Understanding RNA Synthesis in Cellular Biology
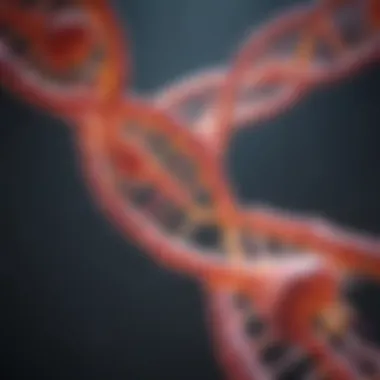
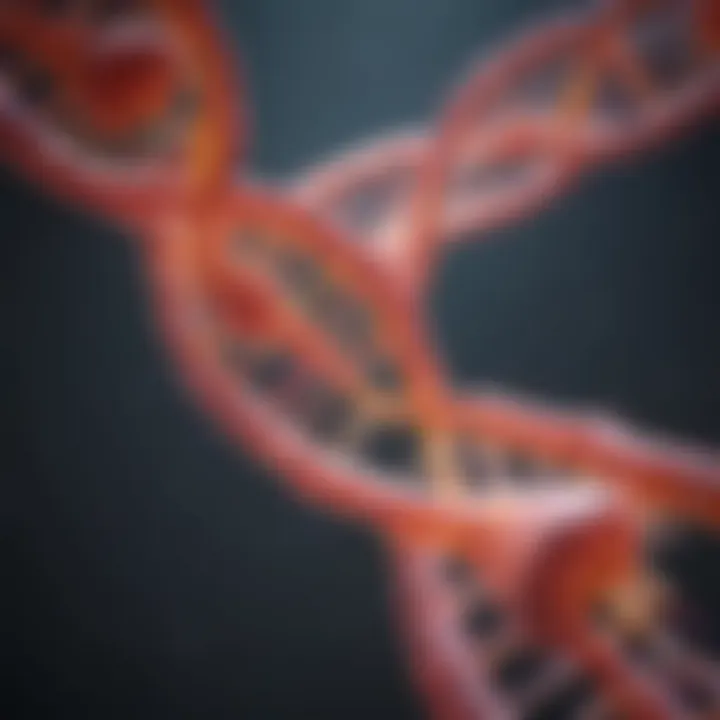
Intro
RNA synthesis, a vital process in cellular biology, is the means by which genetic information is translated into functional molecules. This process, also known as transcription, involves several complex mechanisms that are essential for proper cell function. Understanding RNA synthesis enhances insight into gene expression, regulation, and cellular activities.
The significance of RNA lies not just in its functions as a messenger between DNA and proteins, but also in its various forms, including mRNA, tRNA, and rRNA, each contributing to the intricate web of cellular operations. This article will explore the essential elements of RNA synthesis and its implications in biological science.
Research Overview
Summary of Key Findings
Research indicates that RNA synthesis is mediated largely by RNA polymerases, enzymes that transcribe DNA into RNA. The three main types of RNA produced during transcription are:
- Messenger RNA (mRNA): Carries genetic code for protein synthesis.
- Transfer RNA (tRNA): Delivers amino acids for protein assembly.
- Ribosomal RNA (rRNA): Forms the core structural and functional components of ribosomes.
Post-transcriptional modifications, including capping, polyadenylation, and splicing, also play a crucial role in ensuring the proper function and stability of RNA molecules.
Methodologies Employed
The methodologies for understanding RNA synthesis include:
- Experimental Techniques: Techniques such as RT-PCR (Reverse Transcription Polymerase Chain Reaction) and Northern blotting are used to analyze RNA molecules.
- Genomic Analysis: Next-generation sequencing assists in understanding transcription landscapes and RNA integrity.
These techniques collectively aid in clarifying the mechanisms of RNA synthesis and its regulation within the cell.
In-Depth Analysis
Detailed Examination of Results
The findings from various studies reflect the complexity of RNA synthesis. For example, RNA polymerases I, II, and III each have distinct roles, transcribing different classes of RNA. This specificity is critical in maintaining cellular function and integrity. The regulation of RNA synthesis can be influenced by several factors, including transcription factors, enhancers, and silencers, which can modulate the efficiency of gene expression.
