Exploring Inductively Coupled Technologies and Uses
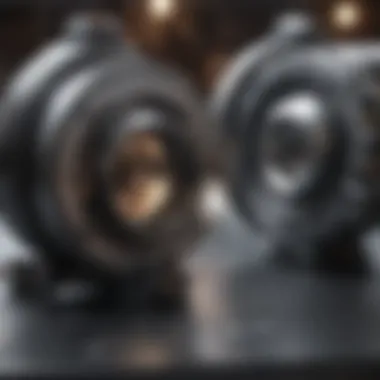
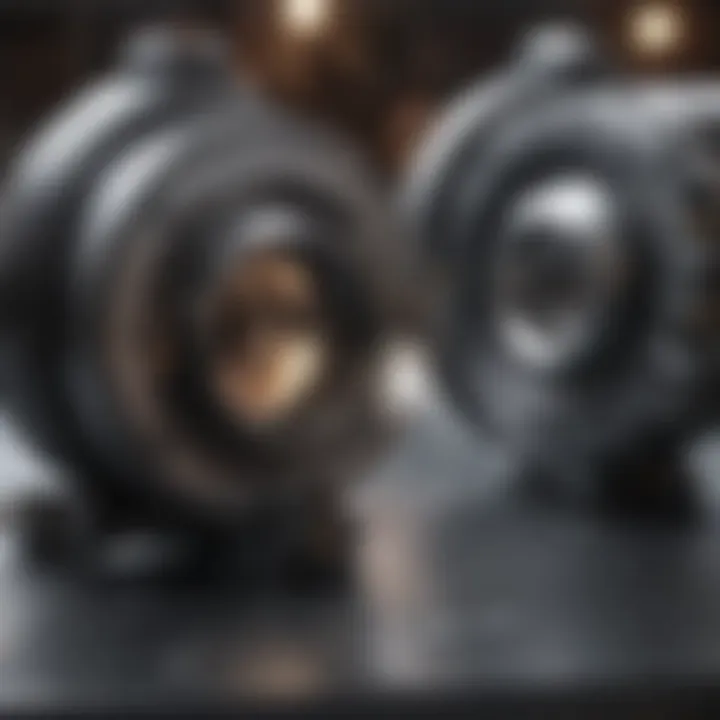
Intro
Inductively coupled technologies have created significant ripples in various scientific fields, revolutionizing how we interact with and understand different materials and processes. From analytical chemistry to telecommunications, the core principles of inductive coupling facilitate an array of applications that enhances both research and practical implementations. The importance of this technology cannot be understated, as it offers powerful tools for exploration and innovation that can shape the future.
As we delve into this article, we will peel back the layers of inductive couplingβstarting with its basic principles, progressing through its methodologies, and finally examining its impact across various industries. Far from being a niche topic, understanding these technologies is crucial to anyone looking to develop a solid foundation in modern science.
Research Overview
Understanding the principles that govern inductively coupled technologies grants insight into their remarkable capabilities. The main findings from recent studies highlight two critical components: efficiency and versatility.
Summary of Key Findings
Recent research underscores that inductively coupled plasma (ICP) systems offer:
- High sensitivity, making them ideal for trace element analysis.
- The ability to operate over a wide range of temperatures, enhancing adaptability.
- Minimal sample destruction, allowing for conservation of materials, particularly in elemental analysis.
Such findings echo the relevance of these technologies in both academic research and industrial processes, where precision and efficiency are paramount.
Methodologies Employed
Research methodologies related to inductively coupled technologies often involve:
- Spectroscopic Techniques: Particularly, ICP mass spectrometry is popular for measuring the concentration of elements in various samples, providing unparalleled accuracy.
- Modeling and Simulation: Employing computational methods to predict behavior in inductively coupled systems helps refine experimental approaches.
The blend of these methods ensures a comprehensive understanding of how core principles manifest in practical applications.
In-Depth Analysis
Going beyond the surface, more granular analysis of inductively coupled technologies is necessary to grasp their broader implications.
