Understanding Timber Strength: A Comprehensive Analysis
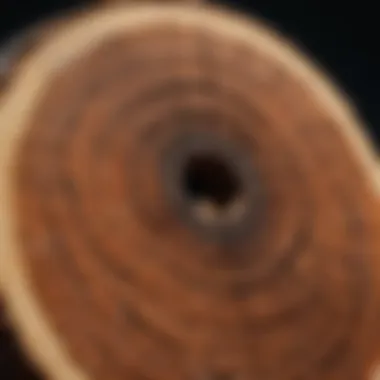
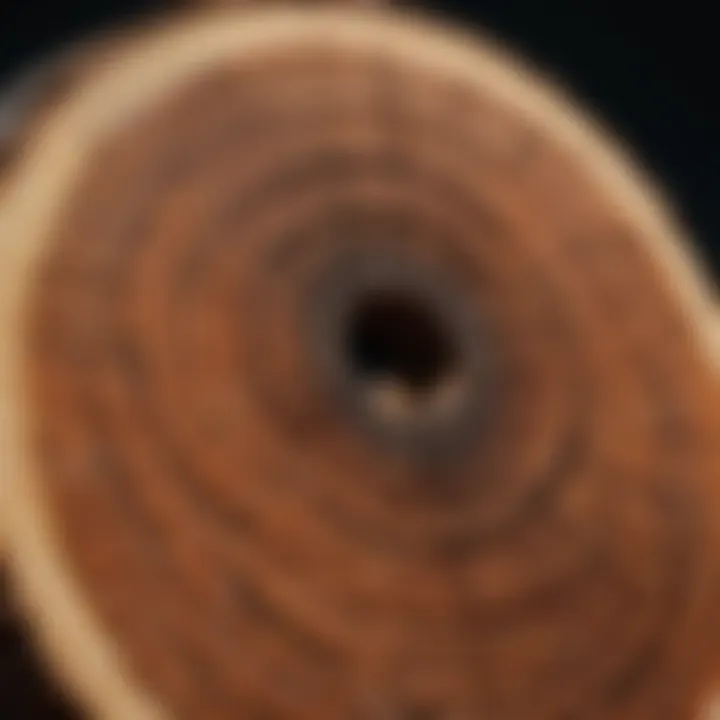
Intro
Timber is a vital material in various industries, particularly in construction and manufacturing. Understanding the strength of timber involves exploring multiple elements such as its biological composition, mechanical properties, and the influence of environmental factors. This article seeks to provide a thorough examination of timber strength, addressing the significance of different wood species, various treatments, and their impacts on performance. It is crucial for students, researchers, and professionals to grasp these concepts to improve the effective and sustainable use of timber.
Research Overview
Summary of Key Findings
Timber strength is influenced by biological factors such as growth habits and cellular structure. Different species exhibit various strength characteristics. Hardwoods like oak and maple tend to show higher strength than softwoods like pine and spruce. Furthermore, the environment in which timber grows plays a significant role, as factors like soil quality and climate affect growth rates and density.
Treatment processes such as drying and chemical preservation also enhance timber durability and strength. Each treatment process alters the physical properties, allowing timber to be utilized in areas requiring enhanced strength under specific conditions.
Methodologies Employed
This research utilized a combination of experimental testing and literature review. Mechanical strength tests, including tension, compression, and shear tests, were performed on various timber samples. Additionally, comparative analyses with existing studies helped identify trends and discrepancies across timber species.
In-Depth Analysis
Detailed Examination of Results
Analysis of the collected data illuminates key aspects of timber strength. For instance, basis weight and modulus of elasticity are fundamental properties that determine how well timber can withstand stress. Aspects such as grain orientation and knot presence also significantly influence strength.
- Hardwood Characteristics
- Softwood Characteristics
- Higher density contributes to greater strength.
- Less prone to warping compared to softwoods.
- Generally lighter and easier to work with.
- Susceptible to more bending under load.
"The strength of timber is not just a product of its physical attributes, but rather an interplay of numerous biological and environmental factors."
Comparison with Previous Studies
Prior studies have laid a foundation for understanding timber's mechanical properties. For instance, early research indicated significant discrepancies between hardwood and softwood performance. Recent findings, however, offer a more nuanced view, suggesting that treatment methods can improve the resilience of weaker timber species. This evolving understanding is essential for those engaged in timber-related fields.
End
Grasping the complexities around timber strength is pivotal for effective application in various sectors. As professionals and educators gain insights into the biological and mechanical nuances of timber, they can better approach sustainable practices and applications.