Comparison with Previous Studies
In comparing newer studies with previous research, it becomes evident that our understanding of RNA synthesis has evolved. Earlier works focused primarily on mRNA synthesis, while recent research emphasizes the importance of non-coding RNAs and their regulatory roles in gene expression. This shift in focus highlights the dynamic nature of gene expression and the necessity of RNA synthesis in various biological processes.
"Understanding RNA synthesis is foundational to grasping the complexities of cellular biology and genetics."
Foreword to RNA Synthesis
The initiation of RNA synthesis marks a critical step in the realm of cellular biology. Understanding RNA synthesis is fundamental as it is intricately linked to genetic expression and the regulation of cellular functions. The synthesis of RNA encompasses a complex series of events, which ultimately govern how genetic information is translated into functional proteins. These proteins play crucial roles in various biological processes, making the study of RNA synthesis an essential component for students, educators, researchers, and professionals alike.
Definition of RNA
Ribonucleic acid, commonly known as RNA, is a nucleic acid that serves various key functions in cells. Structurally, RNA is composed of nucleotides, akin to its relative DNA, but with notable differences. RNA contains ribose sugar, while DNA contains deoxyribose sugar, and in addition, RNA has uracil instead of thymine. RNA exists in several forms, each fulfilling distinct roles, including messenger RNA (mRNA), ribosomal RNA (rRNA), and transfer RNA (tRNA). These different types collectively facilitate the flow of genetic information from DNA to proteins.
Importance of RNA in Cellular Functions
RNA is pivotal for a myriad of cellular functions. Primarily, it is the messenger carrying genetic instructions from DNA in the nucleus to the cytoplasm. This transmission is crucial for protein synthesis, as proteins are synthesized based on the template provided by mRNA. Here are some key points regarding the importance of RNA in cellular functions:
- Gene Expression Regulation: RNA molecules are involved in regulating gene expression, ensuring that specific genes are turned on or off at appropriate times.
- Protein Synthesis: mRNA, rRNA, and tRNA interact during the translation process to produce proteins necessary for cellular structure and function.
- Catalytic Functions: Certain RNA molecules, known as ribozymes, possess catalytic properties, enabling them to facilitate biochemical reactions within cells.
- Cellular Response: RNA plays a role in how cells respond to environmental changes, helping adapt cellular functions to meet physiological demands.
Understanding the nuances of RNA functions offers insights into cellular mechanics and potential therapeutic pathways for various diseases.
Overview of the Cell's Machinery
The machinery within a cell is remarkably complex, playing an essential role in the synthesis of RNA. Understanding this machinery is vital because it sets the framework upon which RNA synthesis occurs. Cells operate with a wide array of structures and components, all contributing to the intricate process of RNA transcription and processing. The information presented in this section can help clarify how different organelles work in harmony to facilitate RNA synthesis and the subsequent cellular responses to RNA products.
Cell Structure and Organelles Involved
Cells possess various organelles that collectively support RNA synthesis. Each structure has specific functions that affect the overall process. The following are key organelles involved in RNA synthesis:
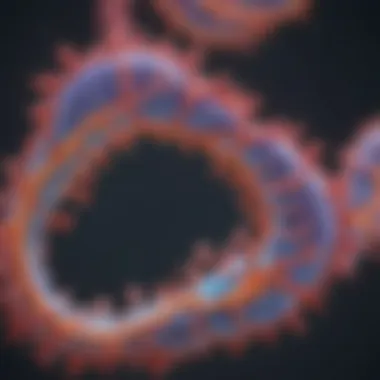
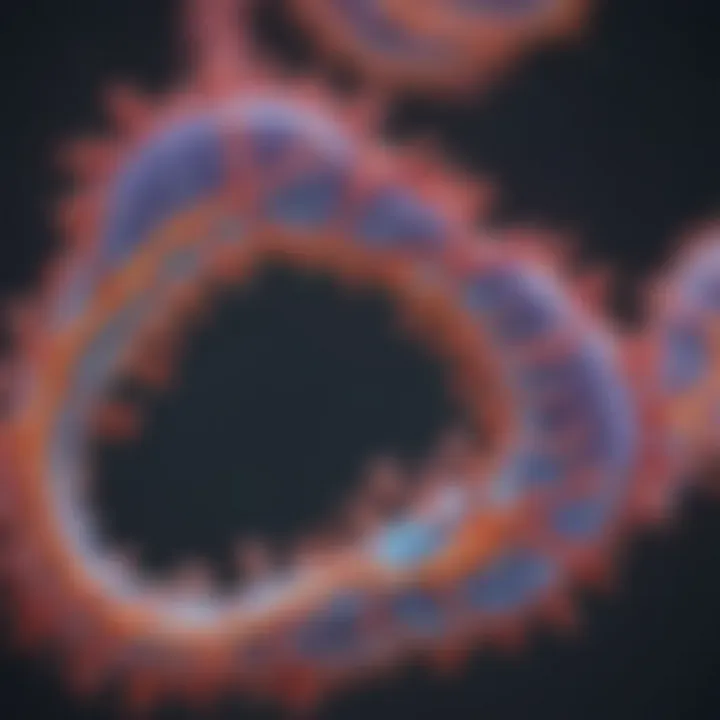
- Nucleus: Often referred to as the control center, the nucleus houses the cell's genetic material. It is where RNA synthesis primarily takes place, initiating the transcription of DNA into RNA.
