Urea Manufacturing: Processes and Future Trends
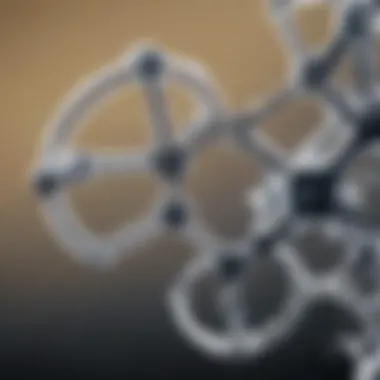
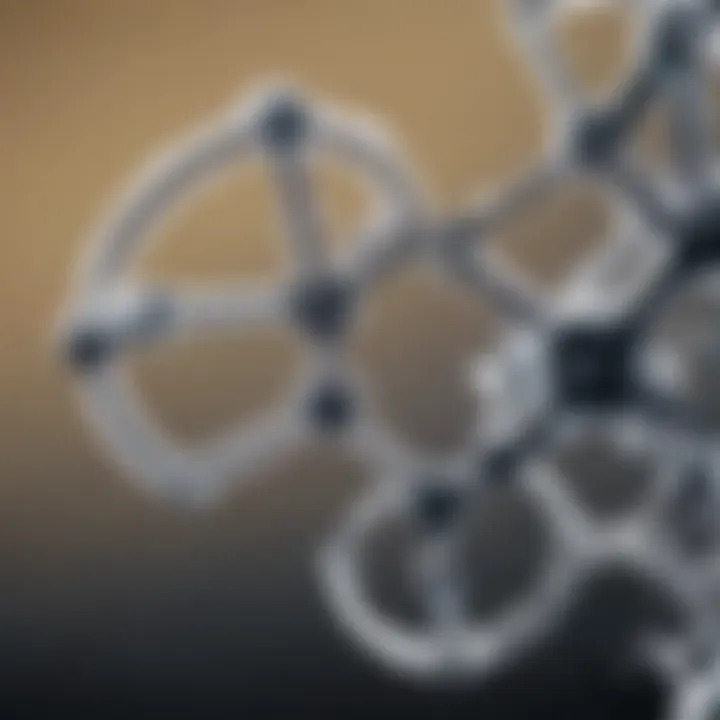
Intro
Urea is much more than just a nitrogenous compound; it is a cornerstone of modern agriculture and various industrial processes. From fertilizers that enrich the soil to raw materials in chemical manufacturing, the significance of urea extends well beyond its simple molecular structure. The journey from raw materials to urea crystals involves a series of complex reactions and methodologies, each with its own unique challenges and benefits. Understanding these processes not only sheds light on the efficiency of urea production but also the broader implications for environmental sustainability and future innovations.
As the world grapples with the challenges of food security and environmental preservation, appreciating the intricacies of urea manufacturing has never been more crucial. It invites a multidimensional exploration into its significance, applications, and potential future directions.
Research Overview
Summary of Key Findings
The research highlights several key findings in the landscape of urea manufacturing:
- Chemical Reactions: The primary reaction for urea synthesis involves ammonia and carbon dioxide under high pressure and temperature, leading to the formation of urea and water.
- Production Methods: Various methods like the Bosch-Meiser process and the more contemporary, energy-efficient techniques are explored.
- Applications: Urea serves as a fundamental component in fertilizers, while also finding uses in plastics and pharmaceuticals.
- Environmental Considerations: The release of ammonia during production and urea's role in soil nitrogen emissions underscores the need for sustainable practices.
These findings lay the groundwork for further exploration into how advancements in urea production technology can mitigate environmental impacts while meeting global demand.
Methodologies Employed
The methodologies for researching urea manufacturing were diverse and robust, featuring:
- Literature Review: An extensive analysis of existing literature on urea production processes and their efficiencies.
- Field Studies: Observations were made in agricultural settings where urea was utilized to gauge effectiveness compared to other fertilizers.
- Comparative Analysis: A side-by-side evaluation of traditional vs. modern methods highlighted advancements in technology and sustainability.
Through these methodologies, the research aimed to present a holistic view of urea manufacturing, illustrating its vital role in addressing pressing global challenges.
