Exploring SYBR Green I in Molecular Biology
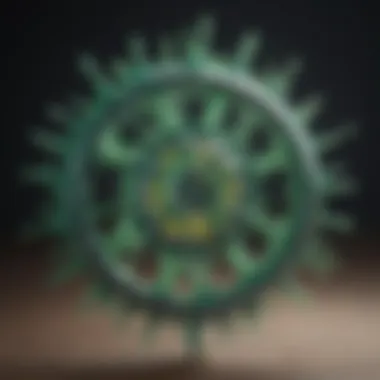
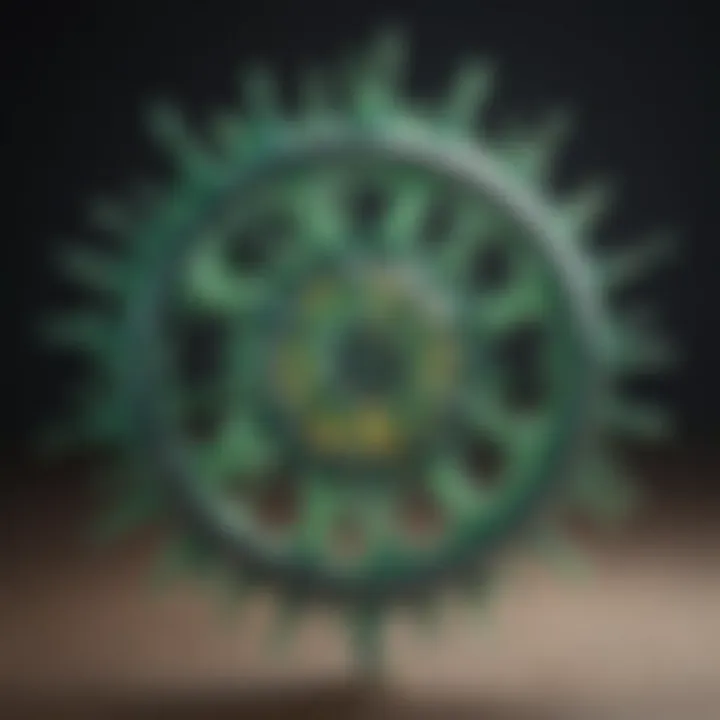
Intro
SYBR Green I represents a pivotal tool in molecular biology, specifically in the domain of nucleic acid quantification. By leveraging real-time polymerase chain reaction (PCR) technology, this fluorescent dye facilitates accurate, rapid, and sensitive analysis of DNA and RNA. Understanding its role extends beyond mere application; it involves grasping the mechanisms, optimizing protocols, and recognizing the limitations inherent in its use. As the scientific community continuously seeks advancements in nucleic acid detection technologies, a thorough examination of SYBR Green I illuminates both its practicality in experiments and its impacts on research outcomes.
Research Overview
Summary of Key Findings
The investigation into SYBR Green I has yielded compelling insights. Firstly, its mechanism is straightforward yet effective, binding to double-stranded DNA (dsDNA) and exhibiting strong fluorescence upon excitation. This makes it particularly suited for quantitative analysis. Secondly, practical applications of SYBR Green I encompass various fields, from clinical diagnostics to genetic research, showcasing its versatility. However, some limitations have been identified, notably its tendency to bind to non-specific products, which can compromise data integrity.
- Quantification: Accurate measurement of nucleic acid concentrations.
- Sensitivity: Detects low-abundance targets effectively.
- Limitations: Non-specific amplification can lead to misleading results.
Methodologies Employed
To gather relevant data, researchers have employed a variety of methodologies:
- Real-Time PCR: This is the gold standard for utilizing SYBR Green I. By monitoring fluorescence intensity in real-time, quantification of nucleic acids becomes possible.
- Comparative Studies: Scientists compare SYBR Green I with other dyes such as EvaGreen or probe-based assays like TaqMan to evaluate performance differences.
- Optimization Studies: Investigating factors such as primer design, annealing temperatures, and the dye’s concentration allows for improved results and reliability.
In-Depth Analysis
Detailed Examination of Results
Each study reviewing SYBR Green I emphasizes its efficacy in quantifying nucleic acids. It consistently demonstrates high sensitivity, showing the ability to detect targets even at picogram levels. For instance, in one significant research, SYBR Green I was utilized to quantify expression levels of target genes in a clinical setting, yielding precise results crucial for diagnostic purposes.
Moreover, the flexibility of SYBR Green I allows for multiplexing applications, where multiple targets are quantified within a single reaction. This has profound implications for studies interested in simultaneous monitoring of various genes or pathogens.
