Contemporary Research Directions in Physics
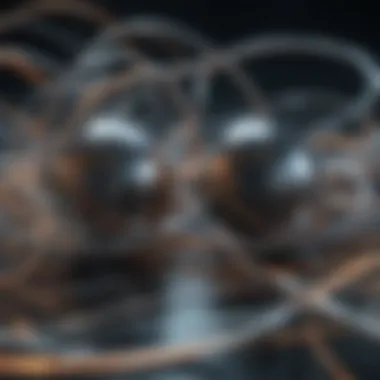
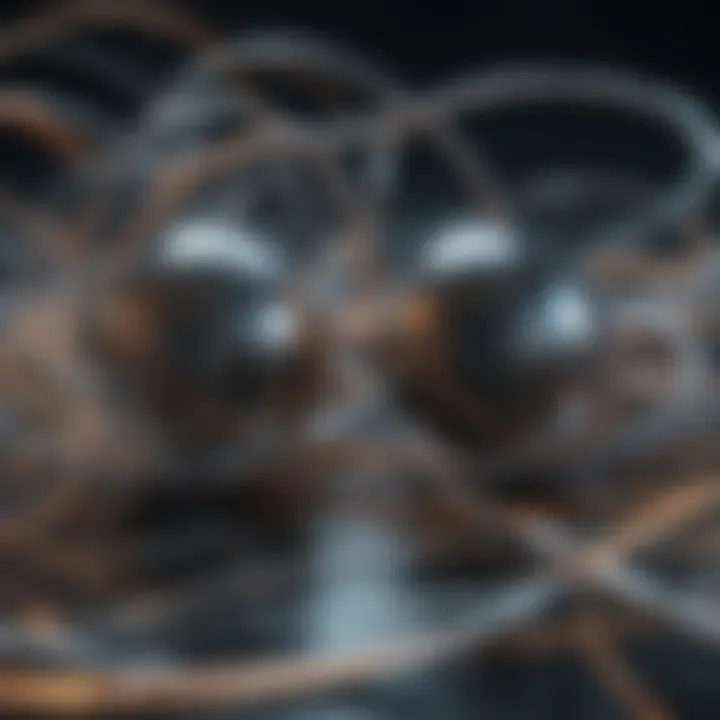
Intro
Physics, at its core, is the language of the universe. It paints a picture of the fundamental principles that govern everything around us. As we dive into the contemporary research topics within this vast field, we see a landscape filled with innovation and exploration—bridging the gap between what we know and what remains to be discovered. The quest to understand our reality has never been more vibrant.
Research in physics is not merely an academic exercise; it has implications that ripple through technology, philosophy, and even ethics. The subjects explored range from the tiniest particles to the grandest cosmic structures. This interplay creates a dynamic environment where researchers constantly seek to push the boundaries of human understanding.
In this article, we will navigate several critical areas of modern physics research. Each topic will be unpacked to reveal the methodologies employed, key findings, and how these connect to previous studies. The journey through classical mechanics, thermodynamics, quantum phenomena, and beyond promises to be as enlightening as it is challenging.
Research Overview
Summary of Key Findings
In the exploration of current research topics, we've observed a few stand-out areas:
- Quantum Computing: This area has burgeoned in recent years, leveraging quantum bits to solve problems impossible for classical computers.
- Dark Matter and Dark Energy: While traditional physics struggles to pinpoint these mysterious components of the universe, recent studies aim to better understand their influence on cosmic structures.
- Gravitational Waves: The detection of these waves has opened doors to new astronomical phenomena, enhancing our understanding of black holes and neutron stars.
Each of these subjects holds significant merit, not just theoretically but also in their practical applications, be it in advanced computing or astrophysics.
Methodologies Employed
The approach researchers take in tackling these significant topics can vary widely, embracing both theoretical mathematics and empirical experiments. Some methodologies include:
- Experimental Techniques: Ranging from particle accelerators to space telescopes, experiments are designed to observe phenomena that can’t be replicated in controlled environments.
