The Essential Role of Wafer Substrates in Electronics
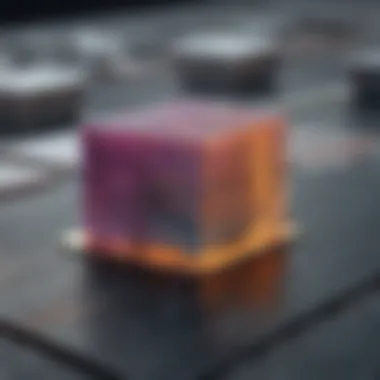
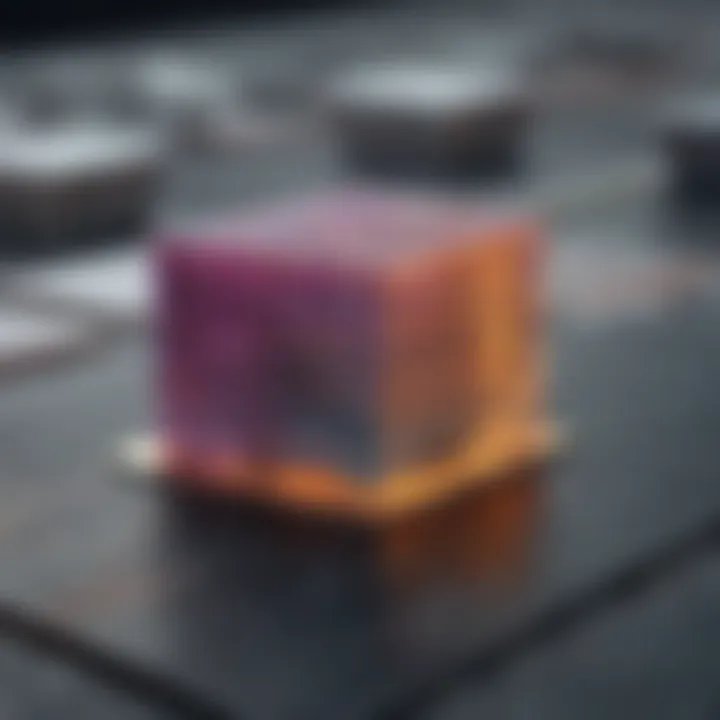
Intro
In the ever-evolving field of electronics, the significance of wafer substrates cannot be overstated. These thin slices of semiconductor materials serve as the foundational building blocks for a vast array of electronic devices. From smartphones to high-performance computing systems, wafer substrates play a crucial role in determining the efficiency, reliability, and longevity of devices. As the demand for advanced electronic capabilities continues to surge, understanding the properties, manufacturing processes, and advancements in wafer substrate technology becomes essential for students, researchers, educators, and professionals alike.
This article embarks on a detailed exploration of wafer substrates, with the aim of providing clarity around their applications and importance in modern electronics. Through a structured examination, this discourse will highlight the key components that characterize various types of wafer substrates and the unique advantages they offer in semiconductor fabrication. A focus will also be placed on emerging trends and technologies that seek to enhance electronic device performance through innovative substrate solutions. The ultimate goal is to equip readers with a comprehensive understanding of how substrate selection influences electronic functionality and system performance.
Prolusion to Wafer Substrates
Wafer substrates are paramount in the realm of modern electronics. They serve as the foundation for various semiconductor devices, influencing their performance, reliability, and efficiency. Understanding wafer substrates provides insight into the broader landscape of electronic development and technology advancement. This section aims to elucidate the significance, definition, and historical context of wafer substrates, setting the stage for deeper exploration into their properties and applications.
Definition and Purpose
Wafer substrates refer to thin slices of semiconductor material used to create integrated circuits and other electronic components. The primary purpose of these substrates is to provide a stable platform for the deposition and patterning of various materials, enabling the development of advanced electronic devices. Their composition often influences electrical, thermal, and mechanical properties critical for device functionality.
In particular, silicon wafers dominate the market due to their favorable electrical properties and cost-effectiveness. The choice of wafer substrate directly impacts the performance metrics of the finished product, such as speed, power consumption, and heat dissipation. This relationship underscores the importance of selecting appropriate substrates for specific applications.