- Ribosomes: These organelles play a crucial role after RNA synthesis. Ribosomes translate messenger RNA (mRNA) into proteins, essential for cell function and structure.
- Endoplasmic Reticulum (ER): The rough ER, in particular, is studded with ribosomes and is where a significant amount of protein synthesis takes placeβdriven by the mRNA produced in the nucleus.
- Golgi Apparatus: This organelle modifies and packages proteins for secretion or use within the cell, playing a critical role in post-transcriptional processing.
Each of these organelles must perform its role efficiently to ensure the proper synthesis, processing, and utilization of RNA within the cell. Disruption in any of these components can have significant implications on RNA metabolism and, consequently, cellular functionality.
Role of the Nucleus in RNA Synthesis
The nucleus is undeniably central to RNA synthesis. It contains the cell's DNA, which serves as a template for RNA production. During the transcription phase, RNA polymerase binds to specific sequences in the DNA, unwinding it and synthesizing RNA complementary to the DNA strand. The nucleus not only facilitates this crucial process but also manages various regulatory mechanisms ensuring accurate and timely RNA synthesis.
Key functions of the nucleus include:
- Transcription Regulation: The nucleus contains transcription factors that help in regulating gene expression by influencing RNA polymerase activity. These factors can enhance or suppress the transcription of specific genes, allowing the cell to respond to internal and external stimuli effectively.
- RNA Processing: After transcription, the produced precursor RNA (pre-mRNA) undergoes several modificationsβcapping, polyadenylation, and splicingβbefore it becomes mature mRNA ready for translation. The nucleus harbors the necessary machinery for these modifications, ensuring that only correctly processed RNA is exported to the cytoplasm.
- Nuclear Pores: These structures facilitate the transport of mature RNA out of the nucleus into the cytoplasm, where translation occurs. This transport is tightly regulated to prevent the accumulation of unprocessed RNA in the cytoplasm.
Transcription: The Process of RNA Synthesis
Transcription is a pivotal process in cellular biology that serves as the bridge between genetic information encoded in DNA and functional RNA molecules. Understanding transcription is essential, as it lays the groundwork for RNA synthesis. In this section, we will discuss the specific elements of transcription, its benefits to the cell, and the considerations that are crucial for this fundamental process.
Initiation of Transcription
Initiation marks the first step of transcription, where the transcription machinery assembles at the promoter region of a gene. The intricate assembly of proteins, which includes transcription factors, is crucial at this stage. These factors recognize and bind to the DNA sequence, facilitating the recruitment of RNA polymerase.
The process begins when RNA polymerase binds to the promoter, inducing a conformational change that unwinds the DNA strands. The exposed template strand serves as a matrix for synthesizing the RNA transcript. It is important to understand that precise initiation is critical for accurate gene expression. Any errors here can lead to improper transcription and affect cellular function.
Elongation Phase of Transcription
Following initiation, the elongation phase commences. During this phase, RNA polymerase traverses the DNA, synthesizing RNA in the 5' to 3' direction. Nucleotides are added one by one, complementing the DNA template strand.