Prolusion to Timber Strength
Timber strength is a critical aspect of material science, especially in fields such as construction and manufacturing. Understanding timber's properties not only ensures structural integrity but also influences design choices and sustainability practices. This introduction will navigate the core elements that define timber strength as well as its significance in various relevant industries.
Recognizing what timber strength encompasses allows professionals to make informed decisions about its application. Timber strength is influenced by various factors including species, growth conditions, and the processing techniques used. Each of these aspects plays a vital role in determining how timber performs under different stresses and environments.
Definition of Timber Strength
Timber strength refers to the ability of wood to withstand load without failing. It encompasses various properties such as compressive strength, tensile strength, and bending strength. These properties are essential for predicting how timber will behave in structural applications. Each type of wood has its own unique strength characteristics based on its density, moisture content, and cellular structure.
Timber strength is typically measured in units like pounds per square inch (psi) or megapascals (MPa). The strength ratings of specific wood species can often be found in resources such as the American Wood Council. Understanding these measurements is key for engineers and architects as they select materials for construction and design.
Importance of Timber in Various Industries
Timber has diverse applications across several industries, making it an invaluable resource. In construction, timber is prized for its favorable strength-to-weight ratio, allowing for both structural and aesthetic advantages. Its natural insulating properties also contribute positively to energy efficiency in buildings.
- Construction Industry: Timber remains a preferred material for framing, flooring, and roofing. Its ease of use allows for quick assembly and versatility in designs.
- Furniture Manufacturing: The aesthetic appeal and strength of timber make it an ideal choice for producing quality furniture. Different species offer varying grains and colors, enhancing marketability.
- Paper and Pulp: Timber is also essential in the paper industry, serving as a primary raw material for producing paper products.
Biological Composition of Timber
The biological composition of timber plays a crucial role in determining its strength and functionality. Understanding the cellular and chemical makeup can provide insights into the performance characteristics of various timber species. Each timber type exhibits unique properties based on its biological structure, which is essential for applications in construction, manufacturing, and design.
The primary components of timber include cellulose, hemicellulose, and lignin. These elements not only define the physical attributes of wood but also contribute significantly to its mechanical properties, such as stiffness, tensile strength, and durability. This section explores the cellular structure of wood and the specific roles of lignin and cellulose in influencing timber strength.
Cellular Structure of Wood
Wood is a complex material composed of cells that create a matrix essential for its structural integrity. The cellular structure of wood includes several types of cells, such as tracheids, vessels, fibers, and parenchyma cells. Each cell type has a specific function:
- Tracheids: These are long, narrow cells that are responsible for water conduction and provide mechanical support. They are present in both hardwoods and softwoods.
- Vessels: Mainly found in hardwoods, vessels facilitate efficient water transport.
- Fibers: These cells contribute to the tensile strength of wood, providing rigidity and resistance to bending.
- Parenchyma cells: They serve metabolic functions and help in storage, although they contribute less to structural strength.
The arrangement and density of these cells influence the overall strength of the timber. Higher density woods, such as oak, generally exhibit superior strength compared to lower density species. This is crucial in applications where strength-to-weight ratio is critical.
Role of Lignin and Cellulose
Lignin and cellulose are the two primary organic compounds in wood that influence its mechanical properties.
- Lignin: This complex polymer provides rigidity and compressive strength to wood. It acts as a binding agent that helps secure the cellulose fibers together, enhancing the overall durability of the timber. Lignin's presence is vital for the structural integrity of wood, particularly in harsh environments where resistance to decay and environmental stress is necessary.
- Cellulose: Composed of long chains of glucose, cellulose is highly fibrous. It is responsible for the majority of wood's tensile strength and stiffness. This biopolymer allows wood to resist stretching and provides the structural support needed in various applications.
