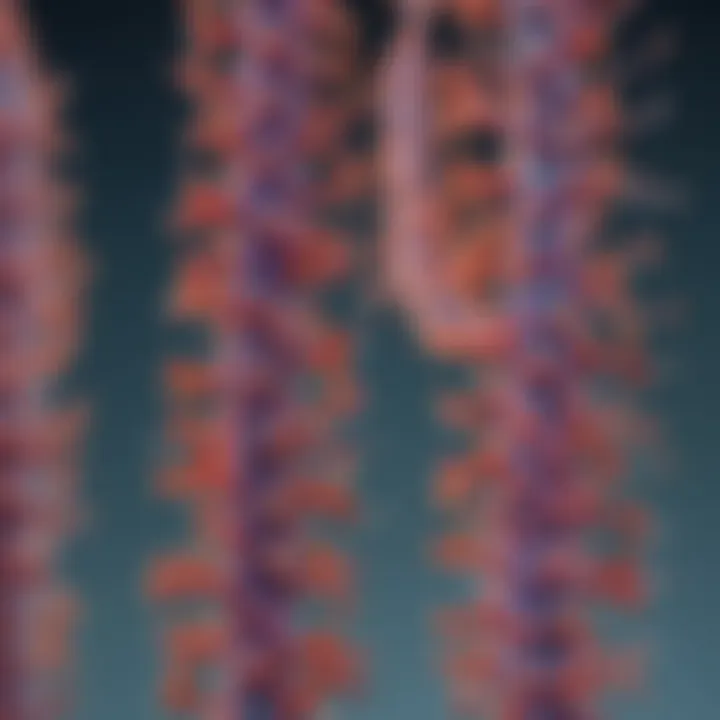
Intro
In the ever-evolving world of cell culture, Cytodex microcarriers have become a cornerstone, not just in academic research but also in the commercial sector. The dynamics of cell growth hinge significantly on the tools researchers use, and Cytodex microcarriers offer a versatile solution. These microcarriers, often described as tiny beads, provide a supportive environment that mimics the natural extracellular matrix, which is crucial for cell adhesion and proliferation.
But what exactly sets Cytodex microcarriers apart? For starters, it's their unique physical and chemical properties, which allow various cell types to thrive. Additionally, as we venture deeper into this article, we will unpack the diverse methodologies associated with the use of these microcarriers and how they can drastically improve cell cultures' yield and viability.
Before diving into the nitty-gritty details, itโs important to highlight the significance of this topic. As industries such as pharmaceuticals and biotechnology continue to expand, the demand for reliable and scalable cell culture techniques increases. Understanding the role of Cytodex microcarriers is pivotal for both current research and future innovations in therapeutic applications.
Research Overview
Summary of Key Findings
Recent investigations into Cytodex microcarriers have unveiled several key findings worth noting:
- Surface Modifications: Enhancements, such as specific coatings, have shown to facilitate better cell attachment and growth.
- Compatibility: Various cell lines have exhibited high viability when cultured on these microcarriers, reinforcing their adaptability across different applications.
- Efficiency Improvements: Studies have indicated that using Cytodex microcarriers can lead to significant increases in cell density and yield compared to traditional methods.
Methodologies Employed
To reach these conclusions, a variety of experimental approaches have been utilized, including:
- Comparative Studies: Evaluating the efficacy of Cytodex microcarriers against other carrier systems under various conditions.
- Cell Viability Assays: Utilizing tools like MTT assays to determine the metabolic activity of cells grown on microcarriers.
- Surface Characterization: Employing techniques such as scanning electron microscopy to analyze the microcarrier surfaces and their interactions with cells.
This structured approach to research provides a solid basis for understanding how and why Cytodex microcarriers are making waves in the cell culture landscape.
In-Depth Analysis
Detailed Examination of Results
By examining the outcomes of various studies, it becomes clear that Cytodex microcarriers cater to intricate bioprocessing requirements. For instance, when exploring various surface modifications, findings consistently demonstrate that specific hydrophilic and hydrophobic coatings lead to enhanced cell attachment. This is particularly significant for suspension cultures, where achieving optimal cell density can be challenging.
Comparison with Previous Studies
Moreover, these results can be directly compared to earlier research that utilized traditional adherent culture methods. In those cases, researchers often faced limitations around scalability, as cells would only grow in two dimensions. Cytodex microcarriers enable three-dimensional growth patterns, which are essential for mimicking natural tissue architectures. The shift from conventional methods to microcarrier-based systems not only enhances cell growth rate but also creates a pathway toward more advanced therapeutic applications.
In summary, as we delve deeper into the world of Cytodex microcarriers, it is essential to keep an eye on the innovative applications and methodologies evolving within this field. Understanding these dynamics will play a crucial role in shaping the future of cell culture technologies and their ability to meet the growing demands of research and industry.
Intro to Cytodex Microcarriers
In the realm of cell culture, Cytodex microcarriers hold significant importance. These microcarriers act as platforms for growing cells in laboratory settings, providing essential support and enhancing cell proliferation. Their unique attributes allow researchers to cultivate a diverse range of cell types, from adherent cells to those used in biopharmaceutical productions, thereby addressing various scientific and industrial needs.
The use of microcarriers can notably improve the efficiency of cell culture processes. They offer several benefits including a larger surface area for cell attachment, which is particularly beneficial in scaling up production. This is crucial for industries targeting therapeutic development or vaccine manufacturing, where the quantity and quality of cells matter immensely.
Moreover, Cytodex microcarriers facilitate a more simplified approach to handling cell cultures. As they can be suspended in a liquid medium, it allows for easier mixing and aeration. This ensures that all cells receive uniform exposure to nutrients and conditions, minimizing the risk of batch variations. Furthermore, these microcarriers can enhance cell yields and viability, an aspect that is paramount when sustainability and cost efficiency are on the table.