Detailed Examination of Results
One significant advantage of inductively coupled plasma sources is the lack of matrix effects compared to other atomic spectrometry methods. This aspect means that the presence of other elements in the sample does not significantly disrupt or alter results. Such robustness is critical in fields where sample integrity varies.
Furthermore, comparative studies reveal:
- ICP systems outperform traditional flame atomic absorption in sensitivity.
- They open avenues for multi-element detection, a daunting task for single-element methods.
Comparison with Previous Studies
Comparative analysis with earlier studies demonstrates how advancements in technology have significantly enhanced the adaptability and efficiency of inductively coupled techniques. For example, older methods often necessitated time-consuming sample preparations and calibration. In contrast, current innovations streamline these processes, making them more user-friendly for researchers and increasing accessibility across various fields.
"Continued improvements in inductively coupled technologies herald a new era of precision in analytical methods."
With advancements flowing steadily, understanding these methods and their practical capabilities is not just an academic exercise but essential in paving the way for future innovations.
Prolusion to Inductive Coupling
Understanding inductive coupling is essential for grasping the broader scope of inductively coupled technologies that permeate various fields today. At its core, inductive coupling facilitates the transfer of energy or signals without direct contact, leveraging the principles of electromagnetic fields. This ability opens doors to innovative solutions across sectors like telecommunications, analytical chemistry, and materials science.
Inductive coupling stands as a bridge between traditional conductive methods and new wireless technologies. Its importance cannot be overstated; the benefits of reduced wear and tear on connectors, increased operational safety, and enhanced flexibility in system design make it attractive for both research applications and commercial ventures. As we delve deeper into this topic, we will uncover the myriad applications and implications that arise from inductive coupling, setting the stage for how these technologies impact our daily lives.
Defining Inductive Coupling
Inductive coupling refers to the mechanism by which energy is transferred between two electrically isolated circuits through a shared magnetic field. When an electric current flows through a coil, it generates a magnetic field around it. If another coil is placed within this field, a current can be induced in that second coil due to changes in the magnetic field caused by the first coil. This principle is fundamental to technologies like transformers and aims to minimize resistive losses, making it a reliable choice for applications where efficiency is paramount.
In practice, the actual coupling is affected by multiple factors, such as the distance between coils, their alignment, and even the materials surrounding the coupling system. These variables make inductive coupling versatile yet challenging, requiring precise design and engineering.
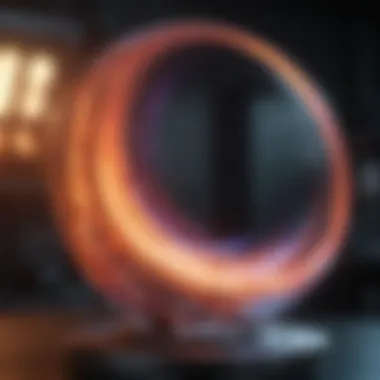
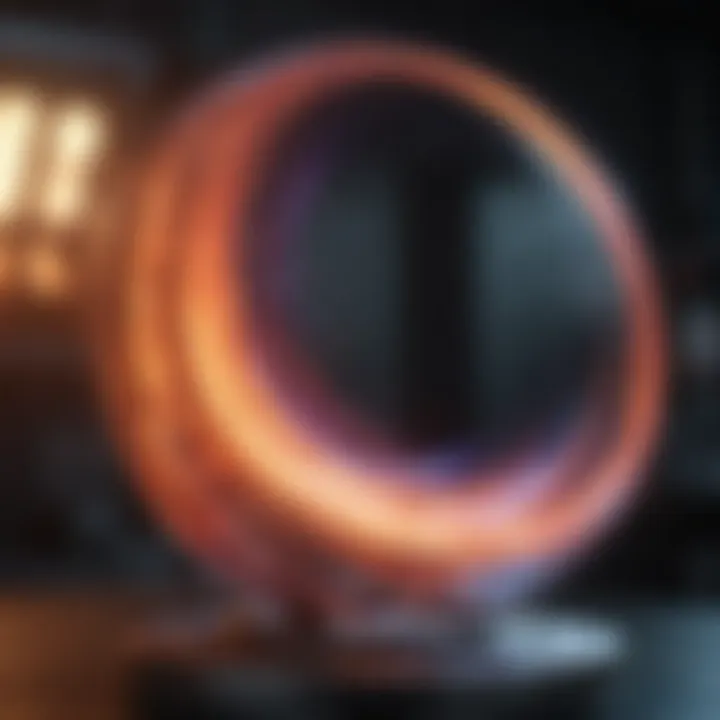
Historical Background
The idea of inductive coupling is not a modern concept; it has its roots in the exploration of electromagnetism dating back to the 19th century. Pioneering physicists like Michael Faraday and James Clerk Maxwell laid the groundwork for our understanding of electromagnetic induction. Faraday's law, which states that a changing magnetic field can induce an electromotive force (EMF) in a nearby circuit, was a breakthrough that paved the way for the application of inductive coupling.