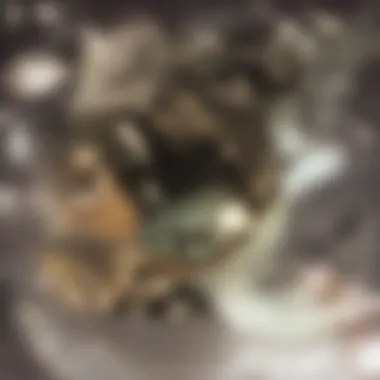
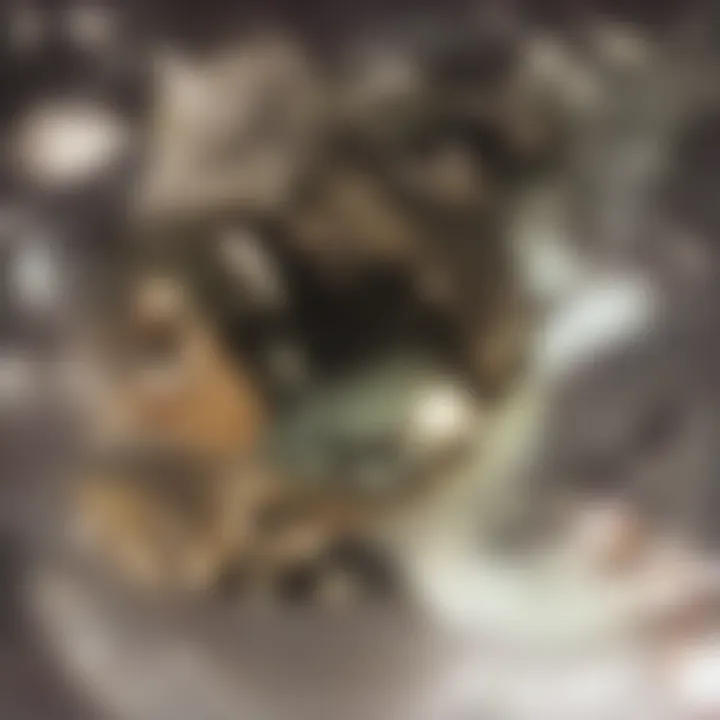
In summary, the synergy between lignin and cellulose shapes the mechanical properties of timber significantly. A well-balanced composition aids in achieving optimal strength characteristics while supporting wood's function in a variety of settings. Understanding these components can guide better selection of timber types for specific uses in construction and design, ensuring performance and longevity of projects.
"The ability to identify the specific strengths derived from wood's biological composition can enhance material choice in construction, promoting sustainability and efficiency."
Careful analysis of these biological aspects provides the foundation for applied research in timber use, making it an invaluable focus for educators and professionals in related fields.
Mechanical Properties of Timber
The mechanical properties of timber are central to its usability and effectiveness in various applications. Understanding these properties enables professionals to make informed decisions in design, construction, and utilization of wood as a structural material. Timber serves as a preferred choice in many structures because of its unique combination of strength, flexibility, and environmental benefits. Therefore, grasping the nuances of timber's mechanical properties is essential for students, researchers, and professionals alike.
Compressive Strength
Compressive strength refers to the ability of timber to withstand axial loads that compress its fibers. This property is critical for structural elements such as beams and columns that bear significant weight. In timber, compressive strength can vary significantly depending on factors such as wood species, grain orientation, and moisture content.
When selecting timber for structural purposes, it is important to know the compressive strength ratings of the specific species being used. Generally, dense hardwoods exhibit higher compressive strength compared to softer woods. For instance, Oak typically has greater compressive strength than Pine. The presence of defects, such as knots or splits, may adversely affect the compressive strength of timber, leading to potential failure under load.
Tensile Strength
Tensile strength measures how well timber can resist stretching or pulling forces. While timber is not primarily designed to withstand tension compared to compression, its tensile strength is still an important consideration in construction. This characteristic is particularly relevant in situations where wood elements experience bending or when used in tension trusses.
Wood retains more tensile strength when its fibers are aligned with the direction of the load. The orientation and condition of the timber can also affect this property. For example, laminated veneer lumber tends to perform better in tensile strength than solid wood beams due to its engineered nature, making it a suitable choice for applications requiring significant tensile capacity.
Bending Strength
Bending strength, or flexural strength, is the property of timber that defines its ability to resist bending forces. This is essential in applications where timber supports loads across spans, such as in flooring and roofing applications. The bending strength of timber indicates how well it can withstand a moment created by forces acting perpendicular to its length.
Specific species, like Ash and Maple, demonstrate higher bending strength, making them ideal for load-bearing applications. Factors affecting bending strength include the ratio of height to width of the timber, the presence of defects, and the type of loading applied. Proper testing, such as bending tests, helps establish the materialβs capacity to resist bending.
Understanding these mechanical properties allows for more sustainable use of timber resources by ensuring that only appropriately rated woods are applied in necessary contexts.
Moreover, engineers and architects should consider these properties when designing structures that require load-bearing elements. Knowledge of compressive, tensile, and bending strengths is invaluable for optimizing performance while minimizing timber waste.