- Mathematical Modeling: Heavily relied upon for fields such as cosmology and quantum mechanics, mathematical models help simulate complex interactions.
- Computational Simulations: Advances in technology have made it possible to simulate various physical phenomena that were previously thought too difficult to analyze.
By employing such diverse methodologies, physics continuously evolves, answering deeper questions about existence itself.
In-Depth Analysis
Detailed Examination of Results
The results emerging from contemporary physics research warrant meticulous examination. For example, in quantum computing, recent studies have shown that quantum entanglement can be harnessed for information transfer at unprecedented speeds. This finding is particularly relevant as it challenges the classical limit imposed by the speed of light, prompting reevaluation of established scientific principles.
Comparison with Previous Studies
When we compare these findings with studies from a decade ago, the contrast is striking. Previously, quantum computing was regarded mainly as a theoretical concept. In contrast, we now see tangible prototypes emerging from companies like IBM and Google, who are turning the abstract into reality.
“The evolution from theory to applied science in such a short span is proof of the dynamic nature of contemporary physics.”
Ending
The ongoing exploration of these contemporary research topics reflects not just a thirst for knowledge but also a commitment to understanding the fabric of reality. By interlinking theoretical insights with practical methodologies, physicists today are shaping the future of both science and technology. The full spectrum of research in this field continues to inspire and challenge, pushing us to reconsider what we know about the universe.
Fundamentals of Physics Research
Understanding the fundamentals of physics research serves as the bedrock for grasping modern advancements in this incredibly vast field. This section aims to elucidate the significance of foundational knowledge and its implications for contemporary explorations in physics. Without a firm grasp of basic principles, diving into complex theories can feel akin to threading a needle in the dark; one may possess the tools, yet remains oblivious to their function. Therefore, this portion of the article emphasizes not just what physics encompasses but also the importance of historical context and its ongoing evolution.
Definition and Scope
Physics is not merely a collection of formulas and concepts; it’s a lens through which we can comprehend the universe. At its core, physics is defined as the natural science concerned with the study of matter, energy, and the fundamental forces that govern their interactions. Unlike many other sciences, physics seeks to unify diverse topics under broad principles — an essence that can be likened to finding patterns in a chaotic painting.
The scope of physics is extensive, with its definitions evolving over time. From classical mechanics, where objects are analyzed under straightforward conditions, to modern physics, which delves into quantum mechanics and relativity, the field spins a rich tapestry of interrelated ideas. Each branch contributes to our understanding:
- Classical Mechanics: Deals with the motion of objects and the forces acting upon them.
- Thermodynamics: Focuses on the principles governing heat and energy transfer.
- Electromagnetism: Explores the forces between electrically charged particles.
- Quantum Physics: Investigates phenomena at the atomic and subatomic levels.
In a nutshell, the definition of physics continues to broaden as new discoveries challenge old paradigms, making it essential for aspiring physicists to remain flexible and open-minded.
Historical Context
To fully appreciate the modern landscape of physics research, it's vital to understand its historical development — a journey marked by lightbulb moments and paradigm shifts. From the early musings of ancient thinkers like Aristoteles, to the revolution brought about by Newton’s laws of motion, each epoch has laid down new tracks for the explorations that followed.
