Bacteria's Essential Role in Genetic Engineering
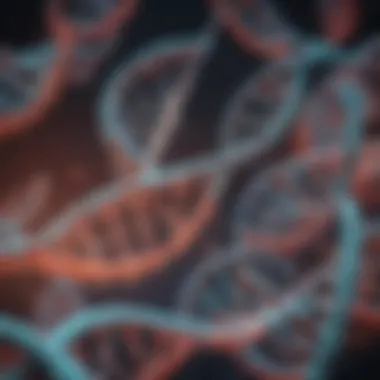
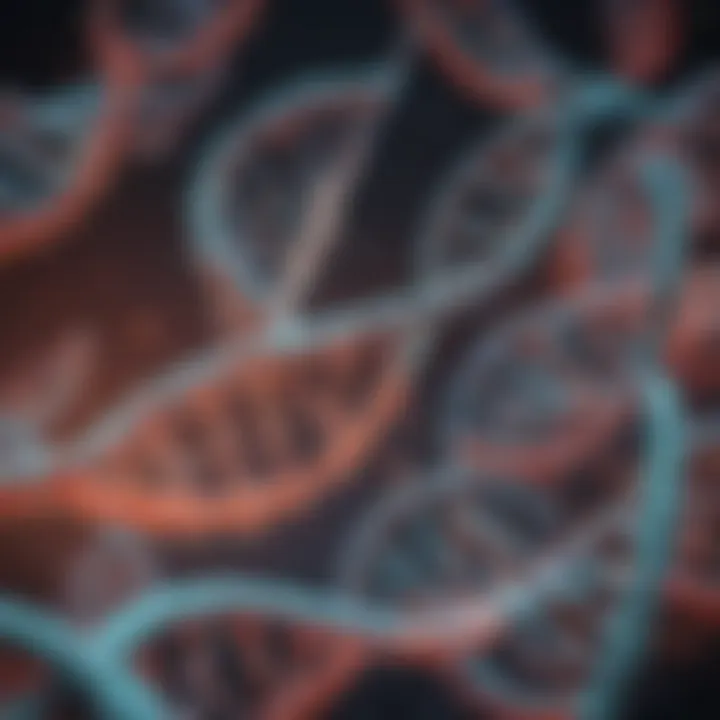
Intro
The role of bacteria in genetic engineering is a story of small organisms doing big things. Bacteria, which are often thought of as mere pathogens or environmental nuisances, hide a treasure trove of genetic potential. Over the decades, researchers have harnessed their capabilities for various groundbreaking applications, fundamentally altering the landscape of biotechnology. These microscopic entities are not just building blocks; they serve as vital cogs in a larger machinery that allows scientists to manipulate life at its core.
This comprehensive exploration draws back the curtain on how bacteria contribute to genetic engineering, shedding light on the mechanisms of gene manipulation, ethical dilemmas that arise, and the potential impacts on society. With advancements in areas such as synthetic biology and gene editing, it becomes evident that our understanding and control over these organisms have profound implications, setting the stage for an era filled with both promise and challenge.
Research Overview
Understanding the pivotal role of bacteria in genetic engineering begins with a thorough review of the research landscape. A summation of key findings reveals not only how these microorganisms function but also why they have become indispensable tools in this field.
Summary of Key Findings
Bacteria have been instrumental in various applications:
- Gene Editing: They serve as vectors for delivering genetic material to other organisms, allowing for precise alterations in DNA within a host.
- Synthetic Biology: Through the combination of biological parts, bacteria can be engineered to produce valuable compounds such as biofuels and pharmaceuticals.
- Therapeutic Development: Many treatments for diseases, including cancer, utilize bacteria to deliver drugs directly to their targets, enhancing efficacy and reducing side effects.
Methodologies Employed
Several methodologies enable scientists to harness the power of bacteria:
- CRISPR-Cas9: A revolutionary technology allowing for targeted gene editing through bacterial immune systems.
- Plasmid Transformation: Techniques that utilize plasmids to introduce foreign genes into bacterial cells, thus creating modified strains for research or therapeutic applications.
- Genome Sequencing: Advances in sequencing technology allow for precise mapping of bacterial genomes, revealing pathways for engineered modifications.
In-Depth Analysis
A deeper dive into these methodologies illuminates our findings and showcases the intricacies involved in manipulating bacterial genomes.
Detailed Examination of Results
Research illustrates a range of impressive outcomes resulting from bacterial applications:
- Strains of E. coli have been modified to synthesize useful drugs and even biodegradable plastics.
- Innovations in gene therapy demonstrate how engineered bacteria can effectively produce proteins to combat genetic disorders.
Comparison with Previous Studies
Historically, the advancements in bacterial genetics have spurred significant breakthroughs:
- Earlier studies primarily focused on natural variations, while modern techniques allow for more deliberate modifications that were previously deemed unattainable.
- Notably, the introduction of CRISPR technology demonstrated capabilities once imagined only in the realm of science fiction.
This thorough exploration offers a panoramic view of bacteria's key role in genetic engineering, reflecting both their historic accomplishments and future potential.
Prelude to Genetic Engineering
Genetic engineering stands as a cornerstone of modern biotechnology. By manipulating the genetic material of organisms, scientists unlock new possibilities that can impact agriculture, medicine, and environmental science. The importance of this field cannot be overstated; it lays the groundwork for innovations that can potentially solve some of humanity's most pressing challenges.