In-Depth Analysis
Detailed Examination of Results
The intricate details of urea's synthesis process reveal the rich chemistry involved. The Haber-Bosch process, crucial for ammonia production, stands out as the foundation for urea synthesis. The synthesis reaction can be simplified into:
[ \text2NH_3 + \textCO_2 \rightarrow \text(NH_2)_2\textCO + \textH_2\textO ]
This reaction underscores the pivotal role nitrogen management plays in agricultural systems, where soil fertility is directly tied to urea application. Research showed that adapting modern methods, such as the TAMU process, could significantly reduce energy consumption by optimizing reaction conditions.
Comparison with Previous Studies
The evolution of urea manufacturing practices has been marked by a transition from rudimentary systems to sophisticated, automated facilities. Previous studies emphasize the environmental hazards linked with nitrogen leaching—a problem that sustainable practices are poised to tackle in the future.
The contemporary emphasis on circular economy principles shows great promise. Technologies that harness byproducts from urea production—such as carbon capture and storage—are emerging as viable options to counter the industries' carbon footprint.
"By rethinking how we approach urea manufacturing, we can lead a charge towards a more sustainable future."
This shift in focus towards sustainability is not just a necessity but a roadmap towards ensuring that urea remains a key player in both agriculture and industrial applications without compromising environmental integrity.
End
The exploration of urea manufacturing processes is not just an academic exercise; it's a crucial discourse as we seek to harmonize agricultural productivity with environmental stewardship. As advancements in technology develop, the future of urea manufacturing looks to blend efficiency with sustainability, ultimately paving the way for more responsible practices in agriculture and industry. Expanding on these findings and methodologies will be crucial as we move forward—both in academic circles and beyond.
Prolusion to Urea
Urea plays a crucial role in the landscape of modern agriculture and various industrial applications. Understanding urea isn't merely a matter of chemical curiosity; it has far-reaching implications that touch both environment and economy. As the most prominent nitrogenous fertilizer, urea is a key player in our food production systems and a vital resource in chemical manufacturing.
Definition and Chemical Structure
Urea, chemically represented as CO(NH₂)₂, is a white crystalline substance made up of carbon, nitrogen, oxygen, and hydrogen. It is typically synthesized through a series of chemical reactions involving ammonia and carbon dioxide. The molecular structure features a carbon atom double-bonded to an oxygen atom and single-bonded to two amine groups, presenting a stable compound that can easily be absorbed by various biological systems. This simplicity in its structure is what allows urea to work effectively as a fertilizer, providing crops with a readily accessible source of nitrogen, which is imperative for plant growth.
Historical Context
The story of urea begins long before it became a staple in fertilizers. Discovered in the early 18th century by Dutch chemist Hermann Boerhaave, urea was initially viewed as a waste product of animal metabolism. However, it wasn’t until 1828 that Friedrich Wöhler made a significant breakthrough by synthesizing urea from ammonium cyanate, marking the first time an organic compound was synthesized from inorganic precursors. This discovery reshaped prevailing thoughts about organic compounds and laid down the academic groundwork for envisioning the synthesis of complex molecules.
As the agricultural revolution gained momentum in the late 19th and early 20th centuries, the demand for effective fertilizers surged. Urea soon emerged as a powerful contender due to its high nitrogen content and efficiency. Today, it stands as one of the most produced chemicals globally, with production methods evolving to become more efficient and environmentally conscious.
Urea's journey from an animal waste product to a vital agricultural fertilizer highlights the shifting paradigms and immense potential of chemistry in society.