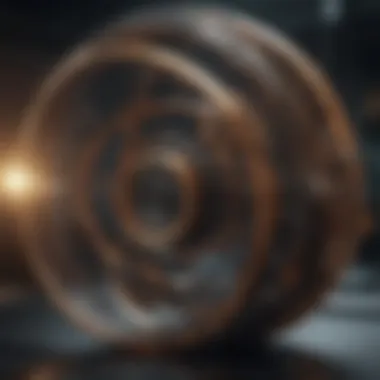
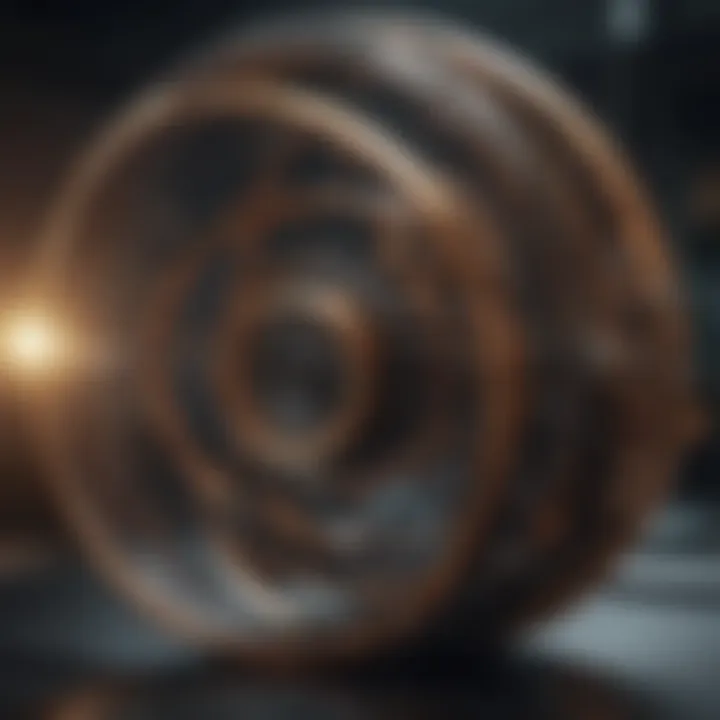
In the late 19th and early 20th centuries, physics took some giant leaps with the advent of electromagnetism and the theory of relativity crafted by Einstein. These theories not only transformed scientific thought but also had real-world implications—changing how we perceive time and space.
Moreover, the 20th century brought quantum physics to the forefront, revealing a bewildering world where particles behave in unexpected ways. It demonstrates that the deeper one probes into the nature of reality, the more intricate and profound the findings become.
"Physics is the poetry of the sciences. It gives words and forms to the otherwise unexplainable mysteries of the universe."
Foundational knowledge rooted in these historical insights not only enriches one's understanding but also sets the stage for contemporary questions. Just as maps evolve with our understanding of territories, the lexicon and frameworks of physics transform as we uncover new truths about existence.
By delving into the fundamentals, researchers today stand on the shoulders of giants, thereby empowering themselves to tackle contemporary challenges in physics with the wisdom gleaned from history.
Contemporary Areas of Physics Research
Contemporary physics research is like a treasure trove filled with a variety of quests for knowledge. Each area offers a unique blend of challenges and promises insights that can shape our understanding of the universe. This section digs into certain key fields of physics research that are gaining attention today, providing perspectives that can benefit students, educators, and professionals alike. The beauty of modern physics lies not only in its complexities but also in how these areas are interlinked, often addressing problems that require multidisciplinary approaches.
Astrophysics and Cosmology
Astrophysics and cosmology remain at the forefront of modern physics thanks to their ability to explore vast realms of existence. Questions about the universe's origin, structure, and ultimate fate sit at the core of human curiosity.
Dark Matter and Energy
Dark matter and energy contribute greatly to our current understanding of the cosmos. Characterized by their elusive nature, dark matter is believed to account for approximately 27% of the universe's mass-energy content, while dark energy constitutes about 68%. This highlights their profound impact on the universe's expansion and structure. Their study provides indirect access to phenomena that cannot be observed directly, making it a popular choice in astrophysical exploration.
As researchers delve deeper, the complexity of distinguishing between dark matter and energy poses ongoing challenges, yet it also opens avenues for novel theories and methods.
Gravitational Waves
Gravitational waves have literally taken the physics community by storm recently and they are a perfect example of how emerging technologies are changing research. The first direct detection of these ripples in spacetime in 2015 marked a milestone, allowing scientists to explore cosmic events like merging black holes and neutron star collisions. This burgeoning field not only confirms aspects of Einstein's theories but also unleashes a new way to observe the universe.