One of the key elements in this area is the ability to edit genes with precision. This precision not only enhances the efficiency of experimental processes but also addresses issues such as food security, disease prevention, and the management of natural resources. For instance, crops that are genetically modified for resilience against pests often produce higher yields and require fewer chemical inputs, ultimately benefiting both farmers and consumers.
Moreover, genetic engineering facilitates personalized medicine; through genetic therapies tailored to individual genetic makeups, patients can see improved treatment outcomes. Here we see the intersection of ethics and innovation, as the profound implications of manipulating life at the genetic level raise important societal considerations.
The engagement with genetic engineering requires careful thought, particularly around the safety, ethical ramifications, and long-term consequences of genetic modifications. This article will explore the pivotal role of bacteria in supporting genetic engineering, shedding light on the diverse applications and the complexities involved in this transformative science.
Defining Genetic Engineering
Genetic engineering, in its simplest form, refers to the process of altering the genetic material of an organism. This alteration could involve adding a new gene, removing an existing one, or modifying a gene's expression. The goal is often to confer desirable traits or to gain a better understanding of specific biological processes.
It encompasses a range of techniques, including but not limited to:
- Recombinant DNA technology: This involves combining DNA from different organisms to create new genetic combinations.
- Gene editing tools: Techniques such as CRISPR-Cas9 allow for precise modifications at specific locations in the genome.
- Gene cloning: This is the process of making multiple copies of a gene or a piece of DNA.
These methods enable advancements in various fields – agriculture, medicine, and basic biological research. The growing importance of genetic engineering in society sets the stage for vast improvements in how we approach health care, food production, and environmental sustainability.
Historical Context and Evolution
The historical roots of genetic engineering can be traced back to early agricultural practices, where selective breeding laid the foundation for manipulating living organisms. However, it wasn't until the mid-20th century that the modern techniques we recognize today began to take shape.
In 1953, James Watson and Francis Crick described the double helix structure of DNA, igniting widespread interest in molecular biology. Fast-forward to the 1970s, and scientists were developing the tools of recombinant DNA technology, allowing for the first time the splicing of DNA from different sources.
- 1973: The first successful insertion of a foreign DNA segment into a bacterium, marking the dawn of genetic engineering.
- 1982: Insulin produced through genetic engineering became the first genetically modified drug available to the public.
- 1994: Flavr Savr tomato, the first genetically modified food product, hit the market, showcasing the commercial applications of this technology.
These milestones highlight how genetic engineering evolved from theoretical understanding to practical applications. As research continues to advance, the integration of bacteria in genetic engineering becomes even more essential. Bacteria not only serve as versatile tools for gene manipulation but also play a pivotal role in producing desirable products, from vaccines to biofuels.
Understanding the historical backdrop enhances our grasp of the current landscape of genetic engineering and prepares us for its future developments.
The Role of Bacteria in Biotechnology
Bacteria are often painted as the villains in the microbiological world, but in the realm of biotechnology, they transform into essential allies. Their role is immense and multifaceted, touching on various aspects from genetic manipulation to bioproduction. Using these microscopic powerhouses, scientists have been able to design, develop, and optimize technologies that can solve pressing health and environmental problems.
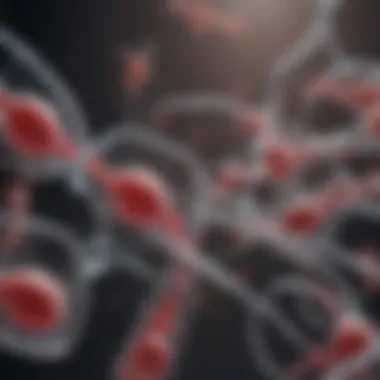
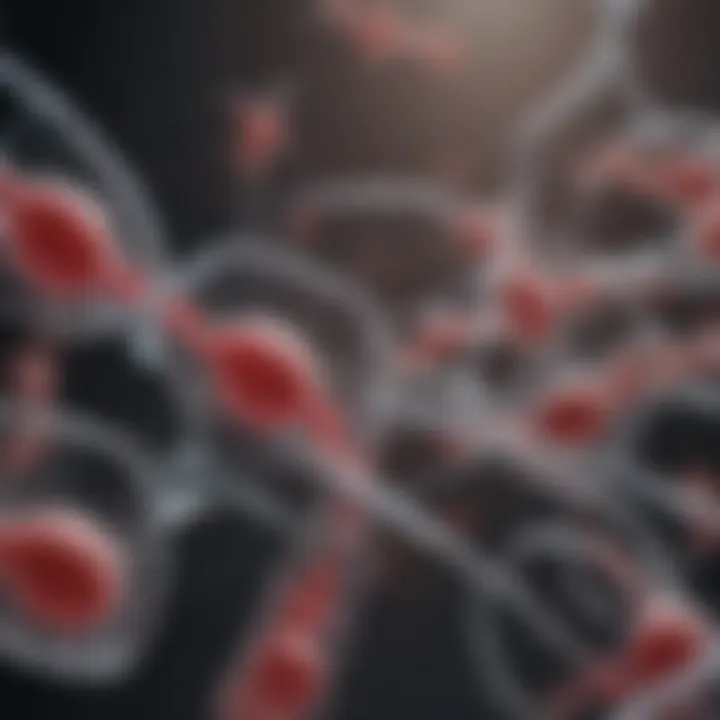
The heart of biotechnology is the conversion of biological organisms into practical applications. And here, bacteria are not just players; they are the stars of the show. With their remarkable adaptability and metabolic versatility, they serve as ideal platforms for a myriad of biotechnological processes.