Considering the growing interest in regenerative medicine and biotechnology, understanding Cytodex microcarriers becomes a vital aspect of navigating through contemporary cell culture applications, urging researchers and industrial practitioners alike to harness their potential effectively.
Definition and Overview
Cytodex microcarriers are essentially small beads made from a variety of materials that provide a surface for cells to grow and multiply. These beads are typically characterized by a size ranging from 100 to 200 micrometers in diameter. They are designed to offer a high surface area while maintaining the physical properties necessary for optimal cell growth.
Cytodex microcarriers can be composed of different materials such as dextran, polystyrene, or even collagen that are tailored to specific applications. Their surface can be modified to increase binding affinity, thus catering to various cell types which demand different growth conditions. By forming a three-dimensional environment, these microcarriers allow for more natural cellular interactions compared to traditional two-dimensional cultures.
In terms of application, these microcarriers can be deployed in several sectors of research and industry. They are widely utilized in the production of vaccines, antibody production, and also in stem cell cultures, showcasing their versatility in bio-manufacturing.
History of Microcarrier Development
The journey of microcarrier technology is quite fascinating. It began in the late 1960s when researchers started to experiment with alternatives to conventional cell culture methods. The desire to improve the efficiency of cell growth led to the development of these tiny spheres which could provide more surface area for cells in a smaller volume of media.
Cytodex brand, launched by the Swedish company, has been a pioneer in this field, introducing microcarriers that are particularly effective for various mammalian cells. Over the decades, advancements in the formulation of these microcarriers have steadily evolved, with innovations focused on enhancing surface chemistry, physical properties, and cell attachment mechanisms to address the growing demands in scientific research and commercial applications.
Today, while novel technologies continue to emerge, understanding the foundational aspects of Cytodex microcarriers is essential for anyone engaged in cell culture, as it provides the groundwork for future innovations in the field.
Biological Significance of Microcarriers
Cytodex microcarriers hold a crucial position in modern cell culture techniques, shaping the landscape from laboratory to industrial applications. Their significance hinges on a variety of biological elements, particularly in how they support cell growth and function. To delve into this topic is to highlight their role not just as passive supports but as dynamic facilitators in cultivating diverse cell types.
Applications in Cell Culture
Microcarriers serve as a cornerstone in cell culture practices, enabling the growth of cells in suspension rather than on solid surfaces. This aspect is a game-changer. It allows for increased density and improved nutrient transfer. When utilizing Cytodex microcarriers, researchers can achieve cell concentrations that are often impossible on standard surfaces.
For example, in the production of vaccines, the ability to grow cells in a large volume using microcarriers means that pharmaceutical companies can scale up production quickly and efficiently. This efficiency is essential, particularly during health emergencies where rapid vaccine development is critical.
Additionally, microcarriers enhance the accessibility of various cell types, including stem cells and primary cultures, which often have specific growth requirements. Their unique design enables researchers to tailor conditions based on the specific cell type, fostering a conducive environment for optimal cell proliferation and differentiation.
Role in Biopharmaceutical Production
In the realm of biopharmaceutical production, Cytodex microcarriers play an indispensable role. They are a primary choice for producing a variety of biologics, such as monoclonal antibodies and recombinant proteins. The mechanism by which microcarriers function allows for a high surface area-to-volume ratio, which is crucial for maximizing cell attachment and productivity.
"The enhanced growth and productivity of cells on microcarriers can significantly reduce the time and cost associated with biopharmaceutical manufacturing."
Their importance cannot be overstatedโusing Cytodex microcarriers not only leads to improved yield but also amplifies the viability of cells during the production cycle. This is particularly pertinent when considering the sensitivity of certain cell lines to changes in their environment. By ensuring a stable growth platform, microcarriers help in maintaining cell health throughout the cultivation process.
Furthermore, as the industry pushes towards more personalized medicine approaches, the adaptability of microcarriers enables companies to fine-tune production processes for specific therapeutic needs. This responsiveness not only addresses the needs of traditional therapies but also accommodates emerging therapies tailored to individual patient profiles.
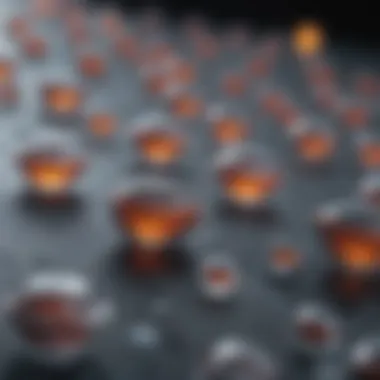
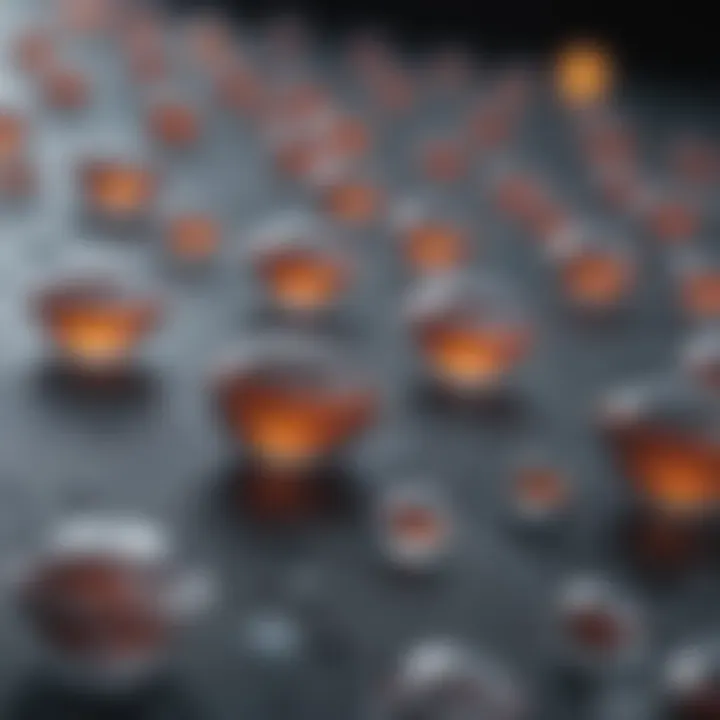
Properties of Cytodex Microcarriers
The properties of Cytodex microcarriers play a pivotal role in defining their functionality and effectiveness in cell culture applications. Understanding these properties is essential, as they influence factors like cell attachment, growth rates, and overall viability. The use of microcarriers in various bioprocesses hinges heavily on these properties, making it imperative for researchers and professionals to grasp their significance.