Studying gravitational waves enables researchers to examine phenomena that are hardly detectable through traditional optical or radio waves, providing invaluable details about the origins of the universe.
Exoplanetary Studies
Exoplanetary studies focus on planets outside our solar system, enthralling physicists and astronomers alike. The ability to detect, analyze, and characterize exoplanets offers insight into planetary systems' formation and composition. As space telescopes with advanced technologies continue their observations, understanding these distant worlds has become more attainable.
The search for Earth-like planets in habitable zones is a particularly compelling aspect of this research area, as it raises questions about the potential for life beyond Earth.
Quantum Mechanics
Quantum mechanics represents a fundamental shift in how we perceive the physical realm, having profound implications in various fields. It challenges our classical intuitions about matter, energy, and information, making it a cornerstone of contemporary research.
Quantum Computing
Quantum computing stands out for its potential to revolutionize data processing and problem-solving paradigms. By employing quantum bits, or qubits, these computers can perform calculations that would be impossible for classical machines. While the technology is still in its infancy, its implications for fields like cryptography, optimization, and drug discovery highlight its importance in modern physics research. As this field evolves, challenges such as developing stable qubits and efficient error correction methods need to be addressed.
Entanglement Studies
Entanglement, a phenomenon where the state of one particle is directly related to that of another regardless of the distance, is a key feature of quantum mechanics that continues to baffle scientists. The study of entangled particles not only has implications for fundamental physics but also exciting applications in quantum information technologies such as secure communication.
This area of research is particularly attractive due to its blend of theoretical and experimental challenges, pushing the boundaries of what’s known.
Quantum Majorana Fermions
Quantum Majorana fermions stand out for their intriguing properties and potential uses in quantum computing. These particles are their own antiparticles and could play a critical role in developing topological qubits, which are expected to be more robust against errors. The hunt for these fascinating particles leads researchers to explore new materials and experimental setups, making it a beneficial choice for this narrative on contemporary physics. While the benefits are significant, the experimental realization of Majorana fermions is still a tough nut to crack.
Condensed Matter Physics
Condensed matter physics focuses on the properties and behaviors of solid and liquid matter, offering a wealth of insights critical to technological advancement and theoretical understanding.
Superconductivity
Superconductivity is fascinating because it allows materials to conduct electricity without resistance at very low temperatures, making it a vital area of research. This characteristic not only fosters advances in magnetic levitation and medical imaging devices like MRI but also holds promise for energy transmission with minimal loss.
However, finding practical applications at room temperature remains an elusive challenge, keeping the research vibrant and dynamic.
Topological Materials
Topological materials exhibit peculiar electronic properties due to their underlying structures. These materials are essential for the development of future electronic devices and may play a vital role in quantum computing. The unique aspect of these materials lies in their ability to conduct electricity on their surfaces while acting as insulators internally, making them a popular choice for exploration.
Yet, understanding these properties in practical applications is still a work in progress, maintaining the engagement of the scientific community.