Variations in Timber Strength by Species
Understanding the variations in timber strength by species is essential in many fields such as construction, furniture making, and woodworking. Each species of timber exhibits unique properties that affect its strength and suitability for different applications. Factors influencing these differences include the speciesβ growth environment, its biological makeup, and how it responds to various treatment methods. Recognizing these variations not only allows professionals to select the right type of wood for their projects but also promotes sustainable practices by effectively utilizing the available timber resources.
Hardwoods vs. Softwoods
When discussing timber strength, the distinction between hardwoods and softwoods is fundamental. Hardwoods come from angiosperms, which are trees that have broad leaves and generally produce seeds with a covering. Examples include Oak, Maple, and Cherry. These woods typically have a denser structure, leading to higher strength values, particularly in terms of bending and compression.
On the contrary, softwoods originate from gymnosperms, trees that bear needles and cones, such as Pine, Spruce, and Cedar. Generally, softwoods are lighter and more workable, making them popular for building frameworks and furniture.
- Hardwoods:
- Softwoods:
- Higher density
- Greater strength
- Better suited for structural applications
- Lower density
- Easier to cut and shape
- Commonly used in construction and packaging
This elemental difference significantly influences their applications. Hardwoods are often favored for flooring and cabinetry, while softwoods are frequently utilized in framing and roofing.
Notable Species and Their Strength Characteristics
Numerous species have specific strength characteristics that make them distinctive. For example:
- Oak: Known for its durability and resistance to warping, oak timber is a preferred choice in high-stress applications such as beams and heavy furniture. Its overall strength makes it favorable for both outdoor and indoor use.
- Maple: This hardwood possesses a tight grain pattern that contributes to its strength. It is ideal for items that endure significant use, like countertops and flooring.
- Douglas Fir: As a softwood, Douglas Fir provides exceptional stiffness and strength. Itβs common in construction for beams and frames due to its reliable performance under load.
- Teak: This hardwood is renowned not only for its strength but also resistance to moisture, making it suitable for marine applications and outdoor furniture.
- Balsa: Notably one of the softest woods, balsa is lightweight and used mainly for model making and crafts rather than structural applications.
In summary, the strength of timber varies significantly across species. Understanding these differences can help in making informed choices in a variety of applications, from construction to fine woodworking. These insights are crucial for those in the industry and serve to enhance the effective use of timber as a renewable resource.
Factors Influencing Timber Strength
Understanding the factors that influence timber strength is essential for maximizing its applications and ensuring its longevity in various constructions. Timber is a natural material that displays distinct strengths and weaknesses based on a variety of influences. These influences can be broadly categorized into environmental conditions, moisture content, and the aging process of the timber.
By recognizing these factors, professionals can make informed decisions regarding timber utilization. This ensures that both the structural integrity and sustainability are maintained throughout the lifecycle of timber, from harvesting to final installation.
Environmental Influences
Environmental influences on timber strength are significant and can affect the performance of wood in practical applications. Various factors in the natural environment, such as temperature, humidity, and exposure to sunlight, can alter the physical properties of timber. For instance, trees grow in diverse climates, and their genetic makeup adapts to those conditions.
A key aspect to consider is the location of timber growth:
- Soil Composition: Timber from nutrient-rich soils tends to have better density and strength.
- Climate Factors: Trees in harsh climates may develop stronger wood due to slower growth rates, improving density.
- Exposure to Elements: Wood exposed excessively to moisture or sunlight may weaken over time due to decay or thermal degradation.
Understanding these environmental factors can guide choices in sourcing timber for specific projects.
Moisture Content and Its Impact
Moisture content is one of the most critical determinants of timber strength. The presence of water in wood can affect its weight, durability, and mechanical properties. Typically, timber is categorized as green (freshly cut) or seasoned (dried), and this distinction plays a significant role in its strength.