Material Composition
Cytodex microcarriers are primarily crafted from polystyrene, known for its ability to support robust cell growth. This material choice is no accident; polystyrene offers a combination of durability and biocompatibility that many cell cultures demand.
- Biocompatibility: Since microcarriers are meant to facilitate cell growth, their underlying materials need to be non-toxic and compatible with different cell types. Polystyrene helps in achieving this compatibility.
- Stability: Another essential aspect is that these microcarriers must maintain their shape and physical integrity during the culture process. Polystyrene provides stability and protects the cells from damage during harvesting.
- Surface Treatment: Many Cytodex products come with a pre-coating of collagen or other extracellular matrix components. This addition enhances adhesion for anchorage-dependent cells, making the transition to microcarrier culture smoother.
In summary, the material composition of Cytodex microcarriers is a crucial factor that directly affects cell growth dynamics, influencing both laboratory and industrial applications.
Surface Characteristics
The surface characteristics of Cytodex microcarriers cannot be overlooked. These properties dictate how well cells can attach and proliferate on the microcarriers. Adjustments in these characteristics can greatly affect the efficiency of the cell culture process.
- Surface Area: A greater surface area means more real estate for cell attachment, which is especially vital in high-density cultures. Cytodex microcarriers are specifically designed to maximize surface area without compromising handling and scalability.
- Texture: The texture of the surface can be tailored. A rougher surface may enhance cell attachment through increased micro-scale interactions, while a smoother surface can promote stronger shear forces, beneficial in specific condition.
- Functionalization: Advanced surface modifications can impart selective properties to microcarriers. For instance, incorporating adhesive molecules can promote the attachment of specialized cell types while discouraging attachment of others.
To better encapsulate the importance of these surface characteristics:
"The choice of surface characteristics in Cytodex microcarriers can significantly influence the success of a culture process, affecting everything from cell yield to the timing of harvest."
Mechanisms of Cell Attachment and Growth
Cell attachment and growth on microcarriers are pivotal components that significantly affect the efficacy of cell culture processes. Understanding these mechanisms can lead to optimized conditions, yielding higher cell viability and productivity. For researchers, educators, and industry professionals alike, knowing the intricacies of how cells interact with microcarriers like Cytodex can turn a good experiment into a great one.
Types of Cell Attachment
When it comes to how cells grip on microcarriers, different types come into play. In essence, these attachment strategies can be broadly categorized into:
- Physical Adsorption: This is the simplest form of attachment, which relies on the cells' ability to stick through van der Waals forces and electrostatic interactions. Though convenient, this method often lacks stability and can be influenced by varying environmental conditions.
- Biochemical Bonding: Here, cells attach through specific molecular interactions. For example, use of proteins like fibronectin can be employed, which actively promotes cellular adhesion. This method tends to enhance stability of the cell layer on microcarriers, making it more resistant to shear stress.
- Matrix-assisted Attachment: In certain setups, an extracellular matrix (ECM) may be used to facilitate cell attachment. This can be beneficial when working with sensitive cell types that require a more natural environment for attachment and growth.
When exploiting these attachment types, itโs crucial to tailor the method to the target cell's needs. For example, stem cells may thrive better with biochemical bonding, while adherent cells could prefer physical adsorption or matrix-assisted attachment.
Influence of Microcarrier Design
The design of the microcarrier itself vastly influences both attachment and growth dynamics. Several design facets come into play:
- Surface Area: A higher surface area can allow for more cells to attach, which is generally desirable. This can lead to improved cell yield, important for biopharmaceutical production.
- Shape and Size: Smaller microcarriers generally facilitate better nutrient and gas exchange; however, they may lead to difficulties in recovery processes. Getting the dimensions right is often a balancing act influenced by the type of cells used and the specific application.
- Surface Coating: Microcarriers can be coated with various materials to enhance cell interactions. For instance, aPEGylated surface may improve nutrient absorption while promoting better cell proliferation.
Ultimately, the relationship between microcarrier design and cell growth cannot be overstated. Each design component can either bolster efficiency or introduce challenges, thus demanding a thoughtful approach tailored to specific applications. A well-designed microcarrier will not just support cell attachment but will also create an ideal environment for growth, helping the cells reach their full potential in culture.
Effective cell attachment strategies are the backbone of successful microcarrier applications in cell culture, influencing yield, viability, and overall process efficiency.
Cytodex Microcarriers in Research
The relationship between Cytodex microcarriers and research is a dynamic one, crucial for understanding cell behavior in various conditions. These microcarriers provide a three-dimensional environment, mimicking in vivo conditions to an extent, which is vital for studies focused on cell growth and differentiation. They serve as an essential tool in both fundamental and applied research, allowing scientists to delve deeper into the complexities of cellular functions. Utilizing Cytodex microcarriers not only improves the results of experiments but also facilitates scalability, making them favorable for larger studies and potential industry applications.