Historical Context
The evolution of wafer substrates parallels the growth of the semiconductor industry. Early attempts at creating electronic components involved rudimentary materials and methods. However, the 1950s marked a turning point when silicon emerged as the preferred semiconductor material. Its availability and characteristic properties facilitated the manufacture of transistors and diodes, leading to the development of integrated circuits.
Over the decades, advancements in crystal growth and wafer fabrication techniques have fostered innovation. For example, methods like the Czochralski process and liquid-phase epitaxy improved production and quality of silicon wafers. Today, various materials such as gallium arsenide and sapphire are also utilized, expanding the applications and capabilities of electronic devices. This historical backdrop highlights how wafer substrates have evolved to meet the rising demands of technology, a trend that continues as we enter new eras of electronics.
Fundamental Properties of Wafer Substrates
Wafer substrates are the backbone of modern electronics, and their fundamental properties critically influence device efficiency and performance. Understanding these properties allows engineers and researchers to make informed choices when selecting substrates for various applications. Factors such as material composition and mechanical characteristics are pivotal in the design and functionality of electronic components.
Material Composition
The material composition of wafer substrates determines their electrical and physical behavior. Different materials offer unique advantages and disadvantages, making them suitable for specific applications in electronics.
Silicon
Silicon is the most commonly used material for wafer substrates. Its key characteristic is its semiconductor properties, which allow it to conduct electricity under certain conditions. This makes silicon an ideal choice for integrated circuits and solar cells. One unique feature of silicon is its abundance in nature and the mature technology surrounding its processing. This leads to lower production costs and widespread availability. However, silicon has limitations in terms of high-speed applications due to its relatively low electron mobility compared to other semiconductor materials.
Gallium Arsenide
Gallium arsenide is another significant substrate material, particularly in optoelectronics. Its key characteristic is its high electron mobility, which allows for faster electronic performance. This makes gallium arsenide favorable for high-frequency devices, such as RF and microwave components. A notable feature of gallium arsenide is its ability to efficiently emit and absorb light, making it essential for LED and laser applications. Nevertheless, the main disadvantage of this material is its cost, which can be significantly higher than silicon, limiting its adoption in mass-market products.
Sapphire
Sapphire is notably used in specialized applications like LEDs and certain MEMS devices. The key characteristic of sapphire is its thermal stability and mechanical strength. This makes it an excellent substrate for components operating at high temperatures. A unique feature of sapphire is its transparency to a wide range of wavelengths, which benefits optoelectronic devices. However, sapphire is more brittle compared to silicon and can present challenges in processing, leading to increased costs.
Mechanical and Thermal Properties
The mechanical and thermal properties of wafer substrates play a crucial role in determining their reliability and suitability for various electronic applications. Key factors include thermal conductivity and mechanical strength.
Thermal Conductivity
Thermal conductivity is vital for managing heat dissipation in electronic devices. A substrate with high thermal conductivity effectively transfers heat away from hot spots, improving the overall reliability and lifespan of the device. For instance, silicon excels in this property, which is one reason for its popularity in the industry. However, some materials might have insufficient thermal conductivity, resulting in device failure under high thermal stress.