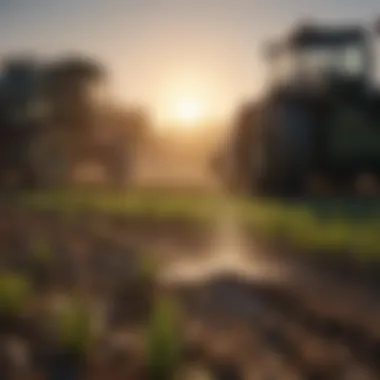
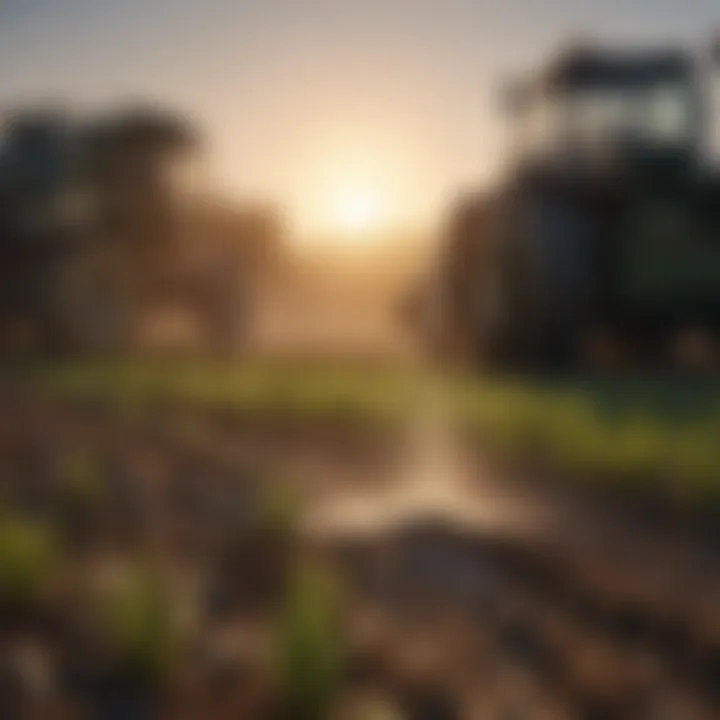
The understanding of urea has thus transitioned from mere academic interest to a network of intricate applications influencing food security and industrial processes worldwide. With the ever-increasing need for sustainable agriculture and efficient industrial chemistry, continuing to explore urea's properties, production processes, and applications holds paramount importance.
The Significance of Urea
Urea holds a pivotal position in both agricultural and industrial contexts, straddling the line between essential agricultural inputs and vital components in various chemical processes. Understanding its significance is crucial, given that it directly influences food production, resource management, and the development of numerous background products that we often take for granted.
Role in Agriculture
The agricultural industry is one of the main beneficiaries of urea, particularly as a nitrogen fertilizer. Nitrogen is a key nutrient for plant growth, and when it comes to boosting crop yields, urea is a front-runner in this area. Here are some points to consider:
- High Nitrogen Content: Urea typically contains about 46% nitrogen by weight, making it one of the most concentrated nitrogen fertilizers available.
- Cost-Effectiveness: Urea is often more affordable than other nitrogen sources. This factor makes it an attractive option for farmers, especially those operating on a tight budget.
- Versatility in Use: Urea can be applied before planting, during, or after crops have emerged, allowing for flexibility in farming practices.
- Conversion Efficiency: When plants absorb urea in soil, it gets converted into ammonium and nitrate, both of which plants can readily utilize. This process significantly enhances crop growth.
The benefits extend further; studies have shown that farmers using urea-based fertilizers often report increased yields, which can lead to higher profitability and improved food security. However, we must discuss the concerning aspects surrounding urea application that can impact the environment, such as nitrogen runoff.
Industrial Applications
In the industrial realm, urea's significance cannot be overstated. It is involved in various processes that range from the manufacture of plastics to the synthesis of chemicals. Here’s a closer look:
- Synthesis of Other Chemicals: Urea is a precursor for several compounds, including melamine, an important ingredient in laminates and resins.
- Component in fertilizers: Beyond its role as a direct fertilizer, it serves as a building block in the production of other fertilizers, like ammonium nitrate.
- Manufacturing of Resins: Urea-formaldehyde resins are widely utilized in the wood industry, offering durable adhesives and finishes for products.
- Pharmaceutical Use: Urea is sometimes used in dermatological preparations, helping to treat skin conditions such as psoriasis by maintaining moisture balance in the skin.
Overall, urea acts as a linchpin linking agriculture and industry, demonstrating immense versatility and utility. This makes it indispensable in today's economy.
The significance of urea extends beyond its use as a fertilizer, permeating various sectors and influencing multiple facets of commerce and environmental interaction. Such a multifaceted role warrants a comprehensive discussion on its implications for sustainable practices, which are increasingly vital as the world strives to address associated challenges.
Chemical Reactions in Urea Production
The section on chemical reactions in urea production is fundamental because it lays the groundwork for understanding how urea, one of the most crucial nitrogen sources in agriculture, is formed. These reactions not only underpin the manufacturing process but also define the efficiency and efficacy of urea as a fertilizer. By examining these chemical transformations, we can grasp their implications for operational practices, economic viability, and environmental concerns inherent in the production of urea.