Utilization in Stem Cell Research
Stem cell research is a field that benefits enormously from the unique properties of Cytodex microcarriers. These microcarriers create a surface that is conducive to stem cell attachment and proliferation. Unlike traditional two-dimensional cultures, the microcarrier system allows for a more physiologically relevant environment that can support the intricate behaviors of stem cells.
- Enhanced Survival: Stem cells grown on Cytodex microcarriers exhibit higher viability due to the improved nutrient diffusion and waste removal compared to static cultures.
- Expansion Efficiency: The surface area provided by the microcarriers allows for a denser population of stem cells. This contributes to higher yields, which is particularly important for research aimed at therapeutic applications.
- Versatility in Applications: Cytodex microcarriers can support various types of stem cells, whether embryonic or induced pluripotent stem cells, thereby broadening the scope of research that can be conducted.
"Cytodex microcarriers represent a pivotal innovation for stem cell research, enabling better scalability and relevance to actual biological conditions."
Application in Oncology Studies
Cytodex microcarriers also find critical applications in oncology studies, where the need for understanding cancer cell behavior in a simulated microenvironment is crucial.
- Tumor Microenvironment Simulation: By facilitating a three-dimensional culture, Cytodex microcarriers help replicate the tumor microenvironment more closely than conventional methods. This aids in studying tumor growth, metastasis, and response to treatments.
- Drug Testing: Utilizing microcarriers for growing cancer cells allows researchers to assess how these cells respond to various therapeutic agents in a setting that more accurately reflects real-life interactions.
- High-Throughput Screening: The scalability of cell cultures on Cytodex microcarriers makes it feasible to perform high-throughput drug screening, an essential step in the development of new cancer therapies.
In summary, the application of Cytodex microcarriers in research, particularly within stem cell and oncology studies, exemplifies their versatility and significant impact on scientific investigation. The capacity to create more relevant cell culture conditions not only enhances our understanding of cellular processes but ultimately paves the way for innovative treatments and therapies.
Optimizing Microcarrier Usage
Optimizing microcarrier usage is crucial for achieving efficient cell culture processes. As various cell types are sensitive to their growth environments, fine-tuning these parameters leads to better yields and cell viability. Knowing how to tailor cultivation conditions and nutrient requirements can significantly affect the overall performance of the microcarriers. This section highlights the importance of creating an optimal environment for cells cultured on Cytodex microcarriers, ensuring all factors work synergistically.
Cultivation Conditions
There are several key factors to consider when establishing the right cultivation conditions for microcarriers. Temperature, pH levels, and oxygenation represent core environmental parameters that demand attention. Deviating from desired conditions even slightly can lead to unfavorable outcomes, such as poor cell attachment or reduced cellular metabolism.
- Temperature: Cell lines exhibit various temperature tolerances. The most common temperature for mammalian cells hovers around 37ยฐC. A stable temperature gradient ensures optimal growth and metabolic activity, thereby enhancing productivity.
- pH Levels: Maintaining the right pH is vital for cell function. Most mammalian cells thrive between pH 7.2 to 7.4. Regular monitoring and timely adjustments can prevent pH drift, which could otherwise lead to cell stress or death.
- Oxygenation: The availability of oxygen significantly impacts the cellular respiration process. Cultures in larger volumes necessitate careful control of dissolved oxygen levels, which can be achieved using bioreactors with aeration systems. Ensuring a sufficient oxygen supply can enhance cell growth and metabolic functions.
In addition to these factors, the physical agitation of the culture medium also influences cell behavior. Balanced mixing encourages the uniform distribution of cells and nutrients, while over-agitation can result in cell detachment or breakage.
"Optimizing cultivation conditions maximizes the potential of microcarriers, transforming theoretical capacity into practical success."
Nutrient Requirements
Nutrient availability is yet another critical factor in optimizing microcarrier usage. For maximizing cell growth and productivity, the following components must be carefully evaluated:
- Basal Media: The choice of basal medium, such as DMEM (Dulbecco's Modified Eagle Medium) or RPMI-1640, can profoundly affect the growth rates of cells on microcarriers. Selecting a medium tailored to the specific cell line is essential for achieving desired results.
- Supplementation: Adding essential supplements, like fetal bovine serum (FBS) or growth factors, can enhance cell proliferation and functionality. Nonetheless, one must strike a balance; too much supplementation may lead to unwanted variables during cell processing.
- Continuous Feeding: Implementing a fed-batch culture technique allows for a continuous supply of nutrients. This strategy prevents nutrient depletion, thereby supporting sustained cell growth over longer periods.
- Metabolite Clearance: As cells grow, waste products accumulate and can hinder performance. Regular monitoring and timely removal of metabolites are crucial for maintaining optimal conditions.
In sum, understanding how to effectively manipulate cultivation conditions and nutrient requirements allows researchers to unlock the full potential of Cytodex microcarriers in their cell culture applications. The focus on optimizing these elements can dramatically influence the efficiency and success of various bioprocesses involving microcarriers.