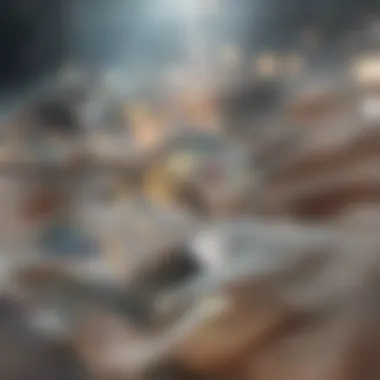
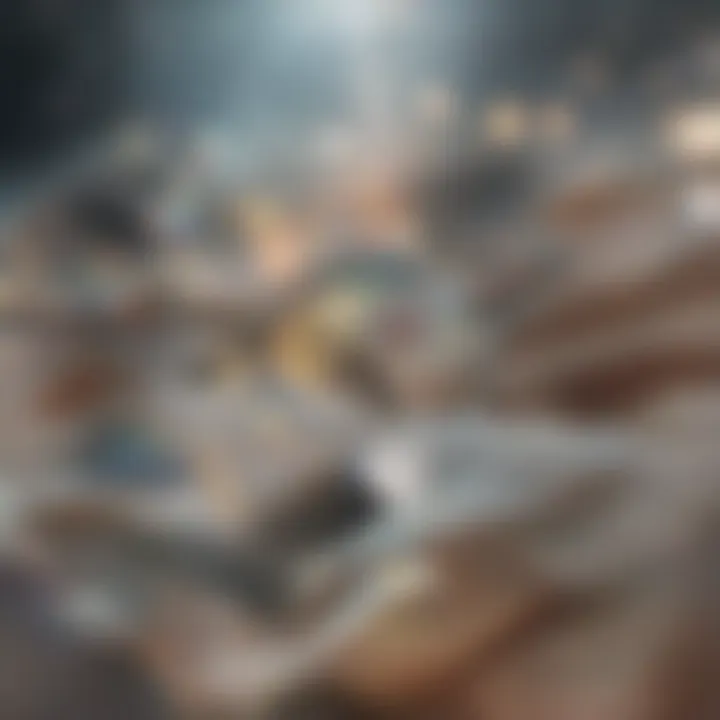
Mechanical Strength
Mechanical strength ensures that the substrates can withstand physical stresses during fabrication and use. Materials with high mechanical strength are less prone to fracturing and can tolerate the rigors of processing. Silicon again demonstrates a good balance between strength and flexibility, making it suitable for various applications. However, there are trade-offs, as substrates with high mechanical strength could also be more challenging to fabricate or process, which may lead to increased costs or complex manufacturing procedures.
The selection of material and its fundamental properties directly impact the performance and reliability of electronic devices.
In summary, the fundamental properties of wafer substrates significantly influence their application in modern electronics. By understanding these aspects, stakeholders can select appropriate materials that meet the demands of their specific technological needs.
Wafer Manufacturing Processes
Wafer manufacturing processes play a crucial role in the production of wafer substrates, key components in modern electronics. These processes encompass a range of techniques designed to create high-quality wafers that meet the demanding specifications of various applications. Understanding these processes is essential for professionals in the field, as they directly influence the performance and efficiency of electronic devices.
Crystal Growth Techniques
Czochralski Process
The Czochralski process is a widely-used technique for producing single-crystal silicon wafers. This method involves melting silicon in a crucible and then slowly pulling a seed crystal upward into the molten silicon. The key characteristic of this technique is its ability to produce large-diameter and high-purity wafers with minimal defects, making it a popular choice for manufacturers.
A unique feature of the Czochralski process is the control it offers over the doping of the silicon. Doping is the introduction of impurities to modify electrical properties, which is crucial for semiconductor applications. However, the process does have disadvantages, such as the high costs associated with maintaining the necessary equipment and the complexity of handling large crystal pulls.
Liquid Phase Epitaxy
Liquid phase epitaxy (LPE) is another important method for growing semiconductor layers. Unlike the Czochralski process, LPE involves the deposition of material from a liquid solution. This technique is particularly favored for its ability to create high-quality, uniform films on substrates. The key characteristic here is the precision with which it can control the thickness and composition of the epitaxial layers.
A unique aspect of LPE is its effectiveness in creating specialized materials, such as compound semiconductors, which are essential for high-frequency and optoelectronic devices. Nevertheless, LPE generally requires longer processing times and can present challenges in achieving large area uniformity, which can limit its application in mass production.
Wafer Fabrication Techniques
Polishing and Cleaning
Polishing and cleaning are essential steps in the wafer fabrication process, ensuring that the surfaces of the wafers are smooth and free of contaminants. The importance of this process cannot be overstated; any imperfections on the wafer surface can lead to issues in subsequent steps, such as lithography and etching.
A key characteristic of polishing is the use of chemical-mechanical planarization (CMP), which combines both chemical and mechanical forces to achieve the desired surface topology. This technique is beneficial for producing wafers with a high degree of flatness, which is critical for modern electronic applications. However, it can be time-consuming and requires careful monitoring to avoid introducing defects.