Formation of Urea from Ammonia and Carbon Dioxide
The core reaction in urea synthesis involves combining ammonia and carbon dioxide. This fundamental process is often simplified but certainly deserves detailed scrutiny. The reaction can be expressed in a classical format as follows:
[ \text2 NH_3 + \textCO_2 \rightarrow \text(NH_2\text)_2\textCO + \textH_2\textO ]
In layman's terms, two molecules of ammonia react with one molecule of carbon dioxide to produce one molecule of urea and a water molecule. But simple as it seems, this reaction occurs under specific conditions — elevated temperature and pressure are key to ensuring that the reaction proceeds efficiently and yields a high percentage of urea.
The role of ammonia is paramount, as it originates from nitrogen fixation processes or synthesis gas derived from natural gas. The carbon dioxide, which is often a byproduct of industrial processes, contributes not only to the urea formation but also plays a role in mitigating greenhouse gases, making this reaction rather significant from a sustainability perspective.
A few aspects to consider are:
- Catalytic Influence: Here’s where catalysts come into the picture. They speed up the reaction without being consumed, significantly enhancing the efficiency and output.
- Energy Use: The energy input for maintaining the right temperature and pressure can affect the cost and carbon footprint of the urea manufacturing process.
- Yield Efficiency: Understanding the stoichiometry and dynamics of this reaction helps in scaling up production for industrial needs while maintaining environmental balance.
This synthesis not only serves the agricultural market but also feeds into diverse sectors, including animal feed and even industrial chemical processes. With such a wide application spectrum, it's clear that the formation of urea is a topic that demands intricate understanding.
The Bosch-Meiser Process
The Bosch-Meiser Process is one of the historical benchmarks in urea production, a bit like an old classic that still delivers hits in modern contexts. This process represents the industrial method for synthesizing urea and builds on the earlier foundational reactions of ammonia and carbon dioxide. What sets it apart is its operating conditions and configuration.
In the Bosch-Meiser Process, ammonia and carbon dioxide are combined in a reactor under high-pressure scenarios. As the reaction occurs, urea is formed along with ammonium carbamate, an intermediate compound that decomposes into urea and water upon heating. This step is crucial because it allows for continuous production. No more waiting around for the batch to complete; it’s more of a constant flow operation.
The essence of the Bosch-Meiser Process includes:
- Reaction Chambers: The design typically features multiple reaction chambers that facilitate various stages of the temperature and pressure controls.
- High Efficiency: By recycling unreacted ammonia and carbon dioxide, this process minimizes waste and optimizes resource utilization.
- Environmental Impact: Less waste means less potential for pollutants — a win-win scenario for producers and the environment alike.
"The Bosch-Meiser process is not just a production method; it's a testament to innovation within the chemical industry."
Additionally, this method is widely recognized for its adaptability; modern implementations often incorporate feedback loops and sensors, thus driving efficiency even further. In a world grappling with the implications of resource usage, processes like Bosch-Meiser offer a glimpse of sustainable chemical manufacturing.
Understanding these reactions and processes reveals the layers of complexity involved in something as seemingly simple as urea. As we delve deeper into the facets of urea manufacturing, the significance of these chemical reactions becomes clearer, showcasing their pivotal role in positioning urea as a cornerstone in agricultural practices.
Manufacturing Processes
The manufacturing processes of urea play a pivotal role within this article, offering insights into how this vital compound is produced on a large scale. Understanding these processes helps to illustrate the complexities of urea synthesis, the potential efficiencies to be gained, and the impact on both environmental sustainability and industry standards. This exploration reveals not just the how-tos of production but also the why's, highlighting the motivations behind technological advancements and the pressing need for sustainable practices.