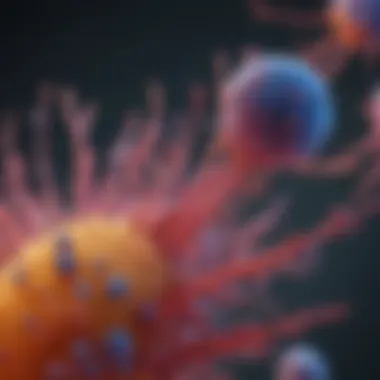
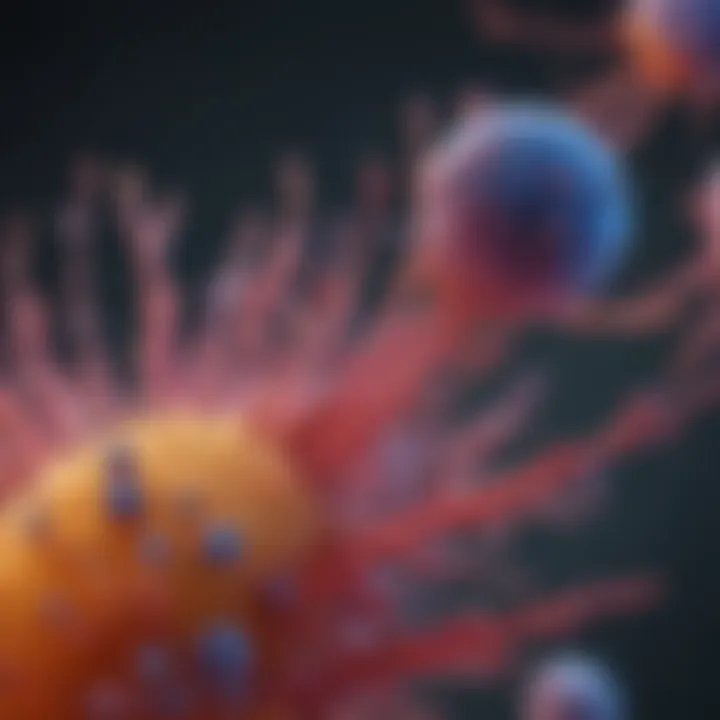
Challenges in Microcarrier Applications
In the evolving field of cell culture technology, the utility of microcarriers cannot be overstated. However, using Cytodex microcarriers comes with a unique set of challenges that require careful consideration and adaptability. Understanding these hurdles is crucial for researchers and practitioners alike, as solutions can have a ripple effect on the efficiency and success of various bioprocesses. By recognizing these challenges, stakeholders can better devise strategies to mitigate them, ensuring the advancement of cell culture methodologies.
Scale-Up Issues in Bioprocessing
Scale-up is often touted as the Achilles' heel in bioprocessing. Transitioning from lab-scale to production-scale introduces intricate complications, not least of which is the challenge of maintaining optimal conditions for cell growth. As the scale increases, factors like shear stress, nutrient distribution, and oxygen transfer become paramount.
In smaller volumes, cells can retrieve nutrients efficiently, and wastes can be easily expelled. But scaling up these conditions means that the microcarrier dynamics can change significantly, leading to potential bottlenecks in performance.
For instance, consider a bioreactor of 10 liters versus one of 100 liters. The turbulence and agitation needed to keep microcarriers suspended become more complexโand sometimes less effectiveโas the volume increases. This can cause uneven cell distribution and reduced yield.
Here are a few specific concerns with scaling up:
- Nutrient Gradient Formation: As volume increases, the concentration gradient of nutrients can vary widely, leading to regions of nutrient deprivation.
- Mechanical Stress: Cells may experience different mechanical stresses in larger systems, which can affect their growth and attachment to microcarriers.
- Risk of Contamination: Higher volumes and increased manipulation raise the likelihood of contamination, which is a significant concern in cell production settings.
Addressing these issues often involves designing bioreactors with tailored mixing systems and monitoring setups that can adapt not just to the scale but also to the cell type being cultured. Innovative approaches, including the use of computational fluid dynamics models, can assist in predicting flow patterns and optimizing bioreactor performance.
Cell Detachment Problems
Cell detachment is another nagging issue that rears its head during microcarrier applications. The ideal scenario involves strong initial attachment to facilitate growth, but when it comes time for harvesting, that same attachment can become a liability.
A fundamental aspect is ensuring that cell-to-microcarrier interactions are optimized, allowing for secure attachment while also incorporating methods for efficient detachment later. Cells might resist leaving their microcarrier homes due to a variety of bonding phenomena, from biological factors to the specifics of microcarrier material and surface treatment. Non-adherent cells or delicate primary cells particularly struggle in conditions that promote detachment. Inadequate techniques might lead to low yields of viable cells, which in turn affect downstream applications like therapeutic product development.
Some noteworthy facets of cell detachment include:
- Mechanical Forces: Current harvesting methods utilize shear forces to detach cells. However, if applied too vigorously, this can compromise cell viability.
- Temperature and pH Changes: Fluctuating environmental parameters can also impact detachment kinetics, making consistency a challenge.
- Surface Treatment: Depending on the microcarrierโs coating, detachment properties can vary. Newer technologies look towards custom surface modifications to enhance the harvest process without sacrificing cell integrity.
Strategies for navigating this challenge often blend established techniques with innovative technologies, such as enzyme-based detaching agents or thermal shocks, to help efficiently liberate the desired cells while maintaining their health.
In summary, challenges in the application of Cytodex microcarriers are multifaceted, affecting various stages of the cell culture processโfrom scaling up bioprocesses to the critical detachment steps. Addressing these intricacies not only enhances yield but also promotes advancements in the industry, facilitating a more robust understanding of cellular behaviors in culture.
Innovations in Microcarrier Technology
In the world of cell culture, microcarriers are essential players. As the demand for efficient cell production process continues to surge, innovations in microcarrier technology have attracted significant attention. These advancements are not just bells and whistles; they directly impact how we cultivate cells, influence cell yield, and suit the ever-evolving needs of research and industry. This section will delve into two pivotal innovations: surface modifications and biomimetic approaches.