Thickness Control
Thickness control is another vital aspect of wafer fabrication. It involves measuring and adjusting the thickness of wafers to meet specific standards required for their intended applications. Maintaining consistent thickness is crucial for avoiding variations in electrical properties across the wafer.
The main characteristic of thickness control is the use of specialized tools and techniques, such as laser interferometry. This method offers high precision and can detect even the slightest variations. While thickness control provides significant advantages in ensuring uniformity, it can increase production times and costs, particularly when adjusting panes of different sizes or materials.
Effective wafer manufacturing processes are vital for enhancing electronic device performance while managing production efficiency.
Types of Wafer Substrates
Wafer substrates serve as the foundation upon which a variety of electronic devices are built. This section focuses on the different types of substrates available, which is crucial not only to understand their applications but also to appreciate their specific benefits and limitations. Each type has unique properties that influence its use in various technological contexts. Here you can find the importance these different materials play in semiconductor manufacturing, balancing costs, performance, and scalability needs in modern electronics.
Single-Crystal Wafers
Single-crystal wafers are fundamental in the realm of integrated circuits. Made from high-purity silicon, these wafers exhibit a uniform atomic arrangement. This uniformity enables superior electrical performance and lower defect levels thereby increasing the yield during fabrication. Their reduced crystal lattice disorder translates into fewer electronic traps. Moreover, the high purity of the material ensures that the electrical characteristics remain predictable. This predictability is vital for high-scale and high-performance device applications, such as processors and memory chips, where any variation can lead to substantial inefficiency. The overall impact of single-crystal wafers on device performance underscores their prominence in electronics today.
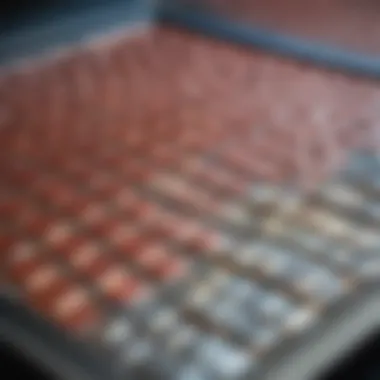
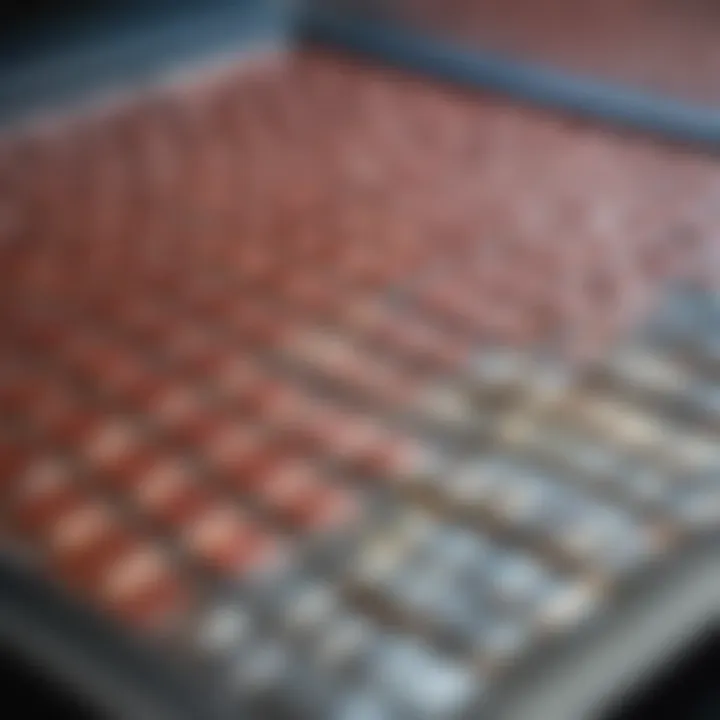
Polycrystalline and Amorphous Wafers
Polycrystalline wafers, while less common than single-crystal varieties, play a significant role in specific applications, particularly for solar cells and less demanding electronic products. Unlike single-crystal wafers which are fabricated from a single continuous crystal structure, polycrystalline wafers are derived from multiple crystallites, which can lead to varied electrical properties. This can be undesirable for high-end semiconductor devices, but it offers a more cost-effective option for mass production.