As RNA polymerase moves along the DNA, it simultaneously unwinds the DNA double helix and re-anneals the unwound regions. This allows the continuous synthesis of the RNA strand, which lengthens steadily. The speed of this elongation process can affect how quickly a gene is expressed. Errors can occur during this phase, but RNA polymerase has a proofreading mechanism that reduces the number of mistakes made during transcription.
Termination of Transcription
Termination signifies the conclusion of the transcription process. It occurs when RNA polymerase encounters specific termination signals in the DNA sequence. These signals instruct RNA polymerase to stop elongation and dissociates from the DNA template.
The newly synthesized RNA molecule is then released and undergoes various modifications for functionality. It is noteworthy that the termination process can be affected by several factors, including the presence of specific proteins that could alter RNA polymerase activity. The rate and accuracy of termination play a crucial role in determining the quality and quantity of RNA produced in the cell.
"The regulation of transcription is paramount to the overall gene expression and cellular identity, making it a critical focus for research in molecular biology."
In summary, transcription is a complex yet vital process involving initiation, elongation, and termination. Each phase must be executed with precision to ensure the proper synthesis of RNA, impacting cellular functions significantly.
RNA Polymerases and Their Functions
RNA polymerases are essential enzymes that play a crucial role in the transcription process of RNA synthesis. Their functions are central to all cellular processes involving RNA. Understanding these enzymes is vital for insights into genetic expression and regulation.
Types of RNA Polymerases
Eukaryotic cells contain three main types of RNA polymerases, each responsible for the synthesis of different types of RNA:
- RNA Polymerase I: This polymerase synthesizes ribosomal RNA (rRNA), which is a major component of ribosomes. These structures are vital for protein synthesis in cells.
- RNA Polymerase II: This enzyme is primarily responsible for synthesizing messenger RNA (mRNA). This type of RNA carries genetic information from DNA to ribosomes, where protein synthesis occurs. RNA polymerase II also synthesizes some small nuclear RNAs (snRNA), which are involved in RNA splicing.
- RNA Polymerase III: This polymerase synthesizes transfer RNA (tRNA) and other small RNAs, such as 5S rRNA. tRNA is crucial for translating the genetic code into proteins.
Each type of polymerase exhibits distinct properties and requirements, which dictate their specific functions within the cell.
Mechanisms of RNA Polymerase Action
The action of RNA polymerases is a multi-step process that involves several key mechanisms:
- Initiation: RNA polymerases require specific sequences on the DNA called promoters, where transcription begins. After forming a complex with transcription factors, the polymerase unwinds a segment of the DNA to access the template strand.
- Elongation: As RNA polymerase moves along the DNA, it catalyzes the formation of RNA by adding ribonucleotides complementary to the DNA template. The RNA strand grows in the 5' to 3' direction. This elongation phase requires the energy derived from the polymerization of nucleotides.
- Termination: Once a termination signal is reached on the DNA, RNA polymerase detaches from the DNA and releases the newly formed RNA molecule. This step is crucial, as it indicates the end of transcription and ensures proper regulation of gene expression.
"RNA polymerase is fundamental to RNA synthesis, directly influencing gene expression in all living organisms."
The interplay of different RNA polymerases and their specific action mechanisms allows for precise regulation of gene expression in response to cellular signals and environmental conditions. Understanding these processes provides significant insights into cellular biology and the molecular underpinnings of life.