Surface Modifications
Surface modifications to microcarriers serve as a game changer in enhancing cell attachment and proliferation. Traditional microcarriers often lack the necessary features conducive for optimum cell growth. By modifying the surfaceโa process that can include coating with biological molecules or altering porosityโresearchers can dramatically boost the interaction between cells and microcarriers.
Benefits of these modifications include:
- Improved Attachment Rates: Adjusting the surface chemistry can lead to stronger and more efficient cell attachment.
- Customization for Different Cell Types: Not all cells are created equal, and modifying surfaces allows microcarriers to cater to specific cell lines, facilitating more precise experimentation.
- Enhanced Environmental Stability: Alterations can provide better protection against environmental stresses, thus preserving cell integrity during culture.
As an example, a study by Habib and colleagues demonstrated that microcarriers coated with collagen not only provided a more favorable environment for mesenchymal stem cells but also improved their viability and proliferation rates compared to uncoated counterparts. This demonstrates the vast potential that surface modifications bring to the table.
Biomimetic Approaches
Biomimetic strategies aim to mimic natural cellular environments, providing microcarriers that closely replicate the in vivo conditions under which cells thrive. This approach acknowledges that cells are not just isolated entities but exist within complex ecosystems. By creating microcarriers that reflect these natural habitats, the chances for successful cell cultures increase.
Key considerations for biomimetic approaches include:
- Integration of Extracellular Matrices: Many research efforts are focusing on incorporating materials similar to the extracellular matrix which cells interact with in their natural settings. This can enhance functionality and increase cell performance.
- Dynamic Interactions Between Cells and Microcarriers: Developing microcarriers that facilitate process interactions, like fusion or co-culturing with other cell types, can drive novel findings in research.
- Enhanced Sensing and Feedback Mechanisms: Innovations do not solely revolve around structure; integrating biosensors into microcarriers can also monitor real-time changes in cellular responses, further informing the cultivation process.
An example of this innovation comes from recent advances in the production of microcarriers that utilize gelatin, which is a naturally derived polyamine. This material has shown promise in providing cues that lead to enhanced cell behavior when compared to synthetic alternatives.
Comparative Analysis with Other Microcarriers
When it comes to enhancing cell culture, itโs crucial to understand the competitive landscape involving different microcarriers. This aspect not only showcases the strengths and weaknesses of Cytodex microcarriers, but it also gives us a clearer picture of how they fit into the broader context of cell culture technologies. By comparing Cytodex with other microcarriers, researchers and practitioners can adopt practices that lead to improved outcomes in their specific applications.
Differentiation Between Various Brands
In todayโs market, various brands offer a plethora of microcarriers, each with its own unique features. Brands like Cytodex, Thermo Scientific, and Eppendorf come to mind. While Cytodex microcarriers stand out due to their well-characterized surfaces and biological compatibility, other brands have carved their niches by addressing specific cultivation challenges. For instance:
- Thermo Scientific features microcarriers that are specially designed for robust scaling and optimized for mammalian cells.
- Eppendorf's microcarriers focus on providing enhanced cell attachment through distinct surface modifications that suit specific applications.
Consequently, understanding these differentiating factors helps researchers select a product that meets their specific needs, such as yielding higher cell densities or creating a suitable environment for stem cell culture. Each brandโs approach to microcarrier design can significantly affect cell growth dynamics, influencing parameters like attachment rates, yield, and overall productivity in biopharmaceutical manufacturing.
Performance Metrics in Cell Culture
The efficacy of a microcarrier in cell culture can largely be gauged through several performance metrics. These include:
- Cell Yield: The total number of viable cells harvested plays a pivotal role in determining the success of any cell culture process. Cytodex microcarriers, for example, have shown superior cell yield in various studies compared to certain competitors.
- Viability Rates: Itโs crucial for a microcarrier to support not just growth, but also maintain cell viability throughout the culture duration. Understanding how different carriers affect cell viability can lead to better application choices.
- Attachment Efficiency: Microcarriers must promote optimal cell attachment. Certain formulations may outperform others based on surface characteristics or material properties. Research has indicated that variations in surface modifications among brands significantly affect attachment efficiencies.
"In the world of cell culture, choosing the right microcarrier is akin to selecting the right stage for a play. The actors' performance is deeply influenced by their environment."
In summary, a thorough comparative analysis allows for informed decision-making when it comes to selecting microcarriers. Cytodex microcarriers offer valuable benefits, yet understanding the full breadth of options available can ensure that researchers and industry stakeholders make choices best suited for their particular applications.
Future Trends in Microcarrier Development
Understanding the future trends in microcarrier development is vital as it paves the way for new innovations and enhancements in cell culture methodologies. With the increasing demand for efficient bioprocessing, the evolution of Cytodex microcarriers will reflect advancements in technology, material science, and applications tailored for specific cell types. While the field is ever-changing, certain elements stand out, notably in terms of benefits and considerations that constitute the backbone of future developments.
Emerging Technologies
The role of emerging technologies in microcarrier development is crucial. As researchers explore advanced materials, the potential for more biocompatible and efficient microcarriers increases. For instance, the integration of 3D printing technology is making waves. This approach allows for the creation of custom microcarriers that can be tailored to meet specific needs regarding thickness and structure. Imagine microcarriers being designed to mimic the exact extracellular matrix of particular tissues. The adaptation of nanotechnology also holds promise, enabling microcarriers to be engineered on a nanoscale for enhanced attachment and growth capabilities.