Nanotechnology Applications
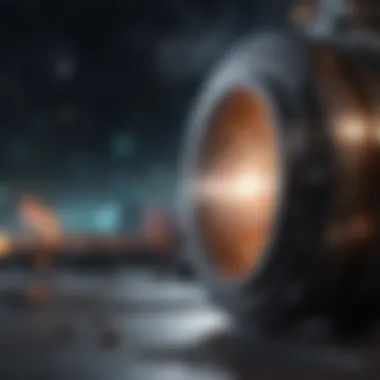
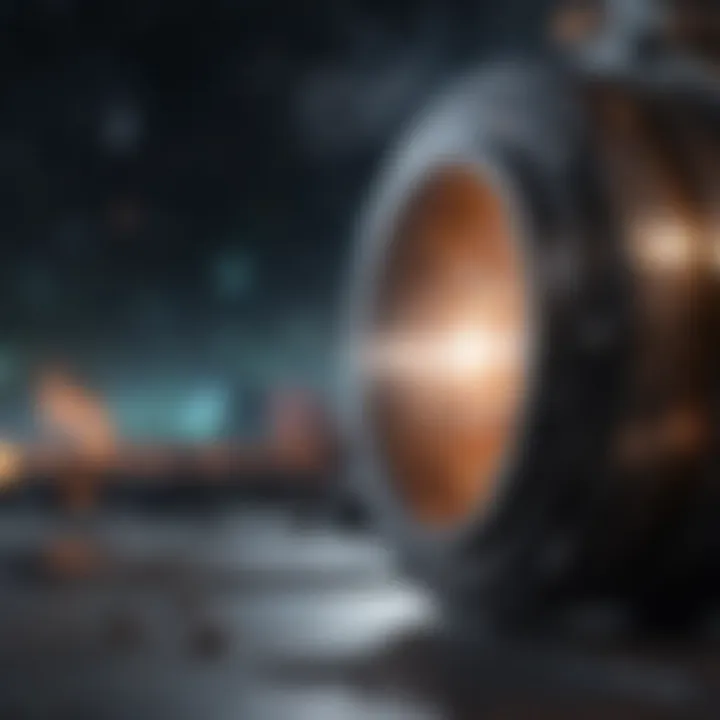
Nanotechnology is all about manipulating matter on an atomic, molecular, and supramolecular scale. Its applications ripple through various sectors, including medicine, electronics, and materials science, making it paramount in modern physics. Nanotech allows for the creation of smarter, more efficient devices by leveraging properties unique to nanoscale materials, making this area a focal point in contemporary research.
The main challenge in this field lies in ensuring that the benefits of these innovations can be safely realized in real-world applications.
Plasma Physics
Plasma physics deals with ionized gases—the fourth state of matter—and is crucial for various technological advancements and our understanding of the universe.
Thermonuclear Fusion
Thermonuclear fusion, the process that powers stars, holds the potential to deliver nearly limitless and clean energy. Research in this realm attempts to replicate the conditions found in the sun, presenting not only a technical challenge but also an opportunity for sustainable energy solutions.
The primary challenge remains in creating the necessary conditions for sustained fusion reactions, which ensures a long-lasting engagement of researchers in this quest.
Space Plasmas
Space plasmas present another captivating area of research, as they are crucial to understanding solar winds and their interactions with Earth’s magnetic field. Studies in space plasmas reveal insights about cosmic phenomena, enhancing our understanding of astrophysics. Utilize of this research helps to predict space weather events that can affect satellite communications and power grids.
Industrial Applications
The industrial applications of plasma physics have been growing, especially in fields like semiconductor manufacturing and material processing. The precision that plasmas provide can lead to improved performance and durability of materials.
While the benefits are evident, the complexities involved in scaling up plasma technologies for commercial use remains a significant consideration.
Engaging with these contemporary areas of physics research allows us to understand the world around us in intricate detail. Each research field contains unique elements that not only contribute to scientific knowledge but also have tangible impacts on technology, society, and our future.
Interdisciplinary Approaches
The realm of physics is not confined to its own boundaries; it often intertwines with other disciplines, resulting in what is termed interdisciplinary approaches. Such intersections broaden the scope of research and innovation. By incorporating elements from fields like biology and chemistry, physicists are uncovering novel insights that can push the envelope of traditional physics research. The beauty of this interdisciplinary collaboration is that each discipline brings its strengths to the table, potentially leading to groundbreaking discoveries and applications.
Physics in Biology
Biophysics
Biophysics stands out as a specialized field that applies the principles of physics to biological processes. One significant aspect of biophysics is its focus on understanding the physical mechanisms underlying life at the molecular level. This includes studying the dynamics of proteins, the mechanics of cellular processes, and other phenomena that fundamentally govern biological functions. The key characteristic of biophysics is its quantitative approach—it uses mathematical models to describe complex biological systems, making it a compelling choice for researchers. One unique feature of biophysics is its ability to bridge the gap between theoretical frameworks and practical applications, thus enhancing our understanding of health and disease. However, challenges include the complexity of biological systems, which can sometimes lead to complications in modeling.