Types of RNA Produced in Cells
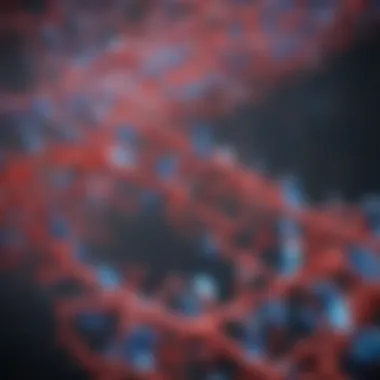
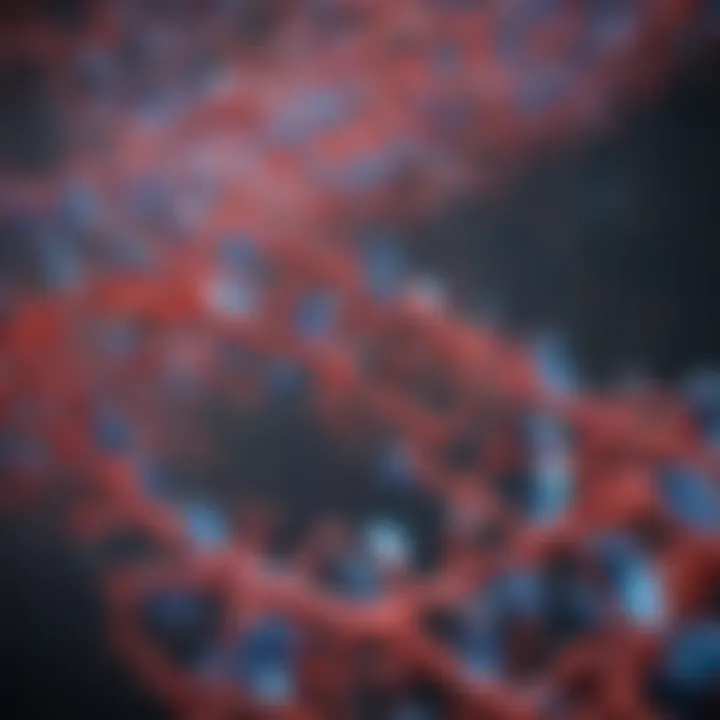
The synthesis of RNA within cells leads to various types of RNA molecules, each serving distinct functions crucial for cellular biology. Understanding these types of RNA is significant in illustrating how genetic information is expressed and regulated. The primary categories include messenger RNA (mRNA), ribosomal RNA (rRNA), transfer RNA (tRNA), and non-coding RNAs. Each type plays a pivotal role in gene expression, protein synthesis, and the overall functionality of the cell.
mRNA: Messenger RNA
Messenger RNA is perhaps the most recognized type of RNA. It acts as the intermediary between the genetic information encoded in DNA and the synthesis of proteins. When a gene is transcribed, the resulting mRNA carries the complementary sequence from the DNA, allowing ribosomes to translate it into proteins. Importantly, mRNA molecules undergo a series of modifications before they can be utilized. These modifications include 5' capping and polyadenylation, which help stabilize the mRNA and facilitate its recognition by the ribosomal machinery. Thus, mRNA is essential for conveying genetic codes and directing the production of proteins, thereby influencing various cellular functions.
rRNA: Ribosomal RNA
Ribosomal RNA is a crucial component of ribosomes, the cellular machinery that synthesizes proteins. rRNA molecules form the structural and functional core of ribosomes, aiding the process of translation. Each ribosome consists of two subunits, each containing rRNA and proteins. The rRNA not only provides a scaffold for ribosomal proteins but also has enzymatic roles, facilitating peptide bond formation during translation. The significance of rRNA extends to its involvement in the accuracy of protein synthesis, ensuring that amino acids are assembled correctly according to the mRNA sequence. Without rRNA, ribosomes could not function effectively, and protein synthesis would be significantly impaired.
tRNA: Transfer RNA
Transfer RNA serves as the adaptor molecule that brings amino acids to the ribosome during protein synthesis. Each tRNA is specific for one amino acid and has an anticodon region that pairs with the corresponding codon on the mRNA strand. This specificity ensures that the correct amino acid is incorporated into the growing polypeptide chain. tRNA plays a critical role in maintaining the fidelity of translation. A malfunction in tRNA can lead to errors in protein synthesis, potentially resulting in nonfunctional or harmful proteins. Therefore, the role of tRNA is indispensable in the translation process, linking the genetic code to the physical structure of proteins.
Non-coding RNAs: A Diverse Group
Non-coding RNAs encompass a variety of RNA molecules that do not code for proteins but are vital for regulating gene expression and maintaining cellular homeostasis. This group includes microRNAs, long non-coding RNAs, and small interfering RNAs, among others. Non-coding RNAs influence various biological processes such as gene silencing, chromatin remodeling, and RNA splicing. For instance, microRNAs can bind to target mRNAs, causing their degradation or inhibiting their translation. This regulatory potential highlights the importance of non-coding RNAs in fine-tuning cellular responses and maintaining the delicate balance of gene expression. Emerging research continues to uncover the complex roles non-coding RNAs play in health and disease, indicating their significance in cellular biology.