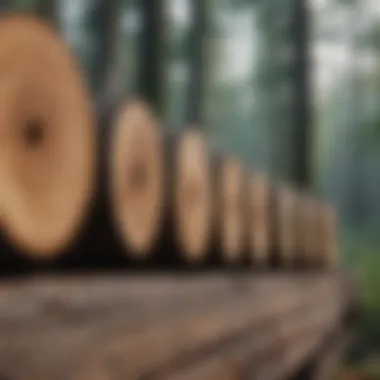
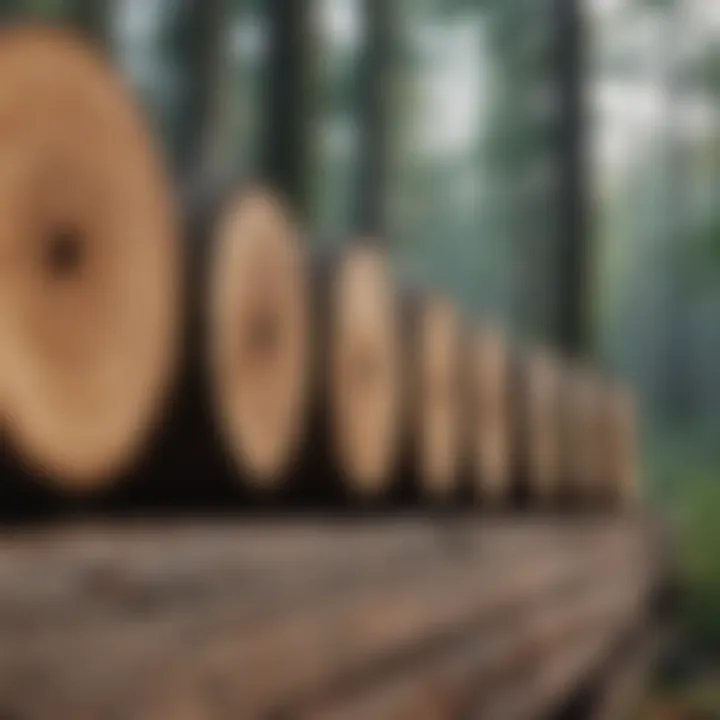
High moisture content increases the weight of timber, making it more susceptible to various forms of damage, including fungal decay and insect infestation. On the other hand, low moisture content increases the potential for wood to splinter or crack.
- Optimal Moisture Levels: For most structural applications, a moisture content of around 12-15% is considered ideal.
- Impact on Strength: Higher moisture levels can lead to reduced compressive and tensile strength, impacting the overall stability of timber constructions.
Proper seasoning techniques must be employed to control moisture content and ensure timber reaches the desired durability and performance before installation.
Aging and Natural Defects
Aging also impacts timber strength, manifesting in various ways throughout its lifecycle. Over time, timber can develop natural defects, which may hamper its ability to withstand loads effectively.
Some common defects include:
- Knots: These can create weak points in timber.
- Splits and Cracks: Aging can lead to the formation of fissures that compromise strength.
- Warping: Changes in moisture content can lead to distortion, affecting how timber interacts with other materials.
Natural aging processes must be considered when assessing the strength of timber. While some defects can diminish structural integrity, a well-maintained aging process can enhance certain characteristics, such as stability and resistance.
Processing and Treatment Methods
The processing and treatment of timber are vital to its overall strength and usability. These methods not only enhance the physical characteristics of the wood but also prolong its longevity and resistance to various forms of degradation. Understanding these processes is essential for anyone involved in timber utilization, particularly those in construction, carpentry, and material science. This section will cover seasoning of timber, preservative treatments, and mechanical strengthening techniques, illustrating their significance and applications.
Seasoning of Timber
Seasoning refers to the process of reducing the moisture content of timber before it is used in construction or manufacturing. Proper seasoning is crucial as it affects both the strength and dimensional stability of wood. There are two main methods of seasoning: air seasoning and kiln drying.
- Air Seasoning: This method relies on natural air movement to reduce moisture content. It is cost-effective but can take several months. The success of air seasoning largely depends on climate conditions and requires careful stacking of timber to avoid warping and fungal growth.
- Kiln Drying: This controlled method uses heat to accelerate the drying process. While it is faster than air seasoning, it demands careful regulation of temperature and humidity. Kiln drying can significantly improve the strength characteristics of timber by ensuring a uniform moisture content.
A well-seasoned timber provides minimal shrinkage, helping to maintain structural integrity. The benefits of proper seasoning include enhanced dimensional stability and reduced risk of defects such as splits and cracks.
Preservative Treatments
Preservative treatments are employed to protect timber from biological threats such as fungi and insects. The use of preservatives is essential in environments where timber is exposed to moisture or soil contact. Common preservative methods include:
- Pressure Treatment: A widely used method that forces preservatives into the wood under high pressure. This ensures deep penetration and long-lasting protection. Common preservatives include copper-based compounds, which are effective against wood rot and insect infestation.
- Surface Treatments: This includes paints, varnishes, and sealants that provide a barrier against moisture and pests. While surface treatments are easier to apply, they may not penetrate the wood deeply, thus requiring more frequent reapplication.
These treatments not only enhance the durability of timber but also extend its service life, making it an essential process in timber processing. The choice of preservative should consider the specific application and environmental conditions.