Fast forward to the 20th century, and you'll find that inductive coupling was increasingly applied in various technologiesβeverything from radio transmitters to the more recent advancements in wireless charging systems for consumer electronics. The evolution of this technology is characterized by continual innovations that have made it more efficient and widespread. This background is vital as it highlights not only the historical significance but also the ongoing relevance in today's tech-driven world.
Fundamental Principles of Inductive Coupling
Inductive coupling operates on principles foundational to electromagnetism. This understanding is crucial when exploring inductively coupled technologies, as it lays the groundwork for their various applications. Essentially, inductive coupling involves the transfer of energy through inductively coupled coils, making it a pivotal technology in numerous fields, ranging from telecommunications to analytical chemistry. This section is intended to dissect its fundamental principles and elucidate why they matter.
Electromagnetic Induction
Electromagnetic induction is the heartbeat of inductive coupling. At its core, it entails generating an electric current in a conductor by varying the magnetic field around it. This phenomenon is an outcome of Faraday's law, a principle that explains how a change in the magnetic environment of a coil can induce voltage across the coil. The relationship can be summarized in a straightforward way: more changes in the magnetic field mean higher current generation.
To illustrate, picture a simple setup: a coil connected to a power supply and another coil nearby. When current flows through the first coil, it creates a magnetic field. If the current in the first coil is varied, the changing magnetic field induces a current in the second coil without direct contact. This principle not only facilitates wireless energy transfer but also enables the functioning of devices like transformers and inductive chargers. Indeed, inductive coupling reflects a bridge where electricity can dance through space without actual wires.
Basic Circuit Design
The circuit design for inductive coupling is where the magic truly happens. Designing an efficient inductive coupling circuit involves focusing on the geometry of coils, frequency of the applied current, and the load characteristics. Getting this design right is essential for achieving effective energy transfer and minimizing losses.
Coil parameters, such as number of turns, radius, and placement, directly affect the inductive coupling coefficient. For instance, the closer the coils are, the greater the efficiency, while air or non-magnetic materials between them can impede energy transfer.
When constructing these circuits, it's also crucial to choose the appropriate resonant frequency. Resonance enhances the energy transfer by aligning the input frequency with the natural harmonic of the circuit. Consequently, an underdamped circuit could lead to losses, while overdamped circuits can stall energy transfer altogether.
Points to Consider
- Exact coil design can significantly affect performance.
- Consideration of surrounding materials and environment is crucial.
- Frequency tuning allows maximization of energy transfer.
By dissecting these principles, we can appreciate their implications across different applications. Inductive coupling is not just a technical concept; it embodies the intricate dance between physics and technology, enabling feats that have real-world implications.
Inductively Coupled Plasma (ICP)
Inductively Coupled Plasma, often referred to simply as ICP, is a foundational technology in various fields, most notably in analytical chemistry. Its importance cannot be understated, as it provides a means to analyze materials at extraordinary sensitivity levels. This is particularly relevant in a world increasingly focused on precision and accuracy in measurements. ICP functions through the generation of an ionized gas, which plays a crucial role in the detection and analysis of elements within samples.