Comparison with Previous Studies
Previously, many researchers relied on traditional methods such as gel electrophoresis for nucleic acid quantification, which, while effective, are labor-intensive and time-consuming. In a study by Wang et al. (2022), it was noted that SYBR Green I outperformed these methods by providing real-time data that not only reduced experimental time but also increased throughput. This is paramount for high-stakes research demands in numerous sectors including biotechnology and clinical lab settings.
Prologue to SYBR Green
In the realm of molecular biology, the quest for precision and efficiency in nucleic acid quantification remains paramount. SYBR Green I emerges as a significant ally in this endeavor. This section dissects the essence of SYBR Green I, laying the groundwork for understanding its multifaceted applications. By appreciating the intricacies of this dye, researchers can leverage its potential to elevate their work, whether it's in the throes of lab-based experimentation or the broader applications in clinical diagnostics.
The significance of introducing SYBR Green I lies in its role as a benchmark for various nucleic acid detection methods. Its versatility informs a vast range of practices—be it in academia, research institutions, or even upon the commercial stage. The upcoming discussion is aimed not just at elucidating the properties of SYBR Green I but also shedding light on the nuances that researchers must consider when employing it in their experiments. This includes an in-depth look into its fundamental characteristics and the historical context that have shaped its utility.
What is SYBR Green ?
SYBR Green I is a fluorescent dye specifically designed for nucleic acid detection. Primarily, it binds to double-stranded DNA, emitting fluorescence when exposed to light at a particular wavelength. This characteristic makes it exceptionally useful for real-time polymerase chain reaction (PCR) applications, where monitoring DNA amplification in real time is crucial. Because it interacts with any double-stranded DNA, its practicality is broad, albeit with certain caveats.
The dye operates based on an intercalation mechanism, where it inserts itself between the base pairs of the DNA helix. Upon binding, its conformation changes, leading to enhanced fluorescence. In simpler terms, it acts somewhat like a spotlight, illuminating the presence of DNA as amplification progresses during PCR cycling.
This capacity to emit light allows researchers to gauge the amount of DNA present in a sample, ultimately providing insight into gene expression, genotyping, or the detection of specific pathogens. SYBR Green I’s effectiveness relies heavily on proper optimization in experimental design, underscoring the importance of understanding the intricacies of its application.
History and Development
The evolution of SYBR Green I speaks to the advancement in molecular diagnostic technologies over the years. Developed in the late 1980s and early 1990s, the dye was part of a larger wave of innovations aimed at improving gel electrophoresis methods. Back then, scientists were grappling with challenges in sensitivity and specificity in nucleic acids analysis. Traditional methods often required laborious processes with limited quantifiable results.
SYBR Green I was a game changer, offering a more straightforward solution for the quantification of nucleic acids without the need for complex procedures. It quickly established itself as a favorite due to its user-friendly nature and good performance across diverse applications.
The dye's compatibility with different instruments also contributed to its rapid adoption. As PCR technology gained traction, SYBR Green I emerged as a staple in labs worldwide. Many consider its reliability and ease of use as key factors, propelling it to the forefront of fluorescent dyes in molecular biology.
As a critical tool in the toolkit of researchers, the continued evaluation of SYBR Green I's performance against its limitations is essential as innovation keeps pace with the ever-evolving landscape of biological research.
"SYBR Green I has transformed the way we approach nucleic acid quantification, paving the way for advancements in research and diagnostics."
Understanding the landscape of SYBR Green I sets the stage for a closer examination of its mechanisms, applications, effectiveness, and challenges, ensuring informed decisions in its usage moving forward.
Mechanism of Action
Understanding the mechanism of action behind SYBR Green I is crucial not just from a theoretical standpoint, but also for its practical usage in day-to-day molecular biology experiments. At its core, the mechanism illustrates how this fluorescent dye effectively binds to nucleic acids. This binding is the foundation for measurements that researchers take during processes like real-time PCR, making it an important aspect of the fluorescence detection capabilities of SYBR Green I. Knowing the ins and outs of this mechanism also helps scientists devise better protocols and troubleshoot issues should they arise. Here we unpack its two main components: intercalation with nucleic acids and fluorescence emission characteristics.