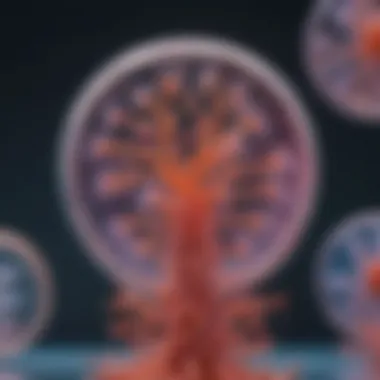
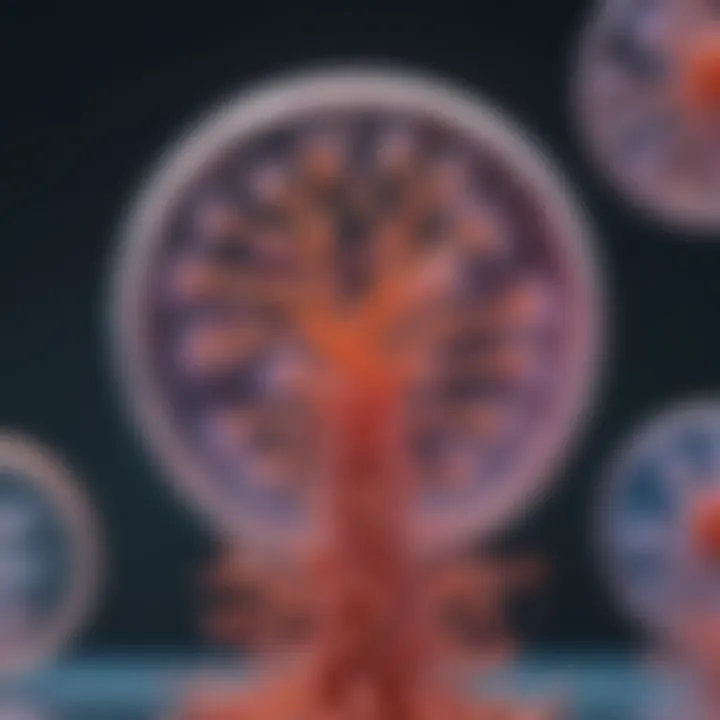
Benefits of these emerging technologies include:
- Customization: Researchers can tailor microcarriers to optimize contact surfaces and adapt facilitation for different cell types.
- Efficiency: Newly developed microcarrier designs focus on maximizing surface area, which can lead to improved cell yields.
- Scalability: Advanced techniques can potentially reduce costs and speed up cell manufacturing processes, making them more accessible in various industries.
The integration of these technologies marks a significant leap toward achieving highly specialized microcarriers that cater to specific cell lines effectively.
Predicted Advances in Applications
Looking ahead, we can expect that advances in the applications of microcarriers will not only enhance existing processes but also open up new avenues in biotechnology and medicine. There is a growing consensus that increased understanding of disease mechanisms will lead to the custom application of Cytodex microcarriers for therapeutic purposes, specifically in the fields of regenerative medicine and vaccine production.
To elaborate, here are some predicted advances in practical applications:
- Regenerative Medicine: Tailored microcarriers could facilitate stem cell therapies, where the microcarrier design promotes better cell differentiation and survival.
- Vaccine Production: Using microcarriers for the growth of various cell lines involved in vaccine production may lead to more expedited processes, ultimately improving accessibility to vaccines during pandemics.
- Gene Therapy: In the context of gene therapy, microcarriers could serve as delivery vehicles, providing a controlled environment for the transfer of genetic materials into target cells.
Cytodex Microcarriers in Commercial Use
Cytodex microcarriers have found a significant niche in commercial cell culture applications. As industries push for more efficient and scalable solutions, microcarriers like Cytodex have risen to meet the demand. This section unpacks how Cytodex microcarriers contribute to larger scale operations, offering both biological and economic advantages that are crucial for commercial success.
Case Studies in Industry
When we look at practical applications, there are compelling case studies highlighting how Cytodex microcarriers have been successfully integrated into various industrial processes:
- Biopharmaceutical Production: One of the concrete examples is found in the production of monoclonal antibodies. Companies like Genentech have utilized Cytodex microcarriers in their bioreactors. This method has allowed them to increase the cell density and production yield compared to traditional adherent surfaces.
- Vaccine Development: During the initial phases of COVID-19 vaccine development, manufacturers moved swiftly to scale up production. Utilizing Cytodex microcarriers played a pivotal role in enhancing the growth conditions for the required cell lines, leading to a faster turnaround for research and production.
- Tissue Engineering: Firms working on regenerative medicine, such as Organovo, have leveraged Cytodex microcarriers in their sculpting of tissues. This approach provides a scaffold that encourages cell attachment, growth, and eventual differentiation, which is fundamental for creating functional tissues.
These instances showcase how Cytodex microcarriers aren't just theoretical tools; they present real solutions in high-stakes environments where efficiency and quality can't be compromised.
Market Overview
As the market landscape evolves, thereโs a growing demand for tailored cell culture solutions. Key elements in the market overview of Cytodex microcarriers include:
- Demand Surge: The global market for microcarriers is expected to grow substantially, with estimates predicting a shift towards a multi-billion-dollar industry within the coming years.
- Product Diversity: Cytodex offers different variants designed for specific applications, highlighting their versatility in meeting diverse industry needs. For instance, Cytodex 1 and 3 have specific surface modifications suitable for various cell types and applications.
- Competitive Landscape: Firms engaged in microcarrier production, such as Thermo Fisher Scientific and Merck, have also recognized the advantages of Cytodex, which helps maintain healthy competition and drives innovation.
- Sustainability Trends: Moreover, there is a noticeable shift towards sustainable practices. Companies are more frequently considering the environmental impact of the materials used in production. Cytodex microcarriers, which can be sourced from biodegradable materials, meet this growing demand.