With broad applications ranging from trace element analysis to its usage in environmental monitoring, this method stands out for its ability to process complex samples with remarkable efficiency and speed. Understanding its mechanisms and applications is crucial for professionals in scientific research and industry.
Mechanism of ICP Generation
The generation of ICP relies on principles of electromagnetic induction and gas-ionization. Initially, an inert gas, usually argon, is introduced into a coil where a high-frequency alternating current is passed. This generates a magnetic field, heating the gas to a point where it becomes ionized. During this ionization process, the gas transforms into a plasma state, which consists of free electrons, ions, and neutral particles.
The effective heating of the gas allows the plasma to reach very high temperatures, typically around 6000 to 8000 Kelvin. This elevated temperature is essential as it assists in the excitation of atoms in the sample, which can then emit light at characteristic wavelengths. The emitted light is captured and quantified to identify and measure the elements present in the sample, offering valuable insights into its composition.
Applications in Analytical Chemistry
Trace Element Analysis
Trace element analysis refers to the determination of minute quantities of elements within a given sample, often in the parts-per-million or less range. This technique is pivotal in various sectors such as mineralogy, pharmaceuticals, and environmental studies. One of the key characteristics of trace element analysis is its sensitivity β enabling scientists to detect elements that otherwise would go unnoticed through traditional analytical methods.
Its benefits are many; for instance, ICP can analyze multiple elements simultaneously, saving time and resources. The unique feature of trace element analysis using ICP is its capacity to provide accurate results for complicated sample matrices. However, one must consider potential interferences in high complex samples which may affect the reliability of the results.
Environmental Monitoring
Environmental monitoring encompasses the routine assessment of pollutants and other contaminants in the environment, crucial for maintaining ecological balance and public health. The use of ICP in this context is highly valued; it allows for rapid analysis of heavy metals and other harmful elements in air, soil, and water samples.
One standout characteristic is its capability to handle diverse matrices, making it a beneficial choice for environmental scientists. Moreover, ICPβs high throughput nature means that it can process numerous samples quickly, addressing the urgent need for timely environmental data. The notable advantage here includes the high accuracy of readings compared to other methods. On the flip side, one limitation is that sample preparation can be labor-intensive, requiring careful handling to minimize contamination risk.
Limitations of ICP Techniques
Despite its advantages, ICP techniques come with limitations that must be acknowledged. One major challenge stems from the requirement for sample preparation, as high purity reagents and contamination-free environments are paramount to ensure precise results. Additionally, the cost of equipment and maintenance can be a barrier for some laboratories, particularly smaller ones with limited budgets. Furthermore, while ICP is capable of detecting a wide range of elements, there are some instances where complex organic matrices may pose difficulties in analysis, leading to potential inaccuracies.
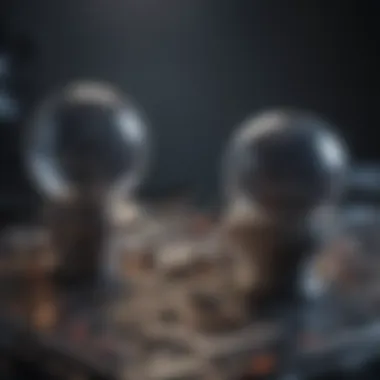
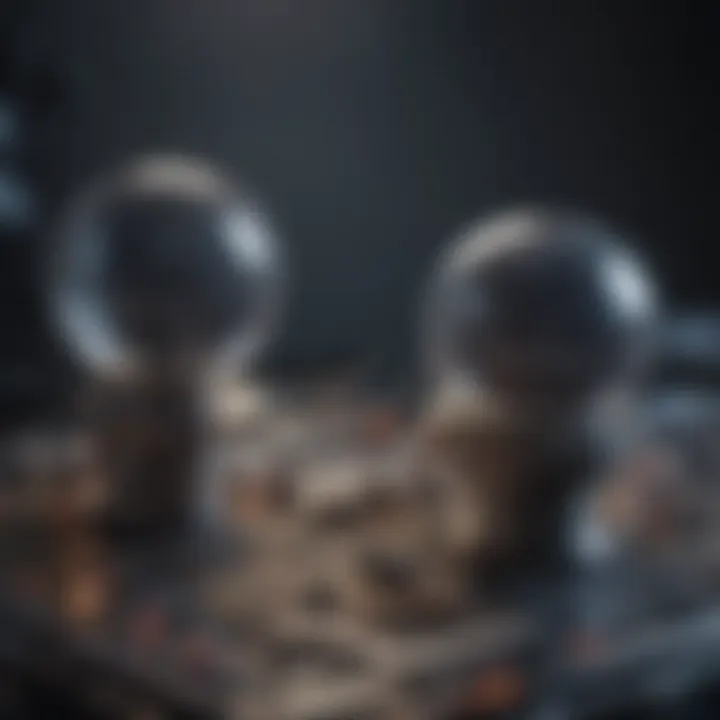
Through a deep dive into these elements surrounding ICP, one can better appreciate the significance it holds in advancing analytical chemistry and its broad applicability across various sectors.