Intercalation with Nucleic Acids
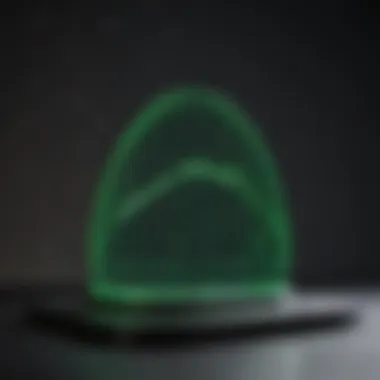
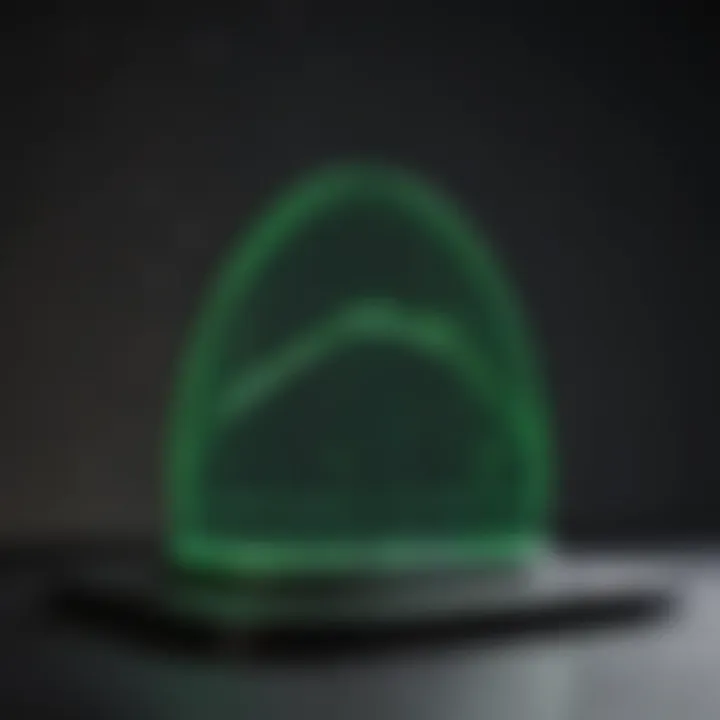
SYBR Green I operates primarily through a process called intercalation, where the dye physically inserts itself between the bases of DNA or RNA. This action enhances the fluorescence signals during nucleic acid amplification and provides a reliable means for quantification. Think of it as providing the "glue" that allows the detection system to catch hold of the genetic material being analyzed.
Key points to consider regarding intercalation include:
- Selective Binding: The target nucleic acids generally have a specific affinity for SYBR Green I, thereby facilitating preferential binding to double-stranded forms. This selectivity underscores its capability for accurate detection.
- Concentration Matters: An optimal concentration of SYBR Green I is vital for efficient intercalation. Too little dye, and signals may be weak; too much, and you might introduce background fluorescence, complicating results.
- Temperature Sensitivity: The intercalation process can be temperature-dependent. Knowing this can be helpful for researchers to control the settings in real-time PCR to maximize accuracy.
This intercalation process not only amplifies the fluorescent signal from the target but also minimizes background noise. This gives researchers one less thing to worry about when seeking clearer, more understandable results.
Fluorescence Emission Characteristics
Once SYBR Green I is intercalated, the magic doesn’t stop there. The real show begins with fluorescence emission, which is central to its utilization in measurements. When exposed to light of a particular wavelength, the dye emits light that is detectable and proportional to the amount of nucleic acid present in the sample. It’s like flicking a light switch in a dark room; more lights mean more visibility.
There are several characteristics about fluorescence emissions to keep in mind:
- Excitation and Emission Wavelengths: SYBR Green I has specific wavelengths for excitation (around 497 nm) and for emission (about 520 nm). Understanding these wavelengths aids researchers in setting up their detection instruments.
- Quantifiable Signal: The intensity of the emitted light is directly proportional to the amount of nucleic acid present. This relationship allows for accurate quantification, enabling researchers to draw sound conclusions from their experimental data.
- Potential Interference: While fluorescence can enhance results, it can also create challenges. Other substances in the sample can emit similar wavelengths, leading to interference. Understanding these pitfalls can streamline experimental design.
Thus, familiarity with the fluorescence emission characteristics of SYBR Green I helps researchers discern not just the quantity of nucleic acids, but also provides insights into the quality and reliability of their results.
Understanding the mechanism of action behind SYBR Green I is pivotal for researchers aiming to utilize its full potential in nucleic acid quantification.
Practical Applications
The practical applications of SYBR Green I in molecular biology highlight its critical role in advancing research and diagnostics. Understanding how to effectively utilize this dye can be a game-changer for many scientists working with nucleic acids. The significance of SYBR Green I extends beyond just being a piece of equipment in a lab; rather, it serves as a transformative tool in various experimental settings. Here, we’ll explore a trio of principal applications: real-time PCR, quantitative analysis of DNA, and genotyping.