Amorphous wafers, which lack a defined crystal structure, further lower costs and offer unique advantages in some sectors. They are best suited for thin-film applications, often used in displays and sensors. Their flexibility also opens opportunities in non-conventional markets, such as wearable technologies. Though their performance may not match single-crystal wafers, their versatility and economic benefits highlight their ongoing relevance.
Specialized Substrates
Specialized substrates cater to niche applications within the broad electronics space. Their unique properties can address specific needs that standard wafers cannot meet.
SOI (Silicon on Insulator)
Silicon on Insulator technology involves layering a thin silicon top layer on an insulator, typically silicon dioxide. One of the key characteristics of SOI is its reduced parasitic capacitance, which contributes to higher performance. This property is especially beneficial for high-speed integrated circuits, as it allows for faster operation with lower energy consumption. The capability for effective power management makes SOI wafers a popular choice for advanced microprocessors and RF devices. However, the higher costs associated with SOI fabrication can be a limiting factor, especially in lower-end applications.
LED Substrates
LED substrates are specific types of wafers used primarily in light-emitting diode technology. The most prominent materials include sapphire and silicon carbide. These substrates allow for efficient light emission and thermal management, vital in achieving high brightness and low energy consumption in LED designs. One important characteristic of LED substrates is their thermal conductivity, which significantly impacts the lifespan and efficiency of the assembled devices. However, challenges in matching thermal expansion properties and costs can affect their applicability depending on the technology in use.
The type of wafer substrate selected can profoundly affect the performance and reliability of electronic devices. It is essential that designers understand these differences when making material choices.
Applications of Wafer Substrates
Wafer substrates play a vital role in modern electronics. They serve as the foundational layers for a multitude of electronic devices. These substrates support the intricate arrangements of components necessary for functionality. Understanding these applications helps clarify how wafer substrates contribute to advancements in technology.
Integrated Circuits
Integrated circuits, commonly referred to as ICs, are one of the primary applications of wafer substrates. These circuits combine various electronic elements into one compact form. Silicon wafers are most commonly used for IC fabrication due to their excellent electrical properties. The efficiency and performance of ICs heavily depend on the wafer substrate. Without well-manufactured wafers, ICs may exhibit deficiencies in speed and power consumption. Moreover, the scaling down of components in ICs has made precise wafer properties even more critical. Tolerance in thickness, flatness, and impurity levels all influence performance. Quality control in wafer production directly affects the reliability of integrated circuits.
Optoelectronics
Optoelectronics combines optics and electronics, using wafer substrates for devices that interact with light. Materials like gallium arsenide are frequently employed for optoelectronic applications. These substrates facilitate the production of components such as lasers and light-emitting diodes (LEDs). In the realm of optoelectronics, substrate selection can impact efficiency and wavelength characteristics. For example, a well-chosen substrate can enhance light emission or improve detection capabilities. As technology evolves, new materials and structures are being explored, including thin films that may optimize optical performance further.
MEMS (Micro-Electro-Mechanical Systems)
Micro-Electro-Mechanical Systems, or MEMS, are another key application of wafer substrates. MEMS technology incorporates tiny mechanical and electronic elements on a single chip. Wafer substrates provide the necessary platform for fabrication. Depending on the design, various materials can be employed, including silicon or specialized biodegradable substrates. MEMS devices find use in sensors, accelerometers, and gyroscopes. Their functionality greatly relies on substrate properties like stiffness and thermal stability. Therefore, higher efforts are made in research and development to enhance MEMS performance through advanced substrate technology.
"Wafer substrates are indispensable in the functioning of modern electronic devices, from ICs to MEMS, enhancing performance and efficiency."
In summary, the applications of wafer substrates are extensive and critical to various electronic sectors. The choice of materials and manufacturing processes has profound implications on device efficiency, reliability, and overall technological advancement.
Emerging Trends in Wafer Substrate Technology
The landscape of wafer substrate technology is evolving rapidly. Recognizing emerging trends is crucial for students and professionals involved in electronics. It highlights how advances in substrate technology can influence electronic performance. New materials and methodologies are creating pathways to enhance device efficiency and functionality. As electronics become more complex, understanding these trends is increasingly important.