Inductive Coupling in Telecommunications
Inductive coupling has transformed how we think about telecommunications, creating pathways for more efficient operations and reducing physical connections that were once essential for communication. This section elaborates on how inductive coupling facilitates advancements in two areas: wireless power transfer and data transmission technologies. The importance of these topics cannot be understated; they help streamline energy use and enhance the speed and reliability of data exchange.
Wireless Power Transfer
Wireless power transfer (WPT) is one of the standout applications of inductive coupling. In this system, power is transferred through inductive means without needing direct contact. The concept is as straightforward as it soundsβan electromagnetic field generated by a coil induces a current in another coil placed within the field's reach.
- Efficiency: Many technological devices use WPT to enable easier and more effective charging solutions. For instance, devices like smartwatches and electric toothbrushes can charge simply by placing them on a pad.
- Convenience: WPT also eliminates complexities associated with cables, allowing for cleaner and more efficient setups. Think about the mess of cords in your living space; WPT tackles that issue head-on.
- Safety: Moreover, WPT provides safety advantages, particularly in environments where traditional wires might pose risks, such as with children or in wet areas. It minimizes the chances of electric shock or short circuit.
Nevertheless, while the benefits are compelling, it is essential to note that wireless power transfer does come with some drawbacks. The efficiency can lessen due to distance, and the technology requires precise alignment for optimal performance.
Data Transmission Technologies
Inductive coupling also plays a critical role in various data transmission technologies. The capacity to send information through inductive links adds a level of flexibility previously unattainable.
- Magnetic Induction: Systems employ magnetically induced fields to transfer data between units. Here, the data packets are modulated onto the electromagnetic waves, transmitted, and demodulated at the receiving end, ensuring that the information reaches its destination intact.
- Near Field Communication (NFC): This technology makes use of inductive coupling to facilitate quick exchanges of information, especially in payment systems. The beauty of NFC lies in its simplicity; you just have to hold devices close together, enabling fast transmission without complicated configurations.
- Potential Applications: Data transmission through inductive coupling can be utilized in smart home devices, automotive technologies, and healthcare devices, connecting systems wirelessly in real-time. This opens doors for interconnectivity in an increasingly digital world.
"The true power of inductively coupled telecommunications lies in its ability to connect devices without physical boundaries, enabling a seamless flow of energy and information."
In summary, the realm of telecommunications, when enhanced by inductive coupling, represents a shift toward a more efficient and interconnected future. Though challenges exist, the strides made in wireless power transfer and data transmission technologies show significant potential for the coming years.
Inductively Coupled Accelerators
Inductively coupled accelerators have become a cornerstone in advancing particle physics and contributing to our understanding of fundamental forces and particles. These devices utilize electromagnetic induction principles to accelerate charged particles, offering unique advantages that traditional methods often cannot match. This section examines various elements of inductively coupled accelerators, their operational principles, and their vital role within particle physics research.