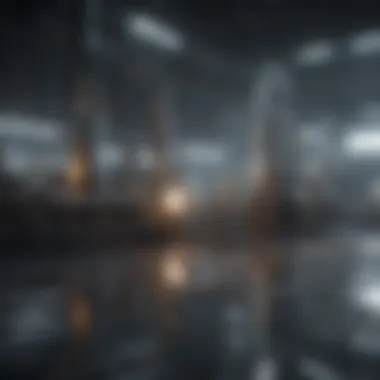
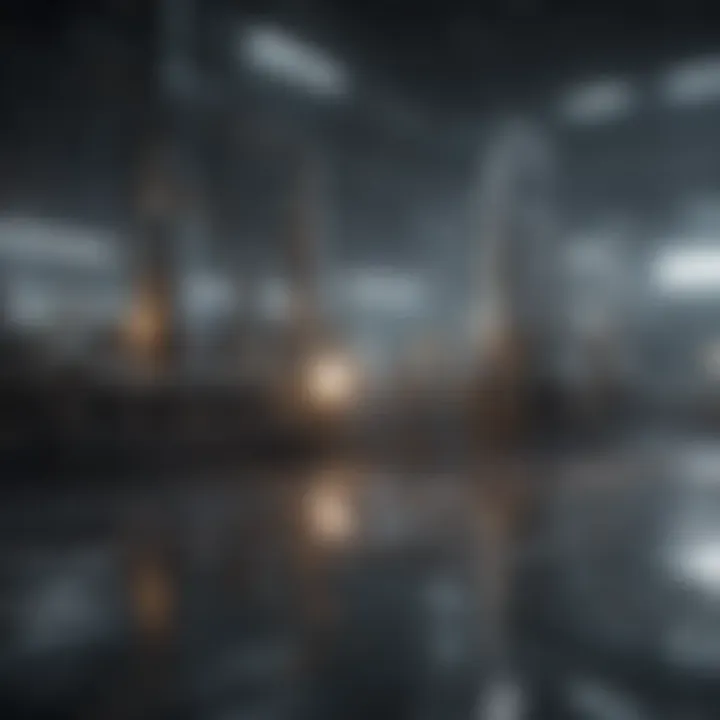
Traditional Methods of Urea Production
Traditional methods of urea production began with the synthesis of urea from ammonia and carbon dioxide. The earliest commercial processes date back to the early 20th century, heavily relying on the Haber-Bosch process. This method combines nitrogen from the air with hydrogen derived from natural gas—a simple yet effective equation of combining raw materials to result in urea. However, it is important to note the significant energy inputs needed, which raises questions about efficiency and sustainability.
The urea synthesis reaction from ammonia and carbon dioxide can be expressed as:
[ \text2 NH_3 + \textCO_2 \rightarrow \text(NH_2\text)_2\textCO + \textH_2\textO ]\
This reaction typically occurs at high pressures and elevated temperatures, making it an energy-intensive process. In many traditional setups, urea is concentrated through evaporative methods. The end result is a solid or liquid fertilizer, but the energy consumption can be a double-edged sword, compounding operational costs while also generating greenhouse gas emissions.
Advancements in Continuous Production Techniques
Recent years have marked a shift towards continuous production techniques that promise to mitigate the limitations of traditional methods. One such innovation is the use of integrated loop reactors, which streamline the urea synthesis by allowing for a constant flow of reactants and products. This dynamic method not only enhances productivity but also improves the overall yield of urea, as it reduces the time spent in production.
Continuous production brings with it other considerations:
- Reduced energy consumption: By minimizing the time and the energy invested in heating and pressurizing large batch reactors, manufacturers can lower their overall operational footprint.
- Higher safety standards: With continuous processes, the risks associated with having large quantities of volatile chemicals in a single batch are reduced.
- Flexibility in production: This approach allows for quick adjustments in formulations or production levels to meet fluctuating market demands.
In short, advancing towards continuous production methods does not just represent a leap in the efficiency frontier but also aligns better with the growing global focus on sustainability.
Impact of Catalysts on Efficiency
Catalysts are unsung heroes in the quest for more efficient urea production. The role of catalysts in manufacturing cannot be overstated, particularly when it comes to enhancing reaction rates without needing to elevate temperatures drastically. The introduction of newer catalyst materials has led to significant improvements in the synthesis process.
For instance, research indicates that transitional metal oxides, like those based on iron and titanium, can dramatically increase conversion rates. Here’s how they work their magic:
- Catalysts lower the activation energy for the reaction, making it easier for ammonia and carbon dioxide to react effectively.
- With improved reaction kinetics, plants can generate urea at lower energy costs.
- The result is both an economic and environmental win, as reduced energy consumption translates to lower emissions.
"Innovation is the key to addressing the challenges posed by traditional manufacturing methods. The integration of catalysts represents just one part of a broader commitment to improving efficiency and sustainability within the industry."
Thus, it’s clear that the consideration of efficient manufacturing processes, from traditional methods to innovative advancements, is critical. Each step contributes to a holistic understanding of urea production and its far-reaching implications in sectors like agriculture and industry.