Mechanical Strengthening Techniques
Mechanical strengthening techniques enhance the inherent strength of timber, making it suitable for various structural applications. These methods can significantly influence a timber productβs load-bearing capacity. Some notable techniques include:
- Laminated Veneer Lumber (LVL): This process involves gluing together thin layers of wood veneers. LVL products are known for their consistent quality and enhanced strength compared to solid lumber.
- Glue-Laminated Timber (Glulam): Similar to LVL, glulam is formed by bonding together layers of dimensional lumber with durable adhesives. This results in a product that can achieve larger spans than traditional timber, making it popular in large-scale construction projects.
- Reinforcement with Steel or Fiberglass: In some cases, timber can be reinforced with steel plates or fiberglass rods to enhance its strength. This is particularly useful in areas where heavy loads are anticipated.
The application of these mechanical techniques allows for more innovative designs and applications in construction and architecture, illustrating the versatility of timber as a building material.
"Processing and treatment methods not only improve the strength of timber but also ensure it meets the demands of modern construction standards."
Through these methods, timber can be transformed into a stronger and more durable material, suited for various applications across multiple industries. For further reading on timber processing methods, you can refer to Wikipedia.
Testing Methods for Timber Strength
Understanding the strength of timber is not only crucial for those in construction and engineering but also for preserving its integrity in various applications. Testing methods for timber strength serve as the backbone of this understanding. By employing these methods, professionals can evaluate the performance of timber products under different stressors. This section will elaborate on these testing methods, focusing on their benefits, considerations, and specific procedures employed in both static and dynamic assessments.
Static Testing Procedures
Static testing is a fundamental method for assessing timber strength. This technique involves applying a gradually increasing load to a timber sample until failure occurs. The main goal is to gauge key properties such as bending strength, compressive strength, and shear strength.
The procedure generally includes the following steps:
- Sample Preparation: Timber samples should be uniform and free of defects. Proper preparation is essential to ensure valid results.
- Equipment Setup: A testing machine, typically a universal testing machine, is equipped with load cells to accurately measure the applied force.
- Loading Process: Load is applied slowly, allowing for observation of deformation. Generally, the load is increased until the material fails, displaying breakage or significant deformation.
- Data Collection: As data is recorded, important metrics such as modulus of elasticity and ultimate strength are determined.
One of the main advantages of static testing is its straightforward execution and reliable results. However, it may not fully represent real-world conditions where dynamic forces are at play. This limitation highlights the importance of supplementing static tests with dynamic evaluations.
Dynamic Testing Techniques
Dynamic testing methods assess the response of timber under varying loads over time. Unlike static testing, dynamic tests are designed to simulate conditions that timber may encounter throughout its service life.
Key characteristics of dynamic testing include:
- Impact Loading: The method typically involves subjecting wood samples to sudden loads, such as impacts or vibrations. This mimics real-life scenarios such as moving vehicles on wooden bridges.
- Frequency and Damping: Engineers analyze the frequency response of timber, focusing on how it absorbs and dissipates energy. Damping ratios can be critical in assessing the ability of timber to withstand seismic events.
- Equipment Usage: Specialized equipment like vibration testing machines or drop weights is often used to create accurate test conditions.
Dynamic testing provides insights into performance characteristics that static methods cannot reveal. Understanding how timber behaves under fluctuating loads can guide effective application in construction. However, this method can be more complex and requires a robust understanding of material dynamics, reinforcing the necessity for trained personnel in execution.
"Timber's response to dynamic loads is crucial for structures expected to experience various stressors over their lifespan."
In summary, the testing methods for timber strength, encompassing both static and dynamic procedures, are essential for guaranteeing the material's performance. These techniques empower professionals to make informed decisions about timber use in diverse applications, ensuring both safety and durability in construction.