Principles of Operation
At the heart of inductively coupled accelerators lies the principle of electromagnetic induction. In simple terms, these systems utilize oscillating magnetic fields created by an alternating current in a coil to induce an accelerating electric field. This alternating magnetic field interacts with charged particles, propelling them to high velocities. Such designs can be extremely efficient since they often eliminate the need for bulky and power-consuming components traditionally found in linear accelerators.
When a charged particle travels through this inducing field, it experiences a force proportional to its charge and the strength of the electric field, enabling it to gain energy continuously without the constraints usually imposed by physical structures. This principle allows for various configurations, leading to significant flexibility in design tailored for different experimental conditions or desired outcomes.
Role in Particle Physics Research
The significance of inductively coupled accelerators can be illustrated in two key areas of particle physics: high-energy physics and nuclear physics.
High-Energy Physics
High-energy physics concerns itself with the fundamental particles and forces that govern the universe. Inductively coupled accelerators stand out in this field due to their ability to achieve ultra-high energies efficiently. These accelerators can generate substantial kinetic energy, which is essential for exploring rare phenomena or producing exotic particles.
One distinctive feature of high-energy physics is its experimental nature, often relying on particle collisions to reveal underlying mechanisms of interaction. Since inductively coupled accelerators can be designed to accelerate particles to desired energy levels with minimal energy losses, they provide a cost-effective option for high-energy physics experiments, making them a popular choice within the scientific community.
"Inductively coupled systems provide unprecedented opportunities for high-energy explorations, opening doors to the unknown realms of particle interactions."
Nuclear Physics
Nuclear physics focuses on the interactions and behavior of atomic nuclei; it plays a crucial role in understanding both nuclear energy and nuclear reactions. Inductively coupled accelerators have made strides in this area by facilitating research in nuclear reactions, particularly in studying radioactive isotopes and fusion energy.
The notable aspect of nuclear physics research involving inductively coupled accelerators is the fine control over particle energy and momentum. By adjusting the operational frequencies and currents, researchers can fine-tune interactions at a nuclear level, allowing them to probe deeper into how matter behaves under extreme conditions. This specificity can lead to groundbreaking discoveries regarding nuclear stability and decay processes, which are vital to both theoretical physics and practical applications in energy production.
This targeted manipulation of particle interactions is a distinct advantage in inductively coupled systems, enabling researchers to maximize their experimental insights and minimize unwanted by-products of collisions that are often seen with different types of accelerators.
Advantages of Inductive Coupled Systems
Inductive coupling is not just a buzzword; it's a vital player in various technological advancements today. It's like the undercurrent, running quietly yet robustly, connecting a myriad of applications from analytical labs to telecommunications. The advantages here are not just surface-level perks but reflections of deeper benefits that can enhance efficiency, adaptability, and reach across numerous domains.