Urea in Fertilization
Urea plays a pivotal role in fertilization, acting as a cornerstone in modern agriculture. Its importance stretches beyond just being a nitrogen source—it also influences crop yields, soil health, and sustainability practices. With growing global food demands, understanding urea’s contribution becomes crucial. It provides essential nutrients to crops, streamlining the process of their growth and development. This section focuses on various types of urea fertilizers, the mechanisms through which these nutrients are delivered, and the broader implications on soil quality.
Types of Urea Fertilizers
There are several types of urea fertilizers available, each tailored for specific agricultural needs. Some common forms include:
- Granular Urea: This is the most widely used form. It can be easily spread over fields and dissolves slowly, providing a steady release of nitrogen.
- Liquid Urea Solutions: These are often utilized for foliar applications and soil injection. They provide more immediate nutrient availability, addressing crops' needs swiftly.
- Controlled-Release Urea: Engineered to dissolve gradually, this type helps optimize nutrient absorption and minimizes leaching into water sources.
- Urea Ammonium Nitrate (UAN): A combination of urea and ammonium nitrate, UAN offers both quick- and slow-release nitrogen, making it versatile for different crop cycles.
Each form has its merits depending on soil types, climatic conditions, and specific crop requirements, thereby allowing farmers to make informed choices based on their unique circumstances.
Nutrient Delivery Mechanisms
Understanding how urea fertilizers deliver nutrients to plants is critical for maximizing their effectiveness. When applied to soil, urea undergoes hydrolysis, a process where it is broken down into ammonium carbonate. Subsequently, it transforms into ammonium and nitrate forms, which plants can readily utilize for growth.
- Ammonium Form: Retained in soil due to positive charge, enhancing its availability for plant roots.
- Nitrate Form: As it is negatively charged, it can leach with water. This emphasizes the need for proper application techniques to minimize nutrient loss.
In addition, various application methods, such as broadcasting, banding, and fertigation, can influence how effectively plants uptake the nutrients. With careful consideration of timing and method, farmers can greatly enhance nutrient delivery, resulting in healthier crops.
Impact on Soil Quality
The use of urea fertilizers has profound impacts on soil quality, influencing factors such as microbial activity, pH levels, and overall soil fertility. Regular application leads to increased nitrogen levels, crucial for various soil organisms that promote nutrient cycling and organic matter decomposition.
However, excessive urea application can lead to detrimental effects:
- Soil Acidification: High quantities of nitrogen can lower pH, making soil less hospitable for some crops.
- Nutrient Imbalance: Relying too much on nitrogen without considering other nutrients can lead to deficiencies and diminished crop health.
- Eutrophication Risks: Runoff from fields treated with urea can contaminate water bodies, leading to algal blooms that threaten aquatic ecosystems.
To mitigate these issues, practices such as soil testing and integrated nutrient management can be pivotal. By finding the right balance, urea can enhance rather than detract from soil health, paving the way for sustainable agriculture.
"Proper use of urea fertilizers can be a game changer in maintaining soil health and enhancing crop productivity. Yet, it comes with responsibilities that cannot be overlooked."
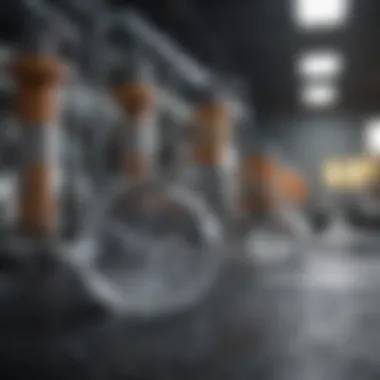
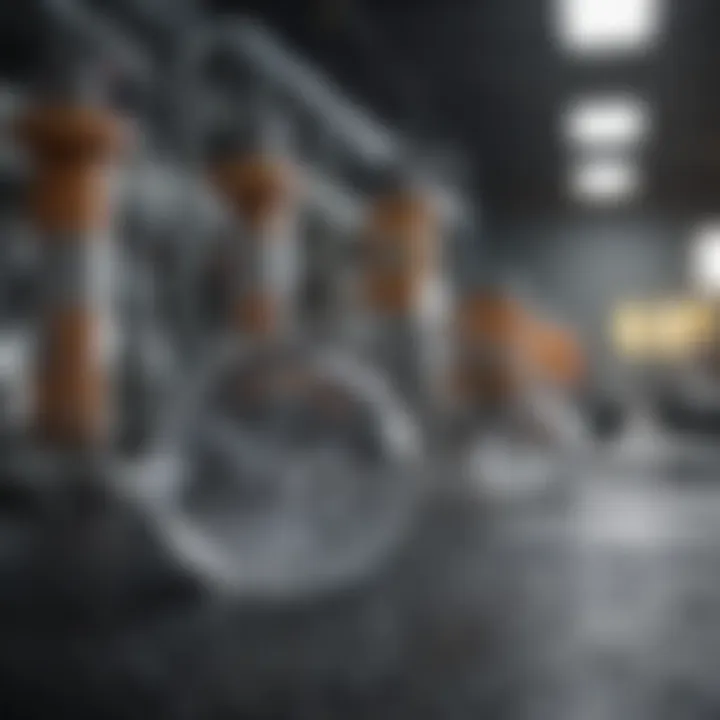
In summary, urea serves as a fundamental element in fertilization strategies. By addressing the different types of urea fertilizers, understanding nutrient delivery mechanisms, and recognizing their impacts on soil quality, one can appreciate the intricate balance needed in sustainable agriculture.