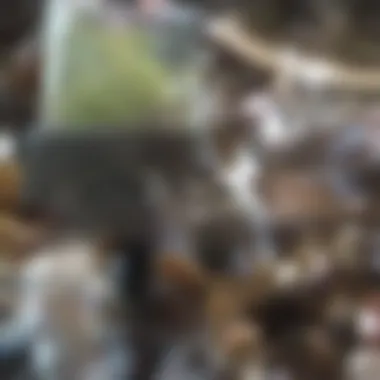
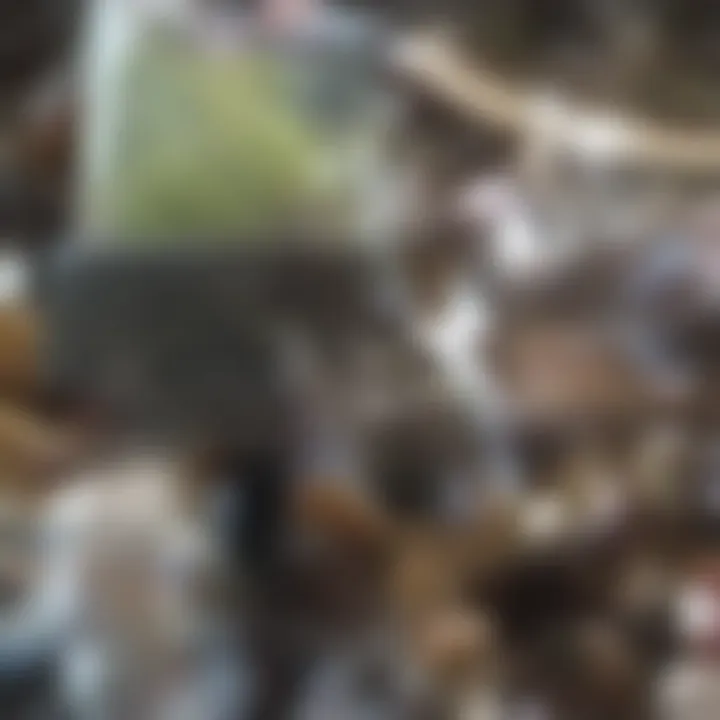
Applications of Timber Strength in the Construction Industry
Timber strength is a critical consideration in the construction industry. It directly influences the design and safety of structures. Understanding how timber performs under various conditions allows architects, engineers, and builders to make informed decisions. Moreover, the properties of timber can offer both aesthetic and functional benefits in several applications.
The ability to assess timber strength ensures materials meet necessary safety standards. It is essential not just for compliance but also for sustainability. Responsible usage of timber not only optimizes resources but also minimizes waste. Given the global push towards more sustainable building practices, the strength characteristics of timber are of increasing relevance.
Structural Applications
Structural applications of timber play a fundamental role in construction. Timber serves as a key material for framing and support systems in residential and commercial buildings. Its favorable strength-to-weight ratio makes it ideal for load-bearing structures, such as walls, roofs, and floors.
In addition to load-bearing, timber is utilized in various forms such as glulam and laminated veneer lumber. These engineered wood products enhance strength and durability. They provide flexibility in design while maintaining structural integrity. Structural elements such as beams and columns made from engineered timber can carry significant loads, contributing to the overall safety of a building.
Some important factors to consider in structural applications include:
- Species Selection: Different wood species have varying strength characteristics. For example, Douglas Fir is selected for its high strength, while Pine is often used for its ease of workability.
- Load Types: Understanding the nature of loads (static versus dynamic) on timber structures is crucial.
Non-Structural Uses
Non-structural uses of timber in construction also present significant advantages. Timber is employed for aesthetic enhancements, interior finishing, and space partitioning. The warmth and natural beauty of wood can elevate the ambiance of a space.
Additionally, timber's versatility allows for its use in furniture, fixtures, and decorative elements. These applications require a different focus on strength, often prioritizing appearance over load-bearing capabilities.
Some common non-structural applications include:
- Flooring: Engineered flooring products offer durability while maintaining aesthetic appeal.
- Wall Paneling: Timber is widely used for paneling due to its attractive finish and insulation properties.
- Furniture: The choice of timber for furniture is often based on aesthetics and ergonomics, albeit strength still plays a role in ensuring longevity.
Timber's strength, combined with its visual appeal, has cemented its place in both structural and non-structural applications. As the construction industry evolves, leveraging the strength of timber becomes more significant.
"An informed understanding of timber strength enhances sustainability and safety in construction."
By focusing on both the structural and non-structural uses of timber, professionals can grasp the multifaceted role this resource plays in modern architecture and design.
Future Trends in Timber Usage
Timber is not just a traditional building material; it is evolving with the times. Understanding future trends in timber usage is crucial for ensuring sustainable practices and enhancing the material's potential in various industries. This section will explore the significance of sustainability in timber sourcing and the latest innovations aimed at boosting timber strength.
Sustainability in Timber Sourcing
Sustainability in timber sourcing involves managed and environmentally responsible practices to ensure that timber is harvested without compromising ecosystem health. Sustainable timber sourcing is critical because of the increasing deforestation and its impacts on climate change. By selecting timber from certified sources like the Forest Stewardship Council, industries can make a positive impact.