Real-Time PCR
Real-time polymerase chain reaction, commonly known as real-time PCR, has revolutionized how researchers quantify nucleic acids. This technique allows for the simultaneous amplification and monitoring of DNA, providing immediate feedback on amplification occurring in the reaction. SYBR Green I plays a pivotal role in this method by intercalating into double-stranded DNA, emitting fluorescence that corresponds to the quantity of DNA produced. Researchers gain the advantage of obtaining precise quantitative data with each cycle of PCR. When you consider the applications across various fields—medical diagnostics, environmental testing—real-time PCR’s ability to offer rapid results cannot be understated.
- Advantages of Real-Time PCR with SYBR Green I include:
- Fast and accurate quantification of DNA.
- High throughput capabilities for large sample sizes.
- Potential for multiplex applications, allowing analysis of multiple targets simultaneously.
Keeping in mind the importance of standardization during real-time PCR ensures reliable results, which is an aspect researchers must carefully calibrate.
Quantitative Analysis of DNA
Quantitative analysis of DNA with SYBR Green I delivers essential insights into the concentration and purity of nucleic acid samples. This approach is key in research settings where understanding specific DNA amounts is critical—for instance, in the assessment of gene expression levels, clinical diagnostics, or even environmental applications like biodiversity studies.
The ability to correlate fluorescence intensity directly with DNA concentration plays to the strengths of SYBR Green I. Researchers regularly employ standard curves to derive exact concentrations, which can significantly influence downstream applications like cloning or sequencing.
"The accurate quantification of DNA is fundamentally important for ensuring validity in molecular analyses."
- Factors impacting quantitative analysis include:
- Sample integrity and purity.
- PCR efficiency, which should be routinely assessed.
- Calibration against standards to establish metrics.
Such diligence allows for confidence in results, bridging the gap to more advanced molecular biology studies.
Applications in Genotyping
Genotyping involves identifying variations in genes and their roles in health and disease, and SYBR Green I emerges as a valuable ally in this domain. The dye's ability to fluoresce in the presence of amplified DNA enables the detection of polymorphisms and other genetic variations. This application is especially useful in population genetics, evolutionary studies, and personalized medicine.
Researchers often implement endpoint PCR utilizing SYBR Green I for genotyping, leveraging its efficiency to differentiate between amplified products based on melting temperature analysis.
- Key aspects include:
- Cost-effective method compared to probe-based genotyping.
- Simplicity in protocol, requiring less specialized equipment.
- Ability to assess multiple samples in a single run, improving throughput.
These factors collectively enhance the efficiency of genotyping applications, which are critical for mapping genetic landscapes and linking them to phenotypic outcomes.
In summary, the adaptability of SYBR Green I makes it indispensable in numerous practical applications within molecular biology. Understanding its capabilities and considerations can empower researchers to harness its full potential in their investigations.
Benefits of Using SYBR Green
When delving into the mechanics of molecular biology, the significance of choosing the appropriate reagents cannot be overstated. One such reagent that has gained considerable traction in laboratory settings is SYBR Green I. This fluorescent dye has proven its mettle in various applications, particularly in real-time PCR. Understanding the benefits of using SYBR Green I not only informs experimental design but also propels advancements in nucleic acid research.
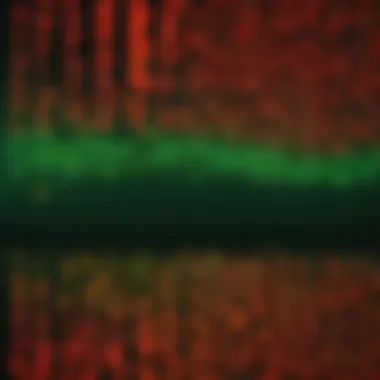
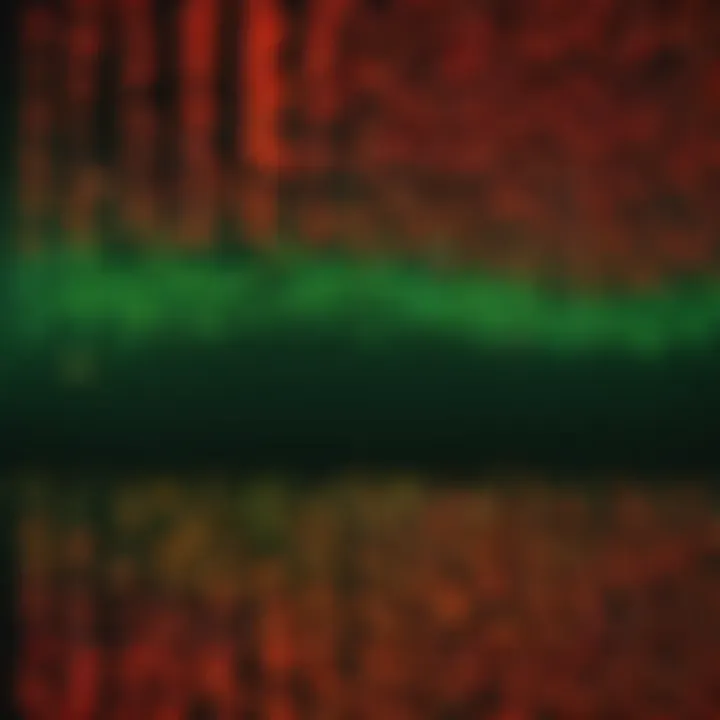
Higher Sensitivity and Detection Limits
Sensitivity in detection is crucial in any biological experiment. SYBR Green I excels in this domain, often outperforming competitors. It binds to double-stranded DNA, resulting in fluorescence that can be measured with high precision. This enhanced sensitivity facilitates the detection of low-abundance targets, making it invaluable for researchers working on projects like gene expression studies or pathogen detection.