Medical Physics
In the medical realm, medical physics plays a crucial role in the development and application of imaging technologies and treatment methods, especially in cancer treatment through radiation therapy. It connects the fundamental concepts of physics to practical healthcare solutions. The ability to apply physics concepts to improve diagnostic imaging, such as MRI and CT scans, makes medical physics invaluable in modern medicine. A distinctive aspect of medical physics is the ongoing necessity for collaboration with healthcare professionals to ensure effective applications of technology, which promotes better patient outcomes. Nevertheless, the field also grapples with ethical issues related to patient safety and the impact of radiation exposure.
Biomaterials
Biomaterials serve as another significant intersection of physics and biology. These materials, designed to interact with biological systems, are increasingly used in medical devices, prosthetics, and tissue engineering. The key characteristic of biomaterials is their ability to mimic biological properties, which is fundamental in enhancing the compatibility and functionality of medical implants. They have unique features—such as being biocompatible and degradable—allowing them to be safely integrated into living systems. However, challenges exist in ensuring long-term functionality in the body while minimizing immune responses, which necessitates ongoing research and innovation in the field.
Physics in Chemistry
Physical Chemistry Research
Physical chemistry encompasses the study of how physical principles govern chemical systems. This field blends physics with chemistry to explore complex topics such as reaction kinetics and thermodynamics. The fusion of these disciplines leads to a deeper understanding of chemical processes at a fundamental level. The key idea is how energy changes within a chemical system shape the behavior and outcomes of reactions. One particular advantage of physical chemistry is its powerful computational tools that allow researchers to model chemical behaviors in silico, paving the way for predictive chemistry. However, an ongoing challenge is the accurate simulation of large and complex molecules, where computational limits can hinder progress.
Quantum Chemistry
Quantum chemistry is an attractive field that investigates the quantum mechanical properties of atoms and molecules. This aspect is pivotal for accurately predicting chemical reactions and understanding molecular interactions. Its popularity stems from its potential to model complex systems that classical mechanics cannot adequately explain. A unique feature of quantum chemistry is the application of quantum mechanics to explain observable chemical phenomena, offering a more profound insight into molecular structures and dynamics. The downside can be the high level of abstraction and mathematical complexity, which can be daunting for some practitioners.
Chemical Physics
Lastly, chemical physics examines the physical principles underlying chemical systems and processes, focusing on phenomena like molecular dynamics and electronic structures. What stands out about chemical physics is its emphasis on integrating concepts from both chemistry and physics, creating a multidisciplinary framework. This dual focus allows researchers to approach problems from various angles, which is highly beneficial for innovation. However, a downside is that it requires a strong grasp of both fields, which may pose a barrier for those specialized in only one discipline.
Interdisciplinary approaches in physics not only broaden understanding but also encourage collaboration, leading to innovative solutions that address real-world problems.
Technological Innovations
The field of physics is experiencing a remarkable transformation as technological innovations continuously push the boundaries of what is possible. These advancements have not only changed the landscape of research but also the way physicists approach longstanding questions. Technologies such as measurement techniques and computational methods hold key importance, reflecting the intricate dance between theory and experimentation. Embracing these innovations enables researchers to gain deeper insights and inform the next wave of scientific discoveries. The significance of technological innovations in physics cannot be overstated, as they lay the groundwork for future explorations and interdisciplinary collaborations.