Bacterial Systems as Biotechnological Tools
Bacterial systems have become the backbone of numerous biotechnological applications. One of the primary reasons for their prominence is their ability to undergo rapid genetic transformation through techniques such as transformation, transduction, and conjugation. Scientists harness these processes to insert or modify genes, allowing for the creation of genetically modified organisms that can produce insulin, vaccines, and other important biological products.
Notably, certain strains like Escherichia coli and Bacillus subtilis are widely used due to their well-characterized genetics and ease of manipulation. These bacterial systems have become essential not only in research laboratories but also in industrial applications, operating on a large scale to produce everything from pharmaceuticals to enzymes that amplify various biotechnological processes.
Advantages of Bacterial Models
Rapid Growth Rates
One standout aspect of bacterial models is their rapid growth rates. Bacteria can double in number every 20 minutes under optimal conditions. This feature significantly contributes to the efficiency of genetic engineering. When researchers manipulate bacteria in laboratory settings, they can quickly gauge the outcomes of their genetic modifications. For example, in developing strains for industrial use, faster growth means quicker production cycles, allowing for faster returns on investments.
Another key characteristic of this rapid growth is the ability to produce a high biomass in a short time. This characteristic is beneficial for both research and practical applications—yielding more products and results in less time.
Unique feature: While fast growth is advantageous, it may present some challenges. For instance, it can lead to increased mutations if conditions aren’t precisely controlled, which is something researchers must constantly manage.
Plasmid Intro
Plasmid introduction is fundamental in the genetic engineering toolkit. These small, circular pieces of DNA can be easily manipulated and inserted into bacterial cells, allowing scientists to introduce new genes. This method is crucial because it enables the amplification of genes of interest and their study in a bacterial context.
The simplicity of using plasmids is one reason they have gained popularity. They can carry antibiotic resistance genes, which help select successfully modified bacteria from a mixture. Moreover, since plasmids replicate independently, they ensure that the gene of interest is amplified alongside the bacterial cell’s growth.
Unique feature: Though plasmid systems are user-friendly, there's the potential drawback of instability. Plasmids can be lost during cell division if not selected for rigorously, which can result in the loss of desired traits in the engineered bacteria.
Simplicity of Culturing
The simplicity of culturing bacteria plays an equally vital role in their suitability for genetic engineering. Basic nutrient media can support bacteria's growth, making it feasible to culture them in a variety of environments without extensive resources. This means both academic labs and large-scale industries can adopt bacterial systems without investing in expensive equipment.
Additionally, controlled growth conditions allow researchers to isolate certain variables and focus on specific genetic alterations without worrying too much about external factors. This predictability is an invaluable aspect when it comes to constructing experiments in genetic research.
However, while culturing is straightforward, scaling it up for industrial production can lead to complications. Contamination or accidents in large fermentors could result in significant losses.
"Bacteria are not just life forms; they are the very building blocks of modern biotechnology, pivotal in turning theoretical research into practical realities."
Through the lens of these advantages, the role of bacteria in biotechnology becomes increasingly clear. Their rapid growth, plasmid transformation capabilities, and ease of culturing elevate them above other organisms in the toolkit of genetic engineering. Understanding these elements is crucial for anyone diving into the world of genetic manipulation and its applications.
Mechanisms of Genetic Manipulation in Bacteria
The mechanisms through which bacteria are manipulated genetically are foundational to the advancement of biotechnology. Understanding these processes allows researchers to harness bacterial capabilities for various applications, from medicine to agriculture. Grasping how genetic manipulation occurs not only paves the way for innovation but also highlights the intricate dance of biological cooperation and competition at the micro level.
Transformation Processes
Transformation is one of the primary pathways for introducing foreign DNA into bacteria. In this process, a bacterium takes up naked DNA from its surrounding environment, a phenomenon that can occur naturally or be induced in the lab. It is significant for several reasons:
- Natural Competence: While some bacteria like Streptococcus pneumoniae have evolved the ability to uptake DNA naturally, others may require artificial means to enhance their competence. This variability makes transformation a versatile tool, showing that not all bacteria play by the same rules.
- Genetic Diversity: Transformation enhances genetic diversity within bacterial populations, allowing them to adapt to changing environments more easily. By integrating new genes, bacteria can develop new traits, which might confer advantages like antibiotic resistance.
- Application in Research: In a laboratory setting, scientists can induce transformation using techniques like electroporation, which applies an electric field that makes the bacterial membrane permeable. This method facilitates the introduction of plasmids carrying target genes, crucial for developing genetically modified organisms.