Advancements in Material Science
Material science plays a pivotal role in the development of modern wafer substrates. Recent advancements have introduced new materials that exhibit desirable properties, such as reduced weight and increased thermal conductivity.
- Silicon Carbide (SiC): This compound semiconductor is ideal for high-power applications. With improved thermal management, it allows for better heat dissipation.
- Gallium Nitride (GaN): GaN is becoming popular for high-frequency applications. Its wide bandgap property leads to higher performance in compact circuits.
- 2D Materials: Emerging materials like graphene are gaining traction. Their atomic thickness enables unique electronic properties that can be harnessed for advanced applications.
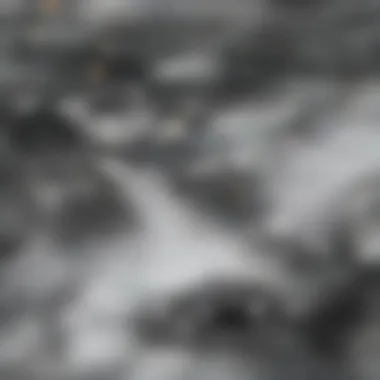
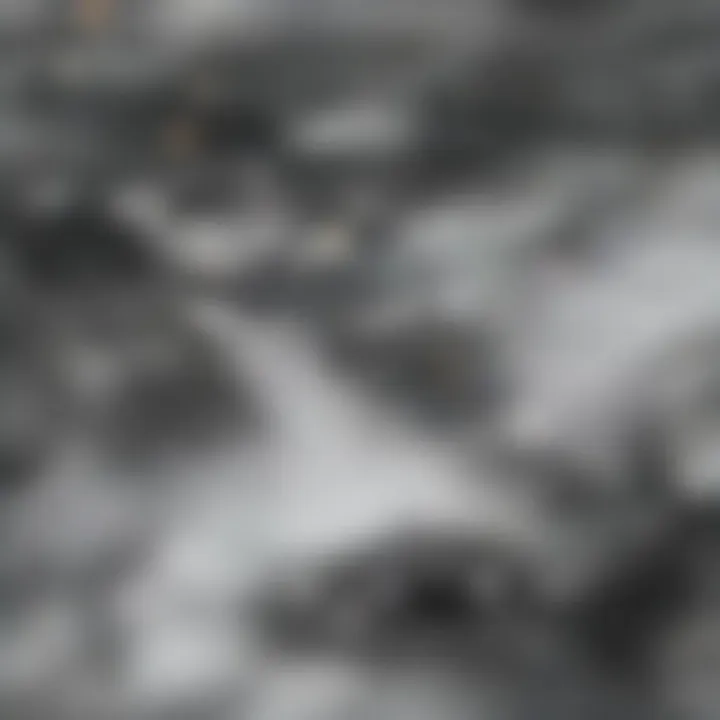
By incorporating these advancements, manufacturers can improve substrate performance criteria such as durability and efficiency. Incorporating innovative materials can lead to the miniaturization of electronic devices without compromising performance.
Nano-Structured Substrates
Nano-structured substrates represent a forward-thinking approach to wafer technology. The pursuit of higher performance electronics has prompted researchers to explore nanoscale designs.
- Enhanced Surface Area: Nano-structured designs increase the surface area, which can enhance reaction rates in various applications. This can be beneficial in fields such as sensors and catalysis.
- Improved Electrical Properties: These substrates can exhibit unique electrical properties due to quantum effects that manifest at this scale. This can lead to better electrical conductivity and faster response times.
- Customization: The nano-scale structure allows for customization based on specific application needs. Tailoring properties to fit particular electronic requirements has potential benefits in specialized applications.
In summary, the integration of nano-structured substrates is a promising direction in electronics, catering to the ever-increasing demand for high performance and efficiency in devices.
"Emerging trends in wafer substrate technology show that innovation in materials and structure is critical for the future of electronics."
Understanding these trends not only prepares professionals for upcoming challenges but also opens doors to new opportunities in semiconductor technology.Updated methods and materials can lead to higher performance devices, ultimately transforming how we think about and use electronics.