Developments in Measurement Techniques
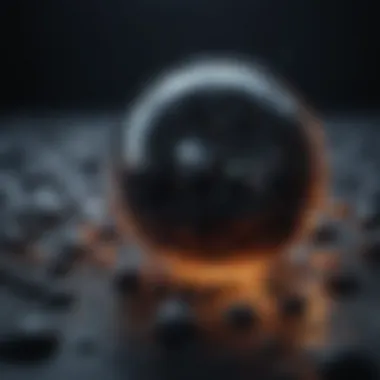
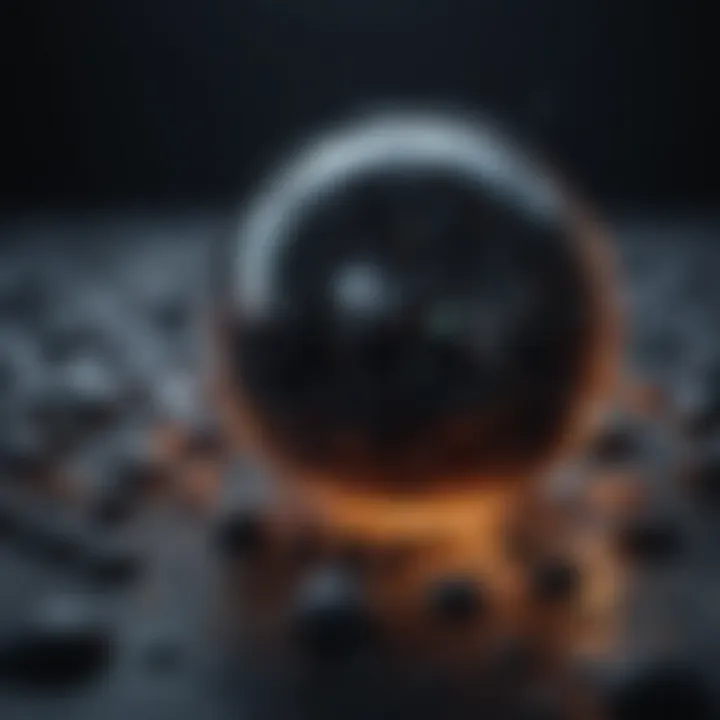
High-Energy Particle Colliders
High-energy particle colliders are pivotal in the quest to understand the fundamental particles that constitute our universe. These colossal machines, like the Large Hadron Collider (LHC), smash protons together at near-light speeds, generating conditions similar to those just after the Big Bang.
The unique aspect of high-energy particle colliders lies in their ability to detect rare events and new particles, significantly contributing to the Standard Model of particle physics and our understanding of forces at play. They remain a beneficial choice for this article because they illustrate the fusion of cutting-edge technology with theoretical frameworks, specifically when searching for elusive particles such as the Higgs boson.
Advantages include the ability to test predictions made by theoretical physicists, while disadvantages range from the immense costs of building and operating these facilities and the complex data analysis they necessitate.
Advanced Imaging Methods
Advanced imaging techniques are another cornerstone of contemporary physics research. These methods, such as electron microscopy and nuclear magnetic resonance (NMR), allow researchers to visualize materials at unprecedented resolutions. The key characteristic of these methods is their capacity to probe the structure of atoms and molecules, unveiling intricacies that were once hidden.
These imaging techniques are crucial for a variety of applications, such as materials science and biological research. They serve as a beneficial inclusion in this article, highlighting how advancements in imaging can lead to significant breakthroughs in understanding complex systems.
A unique feature of advanced imaging methods is their potential to facilitate real-time observations in experimental conditions, though they can be limited by resolution and the physical limitations of the systems involved.
Precision Spectroscopy
Precision spectroscopy is an essential technique that measures the interaction of light with matter to gain insights into atomic and molecular structures. By using highly sensitive instruments, physicists can detect minuscule energy shifts that reveal varying properties of substances. This specificity makes precision spectroscopy invaluable in fields like photonics and quantum mechanics.
What sets precision spectroscopy apart is its ability to test fundamental constants and search for variations, making it an important point of discussion in this article. The clarity it provides also opens the door for new discoveries, while its complexity and need for advanced technology can pose challenges.
Computational Approaches
Simulation Techniques
Simulation techniques have revolutionized the landscape of physics research by allowing scientists to model complex physical systems. By utilizing high-performance computing, researchers can create simulations that tackle dynamic systems that are difficult or impossible to replicate in the lab. This capability has become a valuable tool for testing theories, understanding phenomena, and predicting outcomes.