"The discovery of non-coding RNAs has revolutionized our understanding of regulation in cellular processes."
In summary, the types of RNA produced in cells are foundational to understanding the mechanisms that drive cellular functions. Each RNA type has specific roles that contribute to the intricate processing of genetic information, ultimately influencing cellular behavior and physiology.
Post-transcriptional Modifications of RNA
Post-transcriptional modifications are pivotal steps in the life cycle of RNA molecules, essential for the functionality and stability of the RNA post synthesis. Once RNA is synthesized, it undergoes several alterations that ensure it is mature and ready for its various roles in cellular processes. These modifications serve not only to stabilize the RNA but also to regulate gene expression and enhance the efficiency of translation.
' Capping
5' capping involves the addition of a modified guanine nucleotide at the 5' end of the RNA molecule shortly after transcription initiation. This cap structure is crucial for several reasons:
- Protection from degradation: The cap protects RNA from exonucleases, thus prolonging its lifespan within the cell.
- Facilitates ribosome binding: It is required for the recognition of the mRNA by the ribosome during translation initiation.
- Regulation of nuclear export: The cap is recognized by nuclear export proteins, which facilitate the transport of the mRNA from the nucleus to the cytoplasm.
Without proper capping, the RNA may be rapidly degraded or may not be efficiently translated, impacting protein synthesis.
Polyadenylation
Polyadenylation refers to the addition of a poly(A) tail to the 3' end of the RNA molecule. This modification is significant due to several factors:
- Stabilization of mRNA: The poly(A) tail enhances the stability of the mRNA and protects it from degradation.
- Regulation of translation: A longer poly(A) tail generally correlates with increased translation efficiency, as it enhances ribosome binding.
- Involvement in gene expression regulation: The length of the poly(A) tail can influence how long the mRNA remains active in the cytoplasm, affecting gene expression levels.
Polyadenylation is thus an essential regulatory mechanism in post-transcriptional control and cellular function.
Splicing Mechanisms
Splicing is a crucial process whereby introns are removed from the pre-mRNA and exons are joined together to form a continuous coding sequence. The significance of splicing lies in:
- Diversity of protein products: Alternative splicing allows a single gene to produce multiple protein isoforms, thereby enhancing the diversity of the proteome.
- Quality control: The splicing machinery ensures that only properly processed RNA molecules are exported from the nucleus, which maintains the integrity of the cellular protein synthesis machinery.
- Regulation of gene expression: By modulating exon inclusion or exclusion, splicing can affect the levels and types of proteins produced from a given mRNA transcript.
These mechanisms exemplify the complexity of RNA processing and its integral role in cellular functions.
"Post-transcriptional modifications are not just simple reactions; they are essential processes that determine the fate and function of RNA inside the cell."
In summary, post-transcriptional modifications such as 5' capping, polyadenylation, and splicing are vital for ensuring RNA molecules are functionally competent and stable. Understanding these processes sheds light on the nuances of gene expression and regulation in cellular biology.
RNA Regulation and Control Mechanisms
RNA regulation and control mechanisms are essential in cellular biology. This topic highlights how RNA production and stability are finely tuned to meet the specific demands of the cell. Regulation impacts not only gene expression but also various cellular processes, ensuring that the correct proteins are synthesized at the right time.
Transcription Factors and Their Roles
Transcription factors are proteins that bind to specific DNA sequences. They play a crucial role in initiating and regulating the transcription of genes into RNA. Each transcription factor can control the expression level of multiple genes.