Efficiency and Flexibility
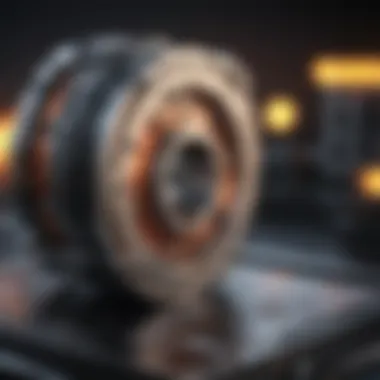
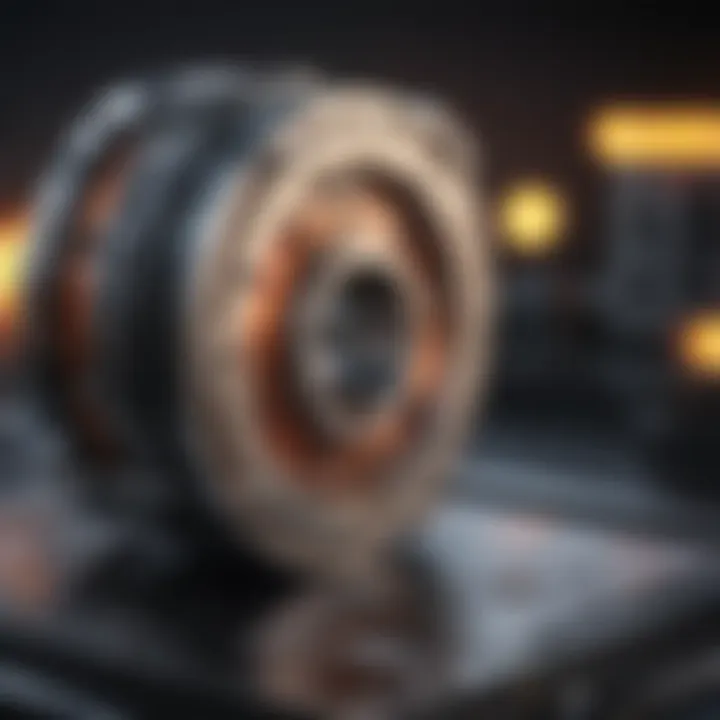
One of the standout features of inductively coupled systems is their efficiency. Initially, letβs consider how these systems perform in power delivery. The transfer of energy can occur without physical connections. Picture a phone charger; instead of fumbling with wires, you set your device on a pad, and voilΓ ! It charges wirelessly. This method, akin to throwing your laundry in the dryer without ever worrying about tangling your cords, reduces energy loss compared to traditional wired systems.
Beyond this, inductively coupled systems also boast flexibility in design and application. The adaptability of these systems means they can be tailored to fit countless needs. From handheld devices to large-scale industrial machinery, inductive coupling serves as a bridge, molding itself to fit various formats without sacrificing performance. Researchers exploring innovative methods have utilized these characteristics to push the envelope of whatβs possible in experimental setups, allowing them to manipulate conditions that once seemed out of reach.
- Applications in various fields:
- In analytical chemistry, for instance, flexible inductive systems enhance measurement accuracy in spectrometric techniques, efficiently adapting to different materials being analyzed.
- In telecommunications, inductively coupled systems facilitate seamless integration with modern smart technologies, ensuring robust data streams even amid interference.
Scalability Across Applications
When it comes to scalability, inductively coupled technologies truly shine. Whether in a lab or an industrial complex, these systems can be expanded or downscaled with relative ease. This means you can start small, experimenting with a new concept, and if it catches on, scale it up without needing to overhaul existing infrastructure.
Consider the following examples:
- A university research lab might initially deploy a small inductive plasma generator for testing new materials. If the results show promise, scaling the technology can be achieved by incorporating more powerful generators to increase throughput.
- Similarly, in the realm of wireless charging for electric vehicles, the scalability of inductively coupled systems allows manufacturers to adapt charging stations as demand grows without the need for extensive ground-up redesigns.
Utilizing inductively coupled systems not only maximizes potential in current applications but also permits future growth. The inherent compatibility with emerging technologies means that businesses and research entities are better equipped to pivot and advance alongside innovations in their fields.
"Inductive coupling is more than an innovative option; it's a strategic advantage that can propel industries forward, adapting swiftly to the evolving landscape of technological demands."
In summary, the advantages of inductively coupled systems extend beyond mere conveniences. Their efficiency and flexibility, alongside their impressive scalability, represent significant benefits that can be harnessed to tackle real-world challenges. As industries strive for greater productivity and adaptability, these systems will undoubtedly hold a pivotal role in shaping the future landscape of technology.
Challenges and Future Directions
As we look to the evolving landscape of inductively coupled technologies, the challenges we face are as critical as the technologies themselves. These issues not only influence current practices but also shape the trajectory for future innovations. In particular, understanding the complexities of these challenges paves the way for significant advancements and applications.
Technical Limitations
At the forefront of inductively coupled systems are several technical limitations that can hinder their effectiveness. Variability in performance associated with inductively coupled systems often stems from a few crucial factors:
- Frequency Interferences: Variations in the operating frequencies can lead to disruptions in the efficacy of power transfer or data transmission. This inconsistency can be particularly problematic in telecommunications where reliable results are paramount.