Here are some key aspects regarding its sensitivity:
- Low Quantity Needs: With SYBR Green I, researchers can work effectively with minimal amounts of samples, which is particularly beneficial when precious specimens are at stake.
- Dynamic Range: The dye offers an impressive dynamic range, enabling accurate quantification across a wide variety of concentrations, thus ensuring reliable detection outcomes.
- Real-time Data: The real-time nature of SYBR Green I’s fluorescence allows for iterative analysis as the PCR progresses, leading to quicker and often more relevant conclusions.
By achieving higher sensitivity levels, SYBR Green I supports intricate molecular investigations, hence proving its worth in modern biology.
Cost-Effectiveness and Accessibility
Cost is a leading concern in research settings, where grants and budgets often dictate operational capacity. SYBR Green I presents a cost-effective alternative compared to other fluorescent options on the market. This affordability does not come at the expense of quality, making it a preferred choice, particularly for laboratories with tighter budgets.
Consider the following advantages:
- Affordable Supplies: SYBR Green I is generally available at a lower price point than specialized probes, thus allowing labs to allocate funds judiciously across other essential needs.
- User-Friendly Protocols: The dye requires minimal optimization, and many suppliers provide straightforward protocols for its application, reducing the time and effort that must be dedicated to training staff in complex methodologies.
- Wide Availability: Easily obtainable from various scientific supply companies, SYBR Green I can be found in most reagent catalogs, enhancing its accessibility for both seasoned researchers and newcomers alike.
Ultimately, the cost-effectiveness and accessibility of SYBR Green I not only make it a staple in molecular biology but also encourage widespread use, fostering collaborative advancements across different research fronts.
Using SYBR Green I can significantly impact research outcomes, making intricate studies not only feasible but also efficient in terms of both time and resources.
Limitations of SYBR Green
Understanding the limitations of SYBR Green I is crucial for any researcher aiming for precise result in molecular biology experiments. While this dye shines in multiple applications, a clear grasp of its constraints helps avoid pitfalls in experimental design. Key limitations can dictate the accuracy of your findings, and that's a critical aspect to mull over in any research scenario.
Specificity Issues in Multiplex PCR
One of the notable challenges with SYBR Green I is its specificity, particularly when it comes to multiplex PCR setups. In multiplex PCR, multiple targets are amplified in a single reaction, which can be a double-edged sword.
- Non-target amplification: SYBR Green I does not discriminate between specific sequences and non-target sequences. This can lead to the unwanted amplification of non-specific products, which in turn can interfere with data interpretation and quantification. For instance, if you are analyzing a target gene and two non-specific bands appear in your gel, you could mistakenly interpret these results as substantial expression of your target, throwing off your entire analysis.
- Differential efficiency: When using SYBR Green I in multiplex conditions, the dye may bind differently to various amplicons, creating discrepancies in fluorescent signals. This can further complicate quantification efforts, as you might end up with varied signals even when the actual target RNA quantity is the same across samples.
- Optimization required: To mitigate these issues, careful design of primers and conditions becomes vital. It’s a common practice to test combinations of primers before finalizing the multiplex reactions. This optimization phase can be time-consuming, often requiring several rounds of testing just to nail down the specific combinations that yield the best results.
Potential for Non-Specific Amplification
Non-specific amplification is another thorn in SYBR Green I’s side. The primary concern lies in its mechanism, which relies on the intercalation of the dye into double-stranded DNA. If your reaction conditions are not tuned to perfection, there can be a rise in non-specific products.