The benefits of sustainable sourcing include:
- Conservation of Biodiversity: Protects diverse habitats and species.
- Reduction of Carbon Footprint: Sustainable practices contribute to lowering emissions by maintaining forests.
- Economic Benefits: Helps local economies thrive by creating job opportunities in sustainable timber harvesting.
- Quality Assurance: Sustainably sourced timber often comes with certifications that indicate quality and durability.
By focusing on sustainability, industries contribute positively to the planet while ensuring that timber remains a viable resource for the future. This not only complies with regulations but also aligns with the growing consumer demand for eco-friendly products.
Innovations in Timber Strength Enhancement
The landscape of timber strength can be transformed through various innovative methods. These advancements are critical, given that stronger timber can offer improved performance in construction and other applications. Some notable innovations include engineered wood products like laminated veneer lumber and cross-laminated timber.
The importance of innovations in timber strength includes:
- Enhanced Load-Bearing Capacity: Engineered timber can bear significantly more weight than traditional timber.
- Design Flexibility: New products allow for more creative architectural designs, leading to lighter and more efficient structures.
- Sustainability: Some of these innovations utilize lower-quality timber, making effective use of all available resources and reducing waste.
- Resilience: Improved treatment processes can make timber less susceptible to moisture and pests.
In summary, future trends in timber usage are centered on sustainability and innovations aimed at enhancing strength. These trends are vital for the timber industry, serving to address environmental concerns while pushing the boundaries of what timber can achieve in construction and beyond.
"As the world moves towards more sustainable practices, the timber industry must innovate to meet the challenges of the future while preserving our natural resources."
Through these efforts, professionals, researchers, and students can engage with timber not just as a material, but as a pivotal component in future sustainable development.
Finale and Implications for Research
Understanding timber strength is vital not just for professionals in construction and material sciences, but also for researchers who delve into sustainable practices. This article has explored various aspects of timber strength, shedding light on biological composition, mechanical properties, environmental influences, and treatment methods.
One of the key elements of this discussion has been the interrelationship between timberβs inherent properties and its applications across different industries. Timber serves as a versatile material, integrating into diverse structures and products. A deep dive into its strength characteristics informs better design choices, enhances durability, and supports sustainability goals.
In future research, there are multiple considerations. It's important to evaluate how advancements in technology and engineering can further exploit timber's potential. For instance, exploring modern composite materials that incorporate timber could lead to enhanced properties and applications. Similarly, testing under varying environmental conditions can yield insights pertinent to climate resilience.
The multifaceted study of timber strength paves the way for innovation in both architecture and environmental sustainability.
Moreover, understanding the interaction of timber strength with biological and chemical treatments opens avenues for developing more effective preservation methods, reducing waste, and promoting responsible sourcing practices. This interconnected knowledge aids in establishing industry standards that ensure safety and effectiveness in timber use.
Summary of Key Findings
- Biological Composition: The cellular structure of timber, primarily composed of lignin and cellulose, plays a crucial role in defining strength characteristics. These components interact to provide unique mechanical properties.
- Mechanical Properties: Timber exhibits varied compressive, tensile, and bending strengths depending on species and environmental factors. Hardwoods generally display greater strength than softwoods, yet both types offer valuable properties suitable for specific applications.
- Environmental Influences: Factors like moisture content, aging, and natural defects considerably affect timber strength. Comprehensive understanding of these elements is critical in construction applications.
- Processing Methods: Proper seasoning and treatment enhance timberβs durability and strength. Advances in mechanical strengthening techniques are also worth noting as they can further optimize timber usage in structural applications.
- Future Trends: A growing focus on sustainable timber sourcing reflects broader ecological concerns. Innovations in strength enhancement technologies and practices are likely to redefine traditional applications and open new markets.
Future Research Directions
Future research should prioritize several important directions:
- Sustainability and Eco-efficiency: Investigating sustainable forestry practices and selecting species based on growth rates and ecological impacts can improve timber sourcing.
- Material Innovations: Exploring hybrid materials that combine timber with other substances might yield stronger, lightweight alternatives suitable for modern construction needs.
- Testing Methodologies: Developing advanced testing methods that simulate real-world conditions can provide critical insights into timber performance over time. This includes dynamic testing that reflects current environmental challenges.
- Long-Term Studies: Longitudinal studies that track timber performance in aging infrastructure can reveal the longevity and durability of wood-based structures under varying conditions.
Adopting a multifaceted research approach encourages an in-depth analysis of timber, ultimately contributing to more sustainable practices and a better understanding of its essential role in various industries.