Transduction Techniques
Transduction, another intriguing mechanism of genetic manipulation, involves the transfer of DNA from one bacterium to another via a bacteriophage, or bacterial virus. This process, while perhaps less direct than transformation, carries unique benefits:
- Efficiency: Transduction is often more efficient than transformation, especially for species that are not naturally competent. Bacteriophages can carry genetic material across species barriers, introducing genes that might never be exchanged through conventional means.
- Specificity: This method can be incredibly specific; certain bacteriophages will only infect particular bacterial species, which helps researchers narrow down their targets in genetic studies. It also sheds light on evolutionary relationships and gene transfer dynamics within environments.
"Transduction shows how viruses can serve as tools for genetic advancement, bridging gaps that natural methods cannot."
- Gene Packaging: The ability for bacteriophages to package and deliver large segments of DNA makes transduction particularly valuable for complex genetic manipulations, such as those needed for creating multi-gene knockouts.
Conjugation Mechanisms
Conjugation introduces a form of genetic transfer that is essentially a bacterial mating process. During conjugation, one bacterium transfers genetic material to another through direct contact. This mechanism stands out due to its profound implications for genetic engineering:
- Horizontal Gene Transfer: Conjugation enables bacteria to share genes rapidly and efficiently, promoting traits such as antibiotic resistance among populations. This horizontal gene transfer is critical for understanding microbial ecology and evolution, as it highlights how bacteria can swiftly adapt to challenges.
- Plasmid Exchange: The ability of plasmids to replicate independently of chromosomal DNA allows for versatile applications. For instance, scientists often use plasmids as vectors to introduce specific genes during experimental procedures.
- Directional Information: Conjugative transfer typically involves a donor and a recipient, which means that the transfer is directional. This characteristic can be useful in specific applications where manipulation requires transferring a gene along with a sequence of regulatory elements that modulate its expression.
As bacteria continue to be the cornerstone of genetic engineering initiatives, understanding these mechanisms becomes increasingly crucial. Each pathway—transformation, transduction, and conjugation—carries its unique significance, opening doors to diverse applications and enriching our comprehension of microbial genetics.
In combining these strategies, researchers can push the envelope of what is possible, crafting bacteria that serve myriad purposes, from bioremediation to production of pharmaceuticals.
Gene Editing in Bacteria
Gene editing in bacteria represents a cornerstone of modern biotechnology, paving the way for advancements across multiple sectors, from medicine to agriculture. Bacteria, with their simple structure and rapid reproduction, serve as effective models for genetic manipulation. Manipulating their genetic material not only enhances our understanding of genetic functions but also provides practical tools for addressing some pressing challenges faced by society today. One of the primary benefits of gene editing in bacteria is the potential for rapid prototyping of genetic sequences, allowing researchers to experiment and iterate at an unprecedented pace.
CRISPR-Cas Systems
Mechanism of Action
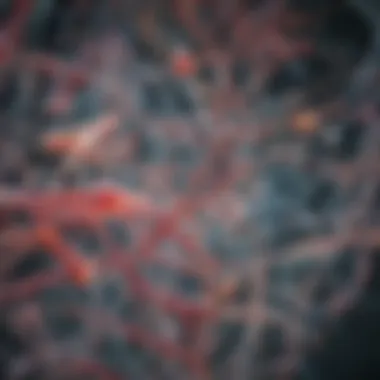
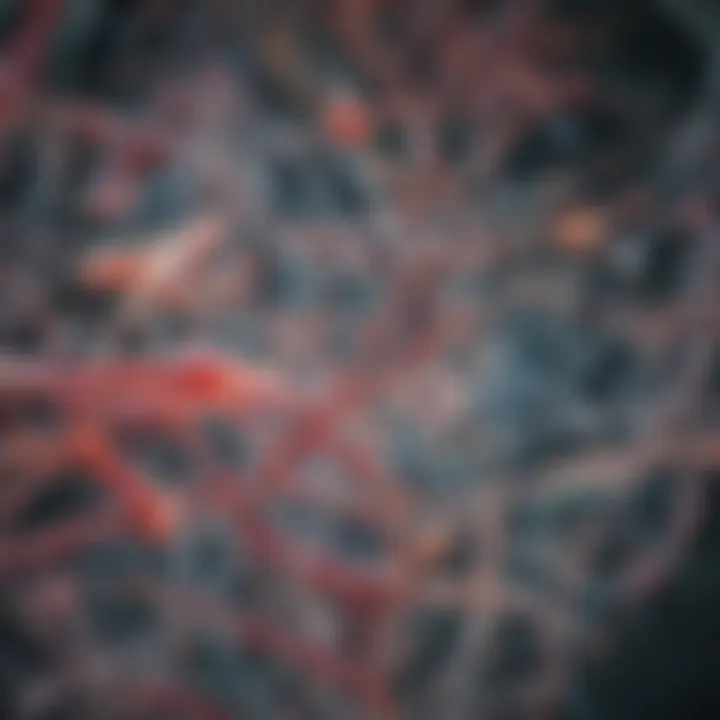
The CRISPR-Cas system is a groundbreaking tool for genetic engineering, characterized by its ability to target and edit specific DNA sequences within an organism's genome. This system operates like a pair of molecular scissors, allowing scientists to make precise changes to the genetic code. One remarkable aspect of the CRISPR mechanism is its function of using RNA as a guide to locate the target DNA sequence. This specificity minimizes off-target effects that are often a concern in genetic editing. The unique feature of CRISPR-Cas is its adaptability, as it can be programmed to target almost any gene, making it a flexible and powerful choice for gene editing in nearly any bacterial system.