Environmental Considerations
The discussion surrounding wafer substrates is not only about technological advancements and electroic performance, but also about the environmental impact of their production and life cycle. As the electronics industry grows, concerns about sustainability and resource management become increasingly pertinent. Understanding environmental considerations in wafer production and recycling processes helps ensure that the semiconductor sector minimizes its ecological footprint.
Sustainability in Wafer Production
Sustainability in wafer production involves several key components that work together to reduce environmental harm. First, optimizing material usage is essential. The process of creating wafers can be resource-intensive, consuming significant amounts of energy and raw materials. Adopting energy-efficient methods in manufacturing can significantly reduce energy consumption. Techniques like the improved Czochralski process leverage less energy and improve yield, minimizing waste.
Moreover, the choice of materials plays a crucial role in sustainability. Many companies are shifting towards using recycled silicon and other eco-friendly materials. The integration of these materials promotes a circular economy, significantly lowering the demand for virgin resources. Additionally, some manufacturers are exploring alternatives like gallium arsenide and silicon carbide, which offer better performance with potentially lower ecological impact.
It's also vital to consider the chemicals used in the production process. Implementing greener chemistry practices can help limit hazardous waste. There are initiatives to replace toxic solvents and cleaners with less harmful substances, which not only support health standards but also reduce environmental contamination.
Recycling Processes
Recycling processes for wafer substrates are gaining traction as technology progresses and waste management practices evolve. In electronics, a large amount of material can often be reclaimed and repurposed. Silicon wafer recycling is particularly significant because it recovers valuable materials, which can be reintegrated into the production cycle.
The recycling process typically begins with the collection of discarded wafers from manufacturers and device end-of-life products. After collection, the wafers undergo various handling procedures to separate useful materials from waste. This stage is critical in ensuring purity for subsequent processing. Some of the key methods in recycling silicon wafers include:
- Physical recycling: This method involves breaking down wafers into smaller pieces before purifying the silicon for reuse.
- Chemical recycling: In this process, chemicals are used to dissolve unwanted materials, allowing for the recovery of high-purity silicon.
Despite the technological advancements, there are still barriers that recycling faces, such as contamination of materials and the costs of processing. However, with growing pressure for better environmental practices, investments in this sector are increasing. Organizations are actively promoting recycling as a vital element of a sustainable electronic industry.
"Adopting sustainable practices in wafer production and recycling is not just beneficial for the environment, but also contributes to long-term economic viability for manufacturers."
The End
The conclusion of this article brings to light the multifaceted role of wafer substrates in the world of modern electronics. It serves as a capstone to the discussions presented, reinforcing the significance of wafer substrates in ensuring the performance, reliability, and efficiency of electronic devices. Each highlighted topic contributes to a deeper understanding of how different substrate materials, manufacturing processes, and application areas combine to shape the future of electronics.
Wafer substrates are not merely a backdrop to semiconductor technologies; they are integral to the development and functionality of various electronic components. As materials evolve and new technologies emerge, ensuring the right substrate selection becomes more critical. This understanding can help professionals in the field make informed choices, leading to advancements in product performance and sustainability.
Summary of Key Points
- Material Significance: The choice of substrate material, such as silicon or gallium arsenide, directly influences device efficiency and thermal management.
- Manufacturing Processes: Nuanced processes like the Czochralski method and wafer polishing are crucial for producing high-quality substrates.
- Types of Wafers: Awareness of the differences between single-crystal, polycrystalline, and specialized substrates allows for better application matching.
- Environmental Responsibility: The need for sustainable practices and recycling in wafer production is increasingly recognized, highlighting the industry's commitment to reducing its environmental footprint.
Future Outlook
The future outlook for wafer substrates is promising, reflecting ongoing advancements in technology and materials science. New materials, such as 2D materials or organic substrates, may emerge as viable alternatives, potentially broadening the range of applications. Research is also focused on improving substrate characteristics for higher efficiency in solar cells and increasing performance in integrated circuits.
Additionally, nano-structured substrates hold promise for enhancing electronic device capabilities.
The nexus between substrate technology and overall functionality in electronics indicates a trajectory of growth and innovation. As industries push for better performance with an emphasis on sustainability, wafer substrates will likely continue to play a pivotal role in shaping the electronics landscape.