- Boosting background fluorescence: This phenomenon stems from the formation of primer-dimer artifacts. These artifacts may form when primers anneal to each other instead of to the target DNA. SYBR Green I binds to these unintended products just as it does with the true target, leading to background fluorescence that can drown out the true signal. Such disruptions could mislead you into thinking that you’ve achieved the desired amplification when in fact it is just background noise.
- Statistical implications: In quantification assays, these non-specific products can nod to false positives in results. For instance, in a statistical analysis, if your assay reports a higher accumulation of non-target sequences, conclusions drawn can lead to misrepresentation of the expression levels in studies like gene expression profiling.
- Reevaluation of parameters: To address this, regular evaluation of amplification efficiency and specificity through methods such as melt curve analysis can be useful. By doing so, researchers can establish baseline thresholds for acceptable levels of non-specific amplification, creating a safeguard against erroneous results.
It’s essential to prioritize specificity when designing experiments that utilize SYBR Green I. Familiarity with potential limitations ensures researchers can avoid common traps, leading to more reliable outcomes whole experimental endeavors.
Navigating the limitations of SYBR Green I is not just about acknowledging issues but also about strategically overcoming them. With each limitation outlined here, you gain the insight needed to optimize your research protocols effectively.
Optimization in Experimental Design
When it comes to experiments that involve SYBR Green I, optimization of experimental design is not just a suggestion; it’s a necessity. The accuracy and reproducibility of results hinge upon a well-thought-out approach regarding various experimental parameters. This section will delve into the nitty-gritty of optimization, shedding light on specific elements that can make or break your results.
Optimal Dye Concentration
Choosing the right concentration of SYBR Green I is pivotal. The dye’s ability to intercalate with DNA means that its concentration directly impacts fluorescence intensity. If the concentration is too low, the signal may not be detectable. Conversely, too high a concentration can lead to high background fluorescence and non-specific signal, which muddles your interpretation of the results.
A practical guideline is to start with the manufacturer’s recommendations. However, realize that this might not always suit your specific experimental context. For example, when working with a particular template or primer set, it may be worthwhile to perform a preliminary titration to determine the optimum dye concentration. Using a gradient PCR setup allows you to keep other variables constant while fine-tuning the SYBR Green I concentration, ensuring you strike the right balance.
"In the world of molecular biology, precision is the name of the game. The smallest detail can have the biggest impact."
Additionally, consider factors like the type of nucleic acid and the specific PCR mechanism while deciding on the concentration. When amplifying long fragments of DNA, a higher concentration of SYBR Green I can help overcome potential issues linked to lower detection sensitivity. In contrast, for short amplicons, lower concentrations may suffice. An understanding of your target nucleic acid and the dynamics at play will shine a light on the path to optimization.
PCR Cycle Adjustments
Adjusting an experimental design often demands tweaks in the PCR cycles as well. PCR cycle times directly influence the efficiency of amplification. The number of cycles largely depends on the amount of starting material and the efficiency of the amplification process itself. Too many cycles may lead to misleading quantification because of non-specific products.
Typically, 30 to 40 cycles is a common range for most applications; however, this can vary depending on your specific needs. A good practice is to conduct a control reaction with a known sample to set a benchmark for optimization. Different samples might react differently, so the ability to adjust your cycles based on the reaction dynamics is necessary.
Moreover, consider varying the denaturation, annealing, and extension times. Optimal times can differ based on DNA complexity and size, as well as primer design. Shorter denaturation times may work if you're using high-fidelity polymerases. Conversely, certain DNA templates may benefit from longer extension periods to ensure complete amplification.