Urea in Chemical Processes
Urea is often considered the linchpin of various chemical processes beyond its well-known role in agriculture. Its significant positioning in the chemical industry stems from its ability to serve as both a reactant and a product in numerous important reactions. The versatility of urea allows it to be a catalyst in synthesizing other chemicals, which in turn, has far-reaching implications for the industrial sector.
Synthesis of Other Chemicals
The synthesis of urea plays a pivotal part in producing various chemicals that are essential for both everyday products and specialized applications. Urea acts as a nitrogen source and feeds into the manufacturing of other nitrogenous compounds. This is particularly important in the production of fertilizers that enrich the soil.
- Common Processes: The formulation of chemicals like ammonium carbonate and biuret are examples where urea is crucial. In these reactions, urea's stable yet reactive nature facilitates the production of compounds necessary for agricultural and industrial applications.
- Ammonium Nitrate Production: One of the notable reactions that involve urea is the reaction with nitric acid to produce ammonium nitrate. This compound is used extensively in fertilizers and explosives, showcasing urea's dual utility in both agriculture and industrial processes.
Moreover, urea's role extends to the synthesis of resins. For instance, in the process of creating urea-formaldehyde resins, urea reacts with formaldehyde. This relationship has significant implications in the production of durable plastics used in cabinetry, flooring, and various construction materials.
Role in Plastics and Resins
Urea's influence isn't just limited to fertilizers; it significantly contributes to the plastics industry. Urea-formaldehyde resins have carved out a niche in the market due to their excellent adhesive properties and affordability. These resins, produced from the reaction between urea and formaldehyde, are reliable and are leveraged in numerous applications.
- Durability and Versatility: These resins are particularly appreciated for their strength and resistance to heat, making them valuable for hard surface applications. They are often found in particleboard and MDF, both of which are staples in furniture manufacturing.
- Thermosetting Nature: The thermosetting quality of urea-formaldehyde resins means they do not melt upon heating, which contributes to their strength and utility in various applications, including electrical components and construction materials.
Urea’s significance in the chemical processes transcends being a mere compound; it is a cornerstone that supports a wide range of industrial applications. By enabling the synthesis of other chemicals and playing an essential role in plastics, urea continues to demonstrate its multifaceted importance in modern manufacturing.
"Urea isn’t just a fertilizer; it’s a foundational compound in our everyday products and industrial successes."
This level of involvement highlights the need for ongoing research into optimizing the chemical processes involving urea, so as not to limit its potential in industrial innovation but rather to expand its capabilities even further.
Environmental Considerations
When discussing urea manufacturing, environmental considerations take center stage. Urea plays a significant role in agriculture and industry, yet its production and application are not without environmental challenges. Understanding these factors is essential for developing sustainable practices that mitigate negative impacts while maintaining agricultural productivity.
The discussions around urea often evoke the conversation surrounding nitrogen runoff and its consequential effect on water pollution. Waterways can become laden with nitrate, resulting in problems like eutrophication, which plummets oxygen levels in water. Recognizing these issues informs more responsible practices in urea production and distribution.