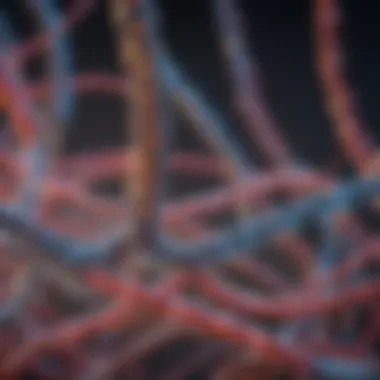
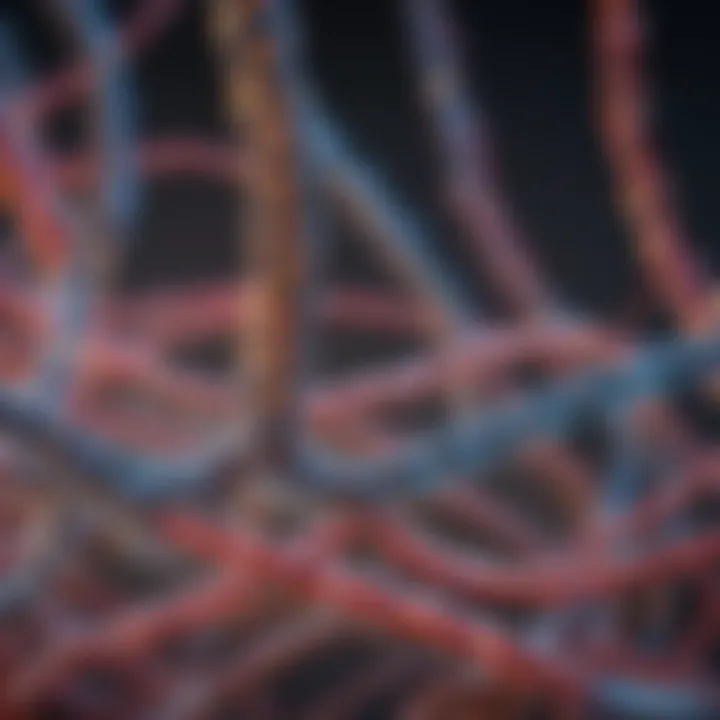
- Activation: Some transcription factors enhance gene expression by promoting the binding of RNA polymerase to the DNA template.
- Repression: Other transcription factors inhibit transcription by blocking RNA polymerase activity.
The interaction between transcription factors and other molecules within the cell creates a complex network. This network allows cells to respond to environmental signals and developmental cues. Consequently, transcription factors are vital for cellular identity and function. Their ability to integrate signals ensures that genes are expressed in a context-dependent manner.
Enhancers and Silencers in Gene Regulation
Enhancers and silencers are regulatory DNA sequences that influence transcription. Enhancers are regions that, when bound by specific proteins, increase the likelihood of transcription, while silencers decrease it.
- Enhancers:
- Silencers:
- Can be located far from the gene they regulate.
- Function via looping to bring transcription factors and RNA polymerase to the promoter region.
- Enable coordinated expression of genes in response to certain stimuli.
- Act as binding sites for repressor proteins.
- Can also be distant from the target gene but effectively lower transcription levels.
- Play a role in maintaining cell specificity by ensuring that non-essential genes are not expressed in a given cell type.
In essence, enhancers and silencers contribute to the precision of gene regulation, enabling a flexible response to internal and external conditions.
Together, transcription factors, enhancers, and silencers form a sophisticated regulatory system. This system is critical for the proper functioning of RNA synthesis and, by extension, cellular biology. Understanding these mechanisms opens a window into the intricate workings of gene expression and its implications for health and disease.
RNA Degradation and Its Implications
RNA degradation is a critical process in cellular biology. It influences how cells respond to changes in the environment and how they maintain homeostasis. The effective regulation of RNA turnover ensures that cells can fine-tune gene expression according to their physiological needs. Understanding this subject is vital, as the stability and lifetime of RNA molecules directly impact protein synthesis, cellular signaling, and overall metabolic functions.
Mechanisms of RNA Degradation
Several mechanisms exist for the degradation of RNA. These processes are essential for cellular regulation and the management of gene expression. Key pathways include:
- Exonucleolytic degradation: This is a common method where RNA is degraded from one end, usually the 5' or 3' end. This can occur via exosomes or other exonucleases.
- Endonucleolytic cleavage: In this process, RNA molecules are cleaved at specific internal sites, which can result in the formation of smaller RNA fragments that are subsequently degraded.
- Non-coding RNA pathways: Specific small RNAs can guide the degradation of their target mRNAs by base-pairing, leading to the recognition by ribonucleases.
These mechanisms help to regulate the levels of RNA within the cell and can play an essential role in cellular responses to stress or changes.