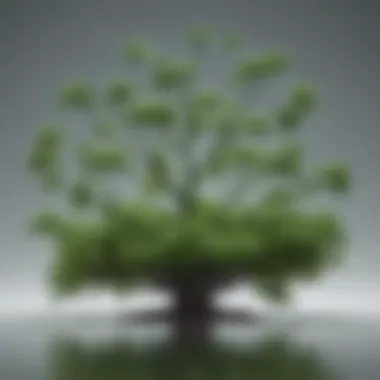
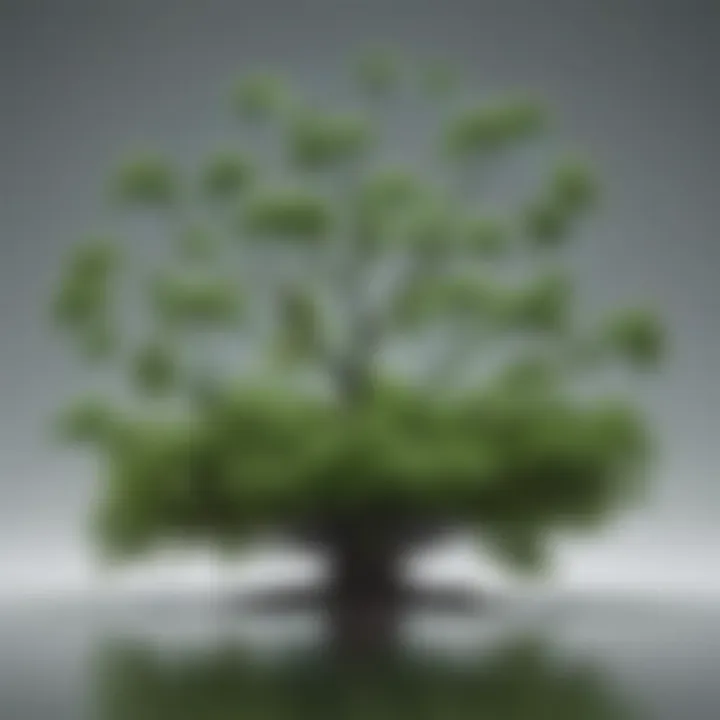
In summary, thoughtful optimization within your experimental design can pave the way for more reliable and interpretable results. Using optimal dye concentrations combined with properly adjusted PCR cycles not only enhances reaction efficiency but also ensures the accuracy of nucleic acid quantification. As researchers delve deeper into the complexities of molecular biology, these small changes orchestrate a symphony of successful experiments.
Comparison with Alternative Fluorescent Dyes
When exploring molecular biology techniques, particularly in nucleic acid quantification, one cannot disregard the importance of comparing SYBR Green I with other fluorescent dyes. Each dye offers unique characteristics, and understanding these nuances is pivotal for researchers seeking reliability and accuracy in their results. This section delves into specific alternatives and weighs their strengths and weaknesses against SYBR Green I.
TaqMan Probes
TaqMan probes are a popular alternative to SYBR Green I; they come with their own advantages. Utilization of TaqMan probes involves a specific oligonucleotide structure that carries a fluorescent dye at one end and a quencher at the other. During PCR, the probe hybridizes to the target sequence. As the polymerase enzyme progresses, it cleaves the probe, leading to the release of the dye from the quencher—thus allowing fluorescent signal detection.
This type of fluorescent detection has some clear advantages:
- Specificity: The probes are designed for particular sequences, reducing the chance of detecting non-specific products. This is a huge benefit in complex reactions where multiple targets exist.
- Quantitative Accuracy: Because the signal increase only occurs with target amplification, TaqMan probes generally provide more reliable quantification, minimizing background noise.
However, there are downsides:
- Cost: Generally, TaqMan probes tend to be pricier than SYBR Green I. For labs working with budget constraints, this factor can be significant.
- Complexity of Design: Creating TaqMan probes requires additional expertise in primer design and validation, which can be a barrier for some researchers.
Despite these drawbacks, the specificity and quantitative capability of TaqMan probes make them a compelling option for applications requiring high precision in nucleic acid analysis.
Other SYBR Dyes
Diving deeper into the SYBR family, there are various alternative SYBR dyes available on the market. These dyes, such as SYBR Safe and SYBR Green II, also emanate fluorescence when binding to nucleic acids.
SYBR Safe is particularly noteworthy because it is designed to be less hazardous than traditional SYBR Green I, making it more suitable for educational settings where student safety is a priority. Other beneficial aspects can include:
- Reduced Toxicity: This lowers the risk during handling, which is important in environments that may not have stringent waste disposal protocols.
- Comparable Sensitivity: While it brings safety advantages, SYBR Safe aims to maintain similar sensitivity levels to SYBR Green I, making it a reliable alternative.
On the flip side, though:
- Performance Variability: Different formulations might show variability in sensitivity and specificity, which can complicate results validation.
- Limited Applications: Some of these alternative SYBR dyes are not as versatile as SYBR Green I in certain experimental setups, leading to a higher likelihood of performance issues depending on the application.
Future Trends in Nucleic Acid Detection
In the realm of molecular biology, staying ahead of the curve is crucial. As research demands increase and the need for rapid, accurate applications gains traction, the future of nucleic acid detection is carved by technological advancements. These transformations promise to heighten the accuracy and efficiency of assays while simultaneously streamlining workflows for researchers. Looking into the future helps scientists identify potential shifts that can raise the bar for quantification techniques. This section delves into significant advancements unfolding in fluorescent technologies and their integration with microfluidics, setting the stage for a new era in nucleic acid analysis.