Applications in Genetic Engineering
The applications of CRISPR-Cas systems in genetic engineering are vast. Primarily, they are employed for gene knockout, allowing researchers to disable specific genes to study their functions. Additionally, CRISPR enables the insertion of new genetic material, thereby providing a pathway for introducing beneficial traits into bacterial strains. This offers practical benefits in various fields such as agriculture, where genetically engineered bacteria can improve crop resilience or productivity. One of the key characteristics that set CRISPR apart from other gene editing technologies is its ease of design and implementation, making it favorable for both academic and industrial applications. However, the efficiency of CRISPR-Cas systems can vary, depending on the organism and conditions, posing challenges that researchers are actively working to understand.
Other Gene Editing Technologies
TALENs
Transcription Activator-Like Effector Nucleases (TALENs) are another important technology in the realm of gene editing. This method utilizes customized proteins to bind to specific DNA sequences, enabling the creation of targeted double-strand breaks. The key characteristic of TALENs lies in their modular design, which allows for the easy customization of binding domains, thus granting a high level of specificity. Nonetheless, while TALENs offer precision, the labor-intensive and time-consuming process of constructing these proteins can be a limitation. The trade-off between specificity and ease of use presents a continuous discussion among researchers considering their application.
Zinc-Finger Nucleases
Zinc-Finger Nucleases (ZFNs) are yet another. They combine DNA-binding zinc finger proteins with nuclease activity. ZFNs are particularly notable for their ability to achieve targeted gene modification and create DNA double-strand breaks. Their main advantage is the versatility that comes with programming different zinc fingers to target a plethora of DNA sequences. However, similar to TALENs, the complexity of designing ZFNs can stem from their requirement for extensive protein synthesis and evaluation, which impacts their application in routine bacterial genetic engineering.
In summary, both TALENs and ZFNs, along with CRISPR-Cas systems, illustrate the diverse toolkit available for genetic editing. Each brings unique advantages and challenges, but their contributions collectively push the field of genetic engineering forward, with bacteria serving as a crucial platform for these innovations.
Synthetic Biology and Bacteria
Synthetic biology has emerged as a transformative field that intricately connects to the capabilities of bacteria. This intersection paves the road for groundbreaking innovations, from biofuel production to healthcare solutions. Bacteria, with their natural ability to adapt and thrive in diverse environments, serve as the backbone for synthetic biological systems. By harnessing their unique properties, scientists can design new biological functions that address pressing challenges in various sectors.
One significant advantage of utilizing bacteria in synthetic biology is their rapid replication rate. For researchers, this translates to faster prototyping and testing of genetic circuits. With advancements in genetic engineering, it's now quite common to manipulate bacterial DNA to create organisms that can perform designated tasks.
Designing Biological Circuits
When it comes to designing biological circuits, bacteria become the ideal canvas. Just as electronic circuits comprise components like resistors and capacitors, biological circuits rely on genetic elements such as promoters, ribosome binding sites, and terminators. Through creative assembly of these components, scientists can engineer bacteria to respond to specific environmental cues or perform sequential biological processes.
- Basic Elements: Components might include gene regulatory elements and signalling proteins.
- Applications: These circuits can be programmed for bio-sensing toxins, producing pharmaceuticals, or even synthesizing novel compounds.
The iterative design-build-test-learn cycle is integral to this process, often leading researchers to unexpected discoveries and advancements. As researchers play with arrangements of genetic material, they not only innovate but also deepen their understanding of biological complexity.
Bacterial Factories for Biofuel Production
Another fascinating application of bacteria in synthetic biology is their role as biofuel production factories. Given the pressing need for sustainable energy sources, leveraging bacterial metabolism to produce biofuels like ethanol or biodiesel has enormous potential. Bacteria can be genetically modified to metabolize non-food biomass, converting agricultural waste into energy-rich compounds.
Benefits of using bacteria in this context include:
- Cost-Effectiveness: Utilizing inexpensive feedstock makes the biofuel production more economical.
- Scalability: Bacteria can be cultured in large volumes, enhancing production capabilities.
- Environmental Impact: This process can reduce carbon footprints by recycling waste.
"Harnessing bacteria for biofuel not only addresses energy demands but also contributes to environmental sustainability."
To conclude, the integration of synthetic biology and bacteria is ripe with possibilities. As researchers continue to innovate in designing biological circuits and creating bacterial factories, they stand on the brink of revolutionary solutions—offering hope for a sustainable future and redefining our relationship with biological systems.
Therapeutic Applications of Bacteria
The therapeutic potential of bacteria has become a vibrant focal point in the field of genetic engineering. This area is not merely about harnessing these microorganisms for industrial purposes; rather, it involves a deeper exploration into their usage as a cornerstone for improving human health. Bacteria can serve as living factories that produce essential molecules, gene therapies, or probiotics that improve overall health outcomes. The discussion around these therapeutic applications is paramount, given their burgeoning role in addressing chronic diseases, enhancing immune responses, and even combating infectious diseases.