In summary, Cytodex microcarriers not only optimize cell culture processes but have also carved a substantial space in the commercial market, responding adeptly to the challenges and opportunities of todayโs biotechnology landscape.
Environmental and Economic Considerations
In today's world, where sustainability plays a crucial role in various industries, the environmental and economic facets of using Cytodex microcarriers in cell culture are gaining attention. These microcarriers significantly impact not just cell growth efficiency but also present opportunities and challenges with respect to ecological footprints and financial aspects. Understanding these dimensions is valuable for researchers and industry stakeholders alike, as it guides decisions towards more responsible practices.
Sustainability in Materials
Cytodex microcarriers are primarily composed of materials that can have a varied impact on the environment. Their formulation often includes polystyrene, which may raise eyebrows regarding environmental sustainability. However, manufacturers are increasingly focused on developing microcarriers that incorporate more environmentally friendly ingredients. The use of biodegradable polymers, for instance, is a step towards reducing waste in laboratories and production facilities.
Adopting sustainable materials is not just beneficial for the environment; it can also elevate a company's public image. An organization that promotes eco-friendly practices is likely to attract clients and consumers who prioritize sustainability. Furthermore, using materials that align with regulations concerning environmental impact can mitigate the risk of fines and compliance issues.
- Examples of emerging sustainable materials include:
- Biodegradable compounds like poly(lactic acid) (PLA)
- Natural polymers such as alginate and chitosan
Conducting life cycle assessments helps in identifying the environmental impact from the production of microcarriers to their disposal. This thorough evaluation indicates areas for improvement and drives innovation in sustainable practices. Thus, when considering the transition to sustainable microcarriers, awareness of these materials becomes indispensable for market competitiveness and ecological responsibility.
Cost Implications for Research and Industry
While sustainability remains a concern, the economic implications of using Cytodex microcarriers cannot be overlooked. The lack of cost-effective solutions can hinder research, especially in budget-constrained environments.
Several factors come into play when assessing the financial impact of microcarrier usage. The initial investment for high-quality microcarriers may seem steep. However, this should be weighed against the long-term benefits they offer, such as increased cell yield and easier scalability of cell culture processes.
- Cost considerations include:
- Upfront purchase price of microcarriers
- Cost of necessary media and supplements
- Operational costs related to maintenance and monitoring
Ultimately, leveraging the right microcarriers can result in significant savings when one considers the overall productivity in the long run. Proper planning and selection strategies are essential to ensure that the adoption of microcarriers aligns with budget constraints while maximizing efficiency.
"Integrating sustainability into the research workflow is not merely a trend; it's a lasting tradition that has the potential to reshape how we approach biotechnology."
Ending and Future Directions
Conclusively, the exploration of Cytodex microcarriers unveils a myriad of possibilities that permeate the realms of cell culture. These microcarriers are not simply passive supports; they play a vital role in both fundamental research and the burgeoning field of biopharmaceutical production. The significance of recognizing their utility cannot be overstated, as advancements in the design and application of these diminutive but powerful tools have the potential to substantially revolutionize methodologies in cell culturing. Understanding these implications leads to enhanced efficiency, higher productivity, and better yields in cellular processes.
The burgeoning interest in sustainable practices, along with the constant evolution of bioprocessing techniques, forms a bedrock for future investigation into Cytodex microcarriers. Incorporating greener materials and techniques could lead to improved ecological outcomes in an industry often criticized for its environmental footprint. Additionally, understanding the biocompatibility and degradation of microcarriers presents another layer of innovation worth considering.
Future research should particularly focus on:
- Enhanced surface modifications: New coatings or structures that mimic natural extracellular matrices can significantly affect cell adhesion.
- Technological integration: Embracing automation and real-time monitoring can refine cell culture parameters, allowing for the optimization of conditions on-the-go.
- Exploring niche applications: Beyond traditional cell lines, examining specific lineage cells could unearth unique insights into their growth dynamics in microcarrier settings.
"The promise of Cytodex microcarriers lies not only in their current applications but also in the expansive horizon of biopharmaceutical advancements they facilitate."
By fostering a culture of inquiry aimed toward these areas, professionals can steadily push the boundaries of what is achievable in cell culture. Engaging with this evolving landscape necessitates collaboration among researchers, industry professionals, and educators to harness collective insights that can drive innovation. The potential to develop more robust, efficient, and sustainable practices within biotechnology hinges on these future directions alongside the understanding gained throughout this article.
Summary of Findings
Throughout this article, we have navigated through the critical landscape of Cytodex microcarriers, emphasizing their importance in cell culture. Key points include:
- Diverse Applications: Their utility spans various fields, from therapeutic production to research-focused investigations, illustrating their versatility.
- Material and Surface Innovations: Understanding their composition and surface properties aids in optimizing performance for specific cell types.
- Challenges and Innovations: Despite hurdles like scale-up issues and cell detachment, ongoing innovations provide a pathway to improved methodologies.
The synergy between traditional and new technologies marks a promising future for these microcarriers.
Call for Further Research
Thereโs no denying that the landscape of biotechnology is ever-shifting, and Cytodex microcarriers sit squarely at the intersection of tradition and innovation. Therefore, a pressing call for further research is paramount. Here are focused areas where more attention and investigation are required:
- Longitudinal Studies: Tailoring studies over extended periods can reveal how cells adapt and thrive over time in microcarrier systems.
- Cross-disciplinary Approaches: Engaging biologists, material scientists, and engineers may yield fresh perspectives and enhance the development of unique microcarrier designs.
- Real-world Applications: Exploring how microcarriers function inside living systems vs. controlled lab environments can provide unparalleled insights, aiding in the translatability of research findings to clinical practices.