Advancements in Fluorescent Technologies
The world of fluorescent technologies is experiencing a renaissance, driven by innovative approaches that enhance detection sensitivity and specificity. Here are some noteworthy developments:
- Nanoparticle-based Dyes: Fluorescent nanoparticles, such as quantum dots, are emerging as potent alternatives to traditional dyes. Their exceptional brightness and resistance to photobleaching present an alluring option for long-term experiments.
- Dual-Color Compatibility: New fluorescent dyes have been formulated to allow dual-color detection. This advancement can facilitate multiplex PCR reactions that amplify target genes simultaneously, providing a comprehensive understanding of gene expression within a single assay.
- Smart Dyes: The development of "smart" fluorescent dyes that change their fluorescence properties in response to specific factors like pH or temperature enhances versatility. Such responsiveness can offer valuable insights into the microenvironment surrounding the nucleic acids.
These advancements should not just be seen as technological novelty but rather as pivotal changes that will influence experimental design, enabling deeper biological insights and more efficient lab practices.
Integration with Microfluidics
Microfluidics is another sizzling area in the future of nucleic acid detection. By manipulating small volumes of fluids, researchers can achieve an unprecedented level of control over their reactions.
- Reduced Sample Volume: Microfluidics minimizes the amount of sample and reagents required. This reduction is particularly crucial when working with precious or limited biological samples.
- High-Throughput Screening: This technology facilitates the simultaneous analysis of multiple samples, making it particularly advantageous in scenarios where time and data volume are important. Researchers can save significant time and resources during experiments.
- Enhanced Sensitivity and Speed: Utilizing micro-scale assays increases reaction rates significantly, which tends to yield results faster without compromising the sensitivity of the detection methods. This is especially relevant in clinical settings, where rapid diagnostics can be a matter of life and death.
In integrating these innovative technologies, the future landscape of nucleic acid detection is shaping up to be not only more effective but also adaptable to a variety of research contexts. By keeping an eye on these trends, researchers can better anticipate future directions and optimize their practices to keep pace with emerging challenges in molecular biology.
"Adapting to advancements in technology is not merely a choice; it is becoming a necessity in the evolving narrative of scientific research."
Moving forward, these trends in fluorescent technologies and microfluidics pave the way for more precise, efficient, and flexible methodologies in nucleic acid detection, crucial for both academic research and clinical applications.
End and Implications
In light of the discussions throughout this article, it's pivotal to understand how SYBR Green I stands not only as a tool but as a cornerstone in the paradigm of molecular biology. Its ability to provide reliable quantification of nucleic acids makes it indispensable for various scientific inquiries, from basic research to applied biotechnology. This dye facilitates the assessment of genetic material in real-time, streamlining the experimentation process and yielding data that can be both reproducible and reliable.
Summary of Key Points
- Highlighting Mechanism of Action: SYBR Green I engages in intercalation between the nucleic acid bases, a critical function that lends to its fluorescence upon excitation. This property is at the heart of real-time PCR, enabling real-time visualization of DNA replication.
- Broad Applications Across Disciplines: This dye is pivotal in quantitative PCR, genotyping, and even microbial load assessments. Researchers from diverse domains, including medical diagnostics and environmental monitoring, leverage SYBR Green I for its versatility.
- Benefits and Limitations: While offering heightened sensitivity and cost-effectiveness, practitioners must be mindful of its specificity issues, particularly in multiplex PCR settings. A balanced understanding of these strengths and weaknesses is key in optimizing experimental outcomes.
- Future Prospects: With the advent of newer technologies, SYBR Green I is likely to evolve. Innovations, particularly in integration with microfluidic systems, promise to further enhance its application range, potentially simplifying complex workflows.
Considerations for Future Research
Moving forward, research diving into SYBR Green I could explore several avenues:
- Improving Specificity in Multiplex Environments: Addressing the challenges associated with non-specific amplification is essential. Future studies could focus on improving binding specificity while maintaining the sensitivity advantage.
- Alternative Formulations: Investigating novel fluorescent dyes or hybridizing SYBR Green I with advanced probes could offer a pathway to enhance signal detection while managing pitfalls.
- Field Applications: Understanding how SYBR Green I can bridge laboratory results with fieldwork, like environmental sample assessments, could be invaluable. Tailoring protocols to various field conditions will help in creating adaptable methodologies.
- Cross-Disciplinary Approaches: Exploring collaborations between chemists, biologists, and engineers might lead to innovative solutions in dye development and application.
In summation, while SYBR Green I is a well-established reagent in molecular assays, its journey is far from over. The future holds potential, contingent upon continuous improvement, understanding its limits, and exploring the untapped possibilities within its framework.