Gene Therapy Utilization
Gene therapy represents one of the most promising avenues in medical treatment, and bacteria play a pivotal role here. By entering the cells and delivering genetic material directly, certain types of bacteria provide a novel mechanism to overcome genetic disorders. Specifically, engineered strains can potentially introduce corrective genes into targeted cells, healing various hereditary diseases. An example of this field is the use of Lactococcus lactis, which, when genetically modified, can produce therapeutic proteins that could address autoimmune diseases or certain cancers.
Additionally, using bacteria for gene therapy ensures a unique and targeted approach. Unlike traditional methods, bacterial delivery systems can be tailored to recognize specific cancer cells, ensuring that the therapeutic agent is directly administered where it is needed most. This precision minimizes damage to surrounding healthy tissues, illustrating the significance of bacterial systems in preventive medicine and treatment strategies. The potential is mind-boggling; as research evolves, it may redefine how we treat some of the most stubborn health issues we face today.
Probiotics as Therapeutic Agents
Not all therapeutic applications of bacteria are about fancy genetic modifications. Probiotics represent a simpler yet profound use of these microorganisms. These beneficial bacteria can significantly impact gut health, influence immune function, and even improve mental health outcomes. The consumption of probiotics, found in products like Yogurt or Kefir, can restore the natural balance of bacteria in the gut, particularly after antibiotic use, which can wipe out beneficial bacteria along with harmful ones.
Research suggests these probiotics can help stave off gastrointestinal issues such as irritable bowel syndrome or antibiotic-associated diarrhea. Additionally, emerging studies are investigating how these microorganisms can impact mood and anxiety levels, suggesting a gut-brain connection that is becoming more recognized in the medical community.
"The exploration of probiotics underscores how therapeutic bacteria are not only a promising avenue for treatment but also a fundamental part of our microbial ecosystem."
In summary, the therapeutic applications of bacteria encompass a wide range of innovative and traditional uses. From sophisticated gene therapy systems that target genetic disorders to the everyday benefits of probiotics improving gut health, the manifold potential of bacteria in medicine is a testament to their invaluable role in genetic engineering. As scientists keep unraveling these possibilities, the future of bacterial applications looks bright, poised to lead to groundbreaking advancements in health care.
Ethical and Societal Considerations
The subject of ethical and societal considerations in the realm of genetic engineering, especially concerning the use of bacteria, is of paramount importance. As advances in biotechnology and genetic manipulation rattle the foundations of traditional practices, a responsible approach is required to navigate the myriad implications that emerge. Understanding ethical implications isn't just an academic exercise; it resonates deeply within public discourse and policy-making. This section dissects two key facets: bioterrorism risks and biosecurity, alongside public perception of genetic engineering.
Bioterrorism Risks and Biosecurity
In an era where information travels at the speed of light, the bioterrorism risks tied to bacterial genetic engineering loom large. The potential for manipulating bacteria — whether for malicious or benign goals — raises eyebrows among security experts and policymakers alike. There exists a fine line between harnessing bacterial capabilities for beneficial applications and opening the Pandora's box of bioengineering misuse.
- Pathogen Development: Altering pathogens to enhance their virulence or resistance can transform a simple lab concept into a catastrophic public health risk. For instance, researchers could theoretically enhance the spread of Yersinia pestis, the bacterium responsible for the plague. This situation emphasizes the need for robust oversight in biotechnological endeavors.
- Biosecurity Protocols: To safeguard against these threats, biosecurity measures are crucial. Laboratories engaged in genetic engineering must adhere to stringent guidelines to prevent unauthorized access and misuse of engineered organisms. Protective strategies should include:
- Secure Lab Environments: Implementing access controls and surveillance mechanisms.
- Research Oversight: Establishing ethical review boards to oversee projects and ensure compliance with ethical standards.
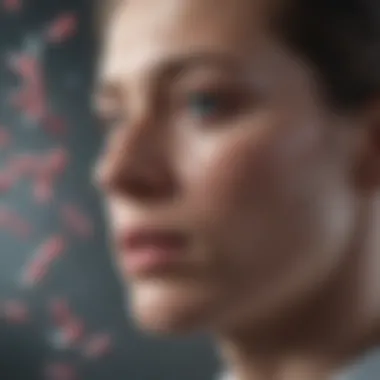
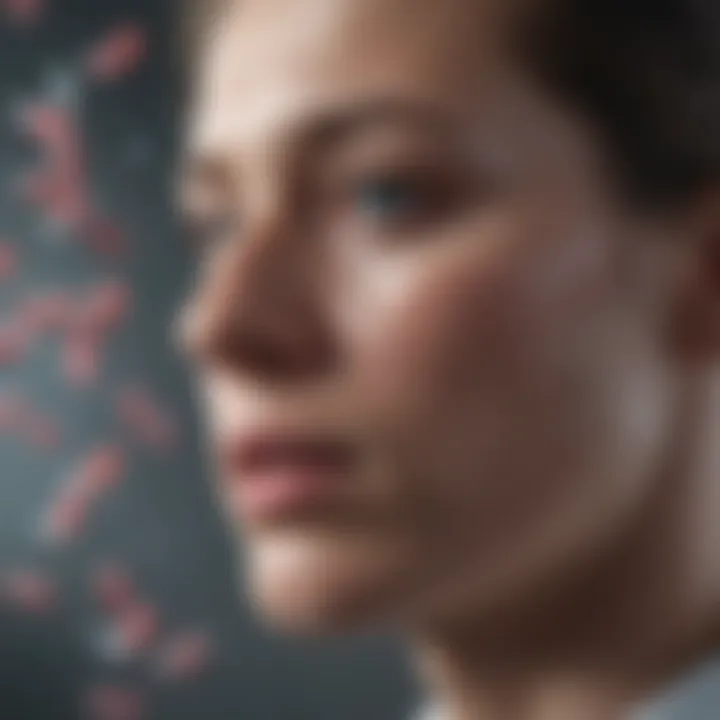
As we push into uncharted territories with genetic manipulation, creating a culture of safety and accountability in research communities can serve as a safeguard against bioterrorist threats, ensuring public trust.