Impact of RNA Turnover on Gene Expression
RNA turnover has profound implications for gene expression. The rate of degradation governs how long an mRNA molecule remains stable inside the cell. Rapid turnover of mRNA can lead to tight regulation of protein production, allowing cells to adjust quickly to internal or external signals.
"RNA degradation is as crucial as synthesis, as it controls the lifespan of mRNA and, thus, the duration of its instructions for protein synthesis."
Considerations about RNA turnover include:
- Adaptation to Stress: Cells facing stress can increase degradation of certain mRNAs while stabilizing others, allowing for a quick shift in necessary protein synthesis.
- Cell Differentiation: During development, specific programs of RNA degradation contribute to the precise timing of gene expression, guiding differentiation processes.
- Disease Relevance: Abnormalities in RNA degradation pathways can lead to diseases, including cancer. Understanding these pathways gives insight into potential therapeutic targets.
In summary, RNA degradation is not merely a process of discarding unneeded molecules. It is essential for the dynamic and responsive nature of the cell, significantly influencing gene expression and cellular function.
Finale: The Role of RNA in Cellular Biology
Understanding the role of RNA in cellular biology is crucial for comprehending how genetic information is expressed and regulated within a cell. RNA is not just a byproduct of gene expression but an active participant in various cellular processes. Its synthesis, modification, and degradation influence numerous biological activities, from protein synthesis to regulatory mechanisms. This article has explored how RNA serves as a critical intermediary between DNA and proteins, facilitating the flow of genetic information essential for life.
Key processes in RNA synthesis include transcription, where RNA polymerase synthesizes RNA from a DNA template. This process is followed by critical modifications, ensuring the RNA is functional and properly regulated. A better understanding of these processes elucidates how cells respond to internal and external stimuli, and manage their resources. The interplay between different types of RNA β mRNA, rRNA, tRNA, and non-coding RNAs β adds layers of complexity to cellular functions, indicating that RNA is at the heart of gene expression regulation.
"RNA is not merely a messenger; it is integral to the expression and regulation of genes, influencing everything from metabolic pathways to signaling cascades."
In summary, the various roles of RNA underscore its significance in biological systems. Cellular health, adaptation, and response are intrinsically linked to the efficient synthesis and regulation of RNA molecules.
Summary of Key Processes in RNA Synthesis
The synthesis of RNA involves several key steps, each contributing to the overall understanding of gene expression:
- Initiation of Transcription: RNA polymerase binds to a promoter region of DNA, signaling the beginning of RNA synthesis. This step is crucial for determining which genes are expressed under specific conditions.
- Elongation Phase of Transcription: The RNA polymerase moves along the DNA, unwinding the double helix and elongating the RNA strand by adding nucleotides complementary to the DNA template.
- Termination of Transcription: Once a termination signal is encountered, the RNA polymerase detaches from the DNA, releasing the newly synthesized RNA molecule.
- Post-Transcriptional Modifications: These modifications ensure that the RNA is processed correctly. Key processes include 5' capping, polyadenylation, and splicing, which collectively enhance RNA stability and functionality.
These processes highlight RNAβs vital role in the architecture of gene expression and its regulation.
Future Directions in RNA Research
RNA research continues to evolve, leading to significant advancements in our understanding of cellular biology. Here are some areas showing promise for future investigations:
- CRISPR-Cas9 and RNA Editing: Advances in genome editing techniques rely on understanding RNA's role in targeted gene modification. Future applications may lead to therapeutic options for genetic disorders.
- Role of Non-Coding RNAs: Ongoing studies into non-coding RNAs reveal their potential in regulating gene expression, cellular signaling, and development. Their functions broadens the scope of molecular biology and can lead to novel targets for drug development.
- RNA and Disease Mechanisms: Research efforts are increasingly focusing on how dysregulation of RNA processes contributes to diseases, including cancer and neurodegenerative conditions. Understanding these connections could pave the way for innovative treatments.
- Synthetic Biology Applications: Engineering RNA molecules for specific functions is a growing field. This involves designing RNA to regulate gene expression, create biosensors, or even develop new therapeutic strategies.
These areas not only demonstrate the multi-faceted nature of RNA but also its potential as a biotechnological tool with applications in medicine, synthetic biology, and beyond. The future of RNA research holds the potential to deepen our understanding of life at the molecular level and to usher in new scientific discoveries.