- Size Constraints: The physical dimensions of inductively coupled devices often present challenges, especially in environments where space is limited. Developing smaller, more compact systems without compromising performance remains a major hurdle.
- Material Limitations: Many materials exhibit poor performance with inductive coupling, primarily due to suboptimal electromagnetic properties. Finding suitable materials that enhance performance is an ongoing investigation in the field.
Overcoming these limitations demands innovation and cross-disciplinary collaboration. Researchers are actively experimenting with new materials and configurations to enhance efficiency, but these ventures can require extensive time and resources.
Research and Development Needs
With the above challenges in mind, there exists a pressing need for robust research and development in the field of inductively coupled technologies. Addressing the challenges requires a strategic focus on:
- Innovative Materials: Developing and synthesizing new materials that can conduct electricity more efficiently could be a game changer. Research on superconductors, for example, could yield breakthroughs that reduce energy losses in inductively coupled systems.
- Advanced Modeling Techniques: Improved simulation and modeling methods can help predict the behavior of inductively coupled systems under various conditions. By refining these techniques, researchers can better understand performance bottlenecks and address them before practical deployment.
- Interdisciplinary Approaches: Innovations often stem from the convergence of different fields. Encouraging collaboration between electrical engineering, materials science, and physics can foster new insights and accelerate the development of solutions that enhance inductively coupled technology applications.
"The future of inductively coupled technologies is bright, but it requires a clear eyed approach in recognizing and addressing its challenges."
As we advance, the collaborative efforts in research and development will prove to be indispensable. It will lay the groundwork necessary for the integration of inductively coupled technologies into a variety of real-world applications. The path forward may be riddled with challenges, but it also harbors immense potential for innovation and growth.
Ending
The conclusion serves as a pivotal part of this article, synthesizing the myriad threads of inductively coupled technologies and their applications. It encapsulates the importance of understanding these technologies, which stand at the nexus of progress in fields ranging from analytical chemistry to telecommunications. By bringing together the insights gained through previous sections, this part highlights how these systems function, their advantages and challenges, and why they matter in the contemporary scientific landscape.
Summary of Key Points
In this article, we explored several critical aspects of inductively coupled technologies:
- Definition and Historical Context: We laid the groundwork by defining inductive coupling and tracing its historical evolution, demonstrating its growing significance over time.
- Fundamental Principles: The article dissected the core principles, including electromagnetic induction, clarifying how they enable various applications.
- Applications in Diverse Fields: We examined specific instances of inductively coupled technologies, particularly in analytical chemistry and telecommunications, revealing their extensive utility.
- Benefits and Limitations: Notably, we acknowledged the efficiency and scalability of these systems while addressing the technical limitations and challenges that researchers face.
- Future Directions: Importantly, we pointed to ongoing research needs and developments that could enhance these technologies further.
This summary of key points underscores the multifaceted nature of inductive coupling and its profound relevance to both current practices and future innovations.
Implications for Future Research
Understanding inductively coupled technologies opens several avenues for future research:
- Enhanced Efficiency: Future studies could focus on optimizing the efficiency of inductively coupled systems, making them even more suitable for various applications.
- Innovations in Applications: There lies rich potential to explore new applications, particularly in emerging fields like nanotechnology and biomedical sciences.
- Addressing Technical Issues: A targeted approach to tackle the existing limitations will be essential for the advancement of these technologies. This includes enhancing the understanding of the limitations in data transmission and power transfer.
- Interdisciplinary Collaboration: Encouraging collaboration across disciplines can lead to groundbreaking applications of inductively coupled technologies, merging insights from physics, materials science, and engineering.
"The future of inductively coupled technologies hinges not only on overcoming present challenges but also on embracing innovative approaches that can redefine their applications and efficiencies."
In summary, the understanding of inductively coupled technologies is not just about appreciating established knowledge but also about fostering an environment where continued research can lead to extraordinary breakthroughs.