Public Perception of Genetic Engineering
How society views genetic engineering, particularly when it involves bacteria, significantly influences its acceptance and regulation. An informed public can guide policy frameworks, while a misinformed one can lead to fear and resistance.
- Knowledge Gaps: Often, the average person has little understanding of the nuanced methods and benefits of genetic engineering. For instance, some might equate genetic modifications to ‘playing God,’ raising ethical eyebrows. This results in a failure to recognize the life-saving potential that genetically engineered organisms hold, such as bacteria engineered to produce insulin or clean up environmental pollutants.
- Media Influence: The portrayal of genetic engineering in the media can skew perceptions. The dramatization of laboratory mishaps or the sensationalistic reporting of biohazard threats often overshadows positive stories and advancements. People may latch onto fear instead of progress, underestimating the thorough assessments these technologies undergo before implementation.
Encouraging informed discussions is essential to bridging the chasm between scientific communities and the public. Initiatives like community-based educational programs can demystify the reality of genetic engineering. Engaging with grassroots organizations, academic institutions, and even social media platforms can pave a path toward transparency and build a society attuned to the complexities of genetic engineering.
"In the age of biotechnology, knowledge is not just power; it’s a necessity for safety."
As we move forward, the key lies in facilitating an open dialogue surrounding the ethical considerations in bacterial genetic engineering. An informed society can help steer the future course of biotechnology responsibly, ensuring that the potential benefits are realized without compromising safety or ethical integrity.
Challenges in Bacterial Genetic Engineering
Genetic engineering utilizing bacteria brings forth a multitude of opportunities, yet it does not come without its fair share of challenges. These obstacles often dictate the pace and extent to which researchers can innovate and apply techniques important for breakthroughs in biotechnology. The ability to genuinely harness the power of bacterial genetic manipulation hinges on addressing these challenges, which can both impede and enrich the field.
Technical Limitations
One might think that the sheer adaptability of bacteria would lend itself to a miracle cure for all our genetic engineering woes, but the reality is more nuanced. While bacteria like Escherichia coli are widely used in laboratory settings due to their rapid reproduction and genetic flexibility, there are inherent limitations that can stymie progress:
- Foreign DNA Integration: The process through which bacteria accept new genetic material is not always seamless. This can lead to issues such as incomplete integration or failure to express the new gene adequately, causing frustration for researchers.
- Genetic Drift: Over generations, bacteria can lose inserted genes through mutations or deletions. This phenomenon can compromise the stability of engineered strains, making long-term studies challenging.
- Metabolic Constraints: Bacteria have specific metabolic pathways, and not all foreign genes may function within these systems effectively. For example, genes from eukaryotic organisms might require different co-factors or conditions that bacteria simply cannot provide.
"In genetic engineering of bacteria, the fact is, the complexity of their innate systems might sometimes display a brain of their own."
Overall, these technical limitations necessitate ongoing research, innovation, and experimentation to circumvent the strategies that work well in microbial systems.
Regulatory Hurdles
The interplay between science and regulations often feels like navigating a minefield. When it comes to bacterial genetic engineering, regulatory hurdles can be particularly complex. There are various factors at play:
- Compliance with Guidelines: Researchers must adhere to various guidelines set forth by government and international bodies, which often govern everything from the specifics of laboratory procedures to geographical considerations for the deployment of modified organisms.
- Public Concerns: The general public may harbor fears about genetically modified organisms, leading to a heightened demand for stricter regulations. Issues like bioterrorism and environmental impact can complicate the approval process for new organisms created through genetic engineering, creating unnecessary delays.
- Intellectual Property Rights: Navigating the murky waters of patents and intellectual property can stifle innovation. Complications can arise when the creator seeks to monetize their inventions, making it essential to understand the regulations that govern ownership.
The balancing act between fostering innovation and safeguarding public interest remains a complicated matter. Addressing these regulatory hurdles will be essential so that bacterial genetic engineering does not languish in red tape, effectively hindering its potential contributions to society.
Overall, both technical limitations and regulatory hurdles stand as significant challenges within the field of bacterial genetic engineering. Understanding and addressing these factors may pave the way for future advancements and applications that could change the genetic engineering landscape.
Future Perspectives in Genetic Engineering
The landscape of genetic engineering continues to evolve at a breathtaking pace, driven largely by advancements in microbial genetics. Bacteria serve as the unsung heroes in this realm, functioning not only as tools but also as models that push the envelope of what's possible in biotechnology. Looking forward, the future perspectives in genetic engineering seem promising yet complex. This section will delve into emerging technologies and potential breakthroughs while emphasizing their significance and implications.