Nitrogen Runoff and Water Pollution
Nitrogen runoff is one of the prominent dilemmas tied to urea application in agriculture. When excessive urea is applied to crops, only a portion gets absorbed by plants. The rest washes away during rain or through irrigation, entering neighboring streams and rivers. As nitrogen builds up in these water systems, it stimulates the overgrowth of algae, creating harmful algal blooms that consume oxygen. This depletion spells danger for aquatic life, disrupting entire ecosystems.
Some specific implications of nitrogen runoff include:
- Depleted Oxygen Levels: Plants and animals in water bodies suffer when oxygen is low, often leading to fisheries collapsing.
- Contaminated Drinking Water: Elevated nitrate levels in drinking water can pose health risks, particularly for infants.
- Loss of Biodiversity: The disruption of aquatic ecosystems can lead to loss of fish species and other aquatic organisms.
Addressing these issues calls for innovative strategies that reduce runoff from fertilizers while ensuring efficient crop growth.
Urea Application Techniques to Minimize Impact
To combat the challenge of nitrogen runoff, various urea application techniques are being implemented. These strategies aim to optimize nitrogen use while reducing its environmental footprint:
- Slow-Release Urea Fertilizers: These fertilizers release nitrogen gradually, allowing for better uptake by plants and minimizing the potential for runoff.
- Controlled Application Timing: Using urea during optimal weather conditions—when rains are less likely to wash it away—helps reduce losses.
- Precision Farming: Employing technology like drones and soil sensors can help farmers apply urea more accurately based on the specific nutrient needs of their crops.
- Split Application: Instead of applying all the urea at once, farmers can apply it in smaller doses throughout the growing season, improving efficiency and reducing runoff.
"Innovative practices in urea application not only support sustainable agriculture but also help protect vital water resources."
Future Directions in Urea Manufacturing
The realm of urea manufacturing is undergoing substantial transformations, driven by evolving demands and increasing sustainability concerns. As the foundation for fertilizers and numerous industrial applications, how urea is produced holds significant implications for the agricultural sector and environmental health alike. Future directions in urea manufacturing not only promise improvements in efficiency and productivity but also target innovations that align with sustainable practices.
Innovative Research and Developments
Recent advancements in urea production technologies mirror the industry's need for optimization and adaptability. Research is increasingly focused on refining the current production methods. \n- Carbon Capture Utilization: One noteworthy innovation is the integration of carbon capture technologies within urea plants. These approaches have the potential to reduce greenhouse gas emissions significantly, transforming carbon dioxide that would otherwise escape into the atmosphere into a feedstock for urea synthesis. This not only decreases the carbon footprint of the manufacturing process but provides a circular economy model for carbon use.
- Microbial Urea Production: Another exciting avenue revolves around biotechnology. Some studies are exploring specific microbes that can synthesize urea directly from atmospheric nitrogen. Though the processes are not yet economically viable on a large scale, ongoing research could revolutionize urea production by providing an alternative to the existing energy-intensive methods.
- Alternative Feedstocks: Research is also redirecting attention to alternative feedstocks that promise more sustainable paths for urea manufacturing. For instance, using bio-based materials instead of conventional fossil fuels could minimize dependence on finite resources while contributing positively to environmental sustainability.
The pursuit of these innovations could reshape not just urea manufacturing, but the broader chemical industries as well, driving a shift toward processes that embrace environmental stewardship.
Sustainability and Green Chemistry Initiatives
A pivotal shift in the industry is the emphasis on sustainability and green chemistry.
- Waste Minimization: Many urea producers are adopting practices that aim at waste reduction throughout their manufacturing processes. By minimizing waste, the overall efficiency improves, subsequently reducing cost and environmental impact. Implementing closed-loop systems is gaining traction, where waste materials are reused or repurposed within the production circuit.
- Green Catalysts: The use of catalysts is central to chemical processes, including urea manufacturing. Researchers are tirelessly working on greener catalysts that can enhance the production process while reducing toxic byproduct formation. These catalysts can lead to lower energy consumption and fewer resources used, aligning closely with the principles of green chemistry.
- Sustainable Agriculture: Lastly, as urea is primarily used in fertilizers, the push for sustainable agricultural practices is impacting the direction of urea manufacturing. This could mean developing urea products tailored to minimize runoff and enhance nutrient absorption by plants. Collaborations with agronomists are key to understanding how urea can be utilized more effectively in farming practices, ensuring that its application contributes to sustainable food production.
"Understanding and improving urea production processes is not solely an engineering challenge, but an essential pathway to sustainable agricultural and chemical practices."