The standout feature of these techniques is their versatility in applications ranging from cosmology to condensed matter physics. They are a popular choice for this article, as they exemplify how theoretical predictions can be validated through computational means. However, models may be constrained by assumptions or simplifications that can limit their applicability, necessitating a careful approach to interpretation.
Data Analysis in Physics
As the quantity of data generated from experiments and simulations continues to expand, effective data analysis becomes paramount in physics research. The emphasis on statistical methods and machine learning algorithms helps researchers sift through vast datasets, extracting meaningful patterns and insights. This aspect of data analysis is critical in drawing valid conclusions from experimental results.
Highlighting data analysis in physics carries benefits, such as enhancing accuracy and improving the efficiency of research efforts. However, the challenges lie in ensuring that the algorithms and models used are appropriate for the data, as errors can lead to misinterpretations.
Machine Learning Applications
Machine learning has emerged as a game-changer within physics, offering sophisticated methods to analyze data, recognize patterns, and even make predictions. With the capacity to adapt and learn from data inputs, machine learning applications allow researchers to tackle complex problems more effectively, often with greater speed than traditional analytical methods.
The key characteristic that makes machine learning a compelling topic for this article is its ability to operate at scales that exceed human capabilities. However, its successful application requires substantial training data and raises concerns about interpretability and the potential for biases within algorithms.
"At the heart of innovation in physics is the interplay between technology and theory—where one informs and enhances the other, carving paths towards discovery." \n
Challenges and Future Directions
In the ever-evolving landscape of physics research, recognizing and addressing the challenges that lie ahead is not just prudent; it’s essential. The complexities of modern physics require a thoughtful integration of theoretical principles and experimental validation. Researchers face the monumental task of not merely pushing the boundaries of knowledge but also fine-tuning methods to translate theory into observable phenomena.
Integrating Theory and Experimentation
One of the key elements of progress in physics research is the synthesis of theory and experimentation. For instance, when researchers propose a new theoretical model, the next question often is: how can this be tested? The bridging of this gap is crucial because theories without experimental backing can lead to stalemates in scientific development.
However, challenges abound. Experimental setups may be limited by technology, resources, or even imprecise theoretical predictions. This disjunction can impede advancements, often leaving the scientific community to speculate the veracity of a theory without the concrete legs of data to stand on. An example would be attempts to detect gravitational waves. Theories around them were formidable, but it was only through collaborations leading up to monumental projects like LIGO that the ideas transformed into published results, reshaping our understanding of the universe. This imperative relationship lays the groundwork for future discoveries.
Ethical Considerations in Research
As physics pushes forward, so too must the ethical frameworks guiding research. This conversation is more than just compliance with guidelines; it’s about the moral responsibilities inherent in scientific inquiry. Physics research, particularly in areas touching on human health, environmental implications, or societal effects, often demands careful scrutiny. Researchers must ask: What are the short-term and long-term consequences of their findings? Are there unintentional harms that could arise from technological advancements?
Locating this discussion within the global context is important. For instance, the implications of nuclear physics extend well beyond the laboratory. With technologies developed in this area, nuclear energy, while a promising tool in combating climate change, also raises questions of safety and waste management. Therefore, a proactive stance on ethical dilemmas ensures that future advancements contribute positively to society rather than diminish public trust in science.
The Role of Education in Advancing Physics Research
Education carries the torch that illuminates future directions in physics. As new questions arise, trained individuals equipped with the right skills will be vital in navigating the complexities of modern physics. Critical thinking, problem-solving, and the ability to grasp advanced concepts are more than academic endeavors; they are necessary qualities that researchers must embody.
Institutions are increasingly aware of the need to enhance curricula that bridge theoretical knowledge with practical applications. Programs that involve hands-on experience in research projects can prepare students not just to enter the field but to innovate within it.
Moreover, interdisciplinary approaches in education can provide a broader perspective. For example, integration of computational sciences or even ethical coursework can equip budding physicists with tools they need to comprehend the broader implications of their research.