Emerging Technologies
Technological innovations frequently reshape the genetic engineering field. One such breakthrough is synthetic biology, where scientists engineer bacteria to create novel bioproducts showcasing synthetic capabilities. These “living factories” can produce not just basic proteins but also intricate compounds like pharmaceuticals and biofuels. In particular, two noteworthy emerging technologies stand out:
- CRISPR advancements: The CRISPR-Cas systems are continually refined to enhance precision and reduce off-target effects. Researchers are developing new tools that can fine-tune the process of gene editing, making it even more applicable across different bacterial types.
- Microbial consortia: Instead of working with a single strain, scientists are beginning to construct cooperative communities of bacteria. These consortia can tackle complex biological tasks, such as breaking down environmental pollutants or producing biofertilizers. By leveraging the strengths of various bacterial types, efficiency and resilience in biotechnological applications increase.
These technologies illuminate not only the potential for new applications but also highlight the need for assessments of safety and efficacy before these methods become commonplace.
Potential Breakthroughs
When one thinks of the future, breakthroughs tend to drift into the realm of the extraordinary. One can speculate on the life-changing impacts that might arise from genetic engineering involving bacteria:
- Precision medicine: Bacteria could be engineered to act as diagnostic tools, specifically targeting diseases at their source. They could circulate in the human body and report back on the presence of pathogens or biomarkers, enabling tailored therapeutic strategies.
- Environmental solutions: As climate change continues to threaten ecosystems, engineered bacteria could be instrumental in carbon capture and pollutant degradation. Imagine bacteria that can thrive in hostile environments while concurrently breaking down toxic waste, serving as an eco-friendly solution to pressing environmental challenges.
- Food security: In a world where population growth steadily rises, genetically modified bacteria could enhance crop yields by increasing nutrient availability or pest resistance. Furthermore, engineered strains might even produce cleaner, more sustainable food products, addressing the dual challenges of food scarcity and dietary health.
"The advancements in bacterial genetic engineering don't merely reflect scientific achievements; they carry the potential for societal transformation."
In summary, the trajectory of bacteria in genetic engineering is less about minor adjustments and more about redefining possibilities. Understanding these emerging technologies and the potential breakthroughs positions researchers and professionals at the forefront of a revolution that merges microbiology with the realms of health, environment, and agriculture. As we ponder these advances, we must also consider the ethical ramifications that inevitably accompany such shifts in capability.
Closure
The conclusion serves as a vital culmination of the varied themes discussed throughout the article. It encapsulates how bacteria have evolved into keystones in genetic engineering and biotechnology. By weaving together intricate threads of scientific discovery, ethical considerations, and real-world applications, a clearer picture emerges. It becomes apparent that understanding the dynamics of bacterial utility in genetic modification is crucial for fostering innovations that can lead to significant societal benefits.
In essence, the exploration of bacteria in genetic engineering isn't merely an academic endeavor; it has profound implications for medicine, agriculture, and environmental sustainability. For instance, imagine a world where bacterial systems are optimized to produce necessary pharmaceuticals at a fraction of the cost. Think about how synthesizing biofuels through engineered bacteria could drastically reduce carbon footprints. Each insight gained contributes to a broader understanding of how these microscopic entities can be harnessed for the betterment of human life and the planet itself, making it undeniably important to grasp their role in this intricate orchestration of life.
"The future belongs to those who believe in the beauty of their dreams." - Eleanor Roosevelt
Summary of Insights
Throughout the article, key insights emerge that underline the intricate relationship between bacteria and genetic engineering. Firstly, it's vital to appreciate the mechanisms through which bacteria are manipulated for research and applications. For example, CRISPR-Cas systems and other gene-editing tools like TALENs have been pivotal in advancing genetic engineering. The growth rates and simplicity in culturing bacteria allow for rapid experimentation, providing researchers with an invaluable asset.
Secondly, the therapeutic roles played by engineered bacteria cannot be overstated, from gene therapy that aims to cure genetic diseases to probiotics that support wellness. Each application highlights bacteria’s dual role as both subject and tool in the complex field of genetic engineering. Furthermore, as we navigate through ethical concerns and societal implications, it becomes clear that responsibility must be at the forefront of all advances made in this domain.
Call for Responsible Research
In light of the potential benefits and challenges presented in manipulating bacterial genomes, a strong call for responsible research is warranted. It is essential that scientists and institutions prioritize ethical guidelines as these revolutionary technologies evolve. With the capability to change lives, comes the responsibility to exercise caution and foresight.
This includes fostering transparency in research methodologies and outcomes, engaging with diverse communities to understand public sentiments, and addressing the potential risks associated with bioterrorism and biosecurity. A collaborative approach, integrating voices from various fields—social sciences, ethics, and community engagement—will provide a more holistic understanding of the implications of genetic engineering.
Education plays a crucial role here. Equipping current and future scientists, as well as the public, with knowledge about genetic engineering's promises and pitfalls can cultivate informed discussions around its societal impact. In this way, responsible research not only enhances technological advancements but also ensures that they align with the ethical standards that nurture trust in science.