Thermal Properties of Alumina: Exploring CTE and Applications
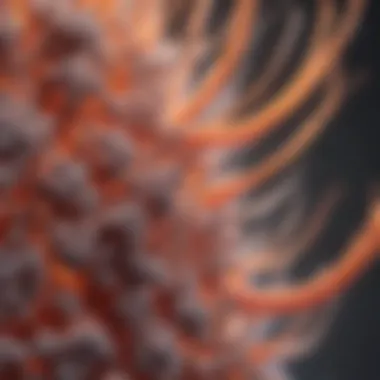
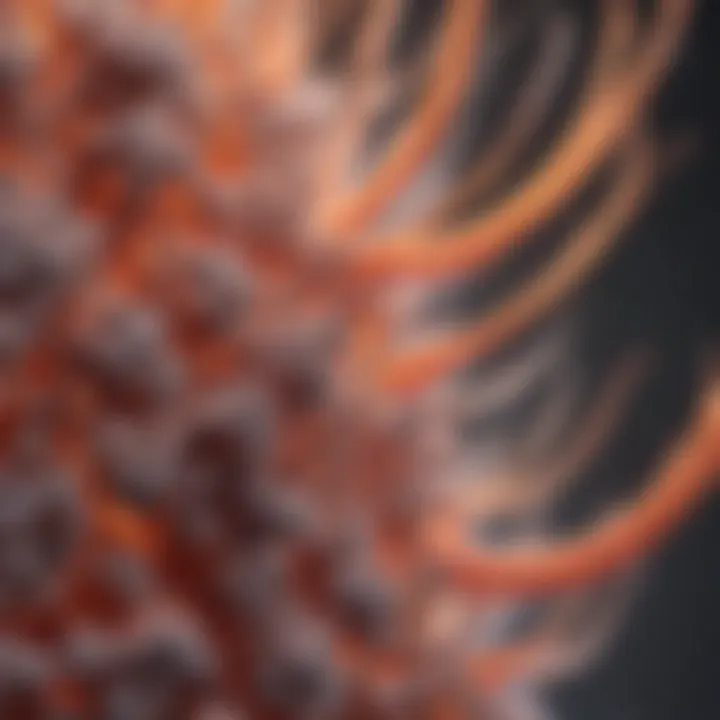
Intro
The study of materials science often brings to light fundamental characteristics that impact practical applications. One such property that stands out is the coefficient of thermal expansion (CTE) of alumina. This metric plays a significant role in numerous fields, influencing everything from the microchip industry to ceramics fabrication. A deeper understanding of how alumina expands and contracts with temperature variations can open doors to innovations that require precise thermal management.
Alumina, or aluminum oxide, is not only favored for its durability but also for its remarkable thermal properties.
In the upcoming sections, we will explore how the CTE of alumina affects its utilization in various industries, analyze the methodologies employed in measuring this coefficient, and delve into insightful case studies that illustrate both successes and challenges associated with alumina's thermal behavior.
Research Overview
Summary of Key Findings
Research indicates that the CTE of alumina varies based on its composition and structure. The findings highlight:
- A typical CTE range for alumina between 4.5 to 8.0 x 10^-6 /°C.
- Factors such as impurities, crystal structure, and temperature fluctuations impact the CTE significantly.
- Applications ranging from aerospace to electronics require specific CTE values to ensure compatibility with other materials.
These findings lay ground for understanding how variations in the CTE can lead to performance issues in specific applications, inviting further scrutiny into material selection and design.
Methodologies Employed
To obtain reliable data on the CTE of alumina, several methodologies have been utilized in recent studies:
- Dilatometry: Often the preferred technique, this method measures the expansion of a material as temperature varies, providing accurate readings of the CTE.
- Thermomechanical analysis (TMA): This technique assesses the dimensional stability of alumina under thermal stress, providing complementary data to dilatometric studies.
- X-ray diffraction: An advanced approach aimed at analyzing microstructural changes in alumina at different temperatures, shedding light on how these changes correlate with CTE variations.
By integrating results from these methodologies, researchers can build a coherent picture of how alumina behaves under thermal stress and develop strategies to leverage its strengths while mitigating potential drawbacks.
In-Depth Analysis
Detailed Examination of Results
A detailed examination reveals that the microstructure of alumina heavily influences its CTE. For instance, samples with smaller grain sizes typically exhibit a lower CTE compared to those with larger grains. This factor not only impacts performance but also poses challenges during the manufacturing process. In high-temperature applications, such as in jet engine components, even slight discrepancies in CTE can lead to catastrophic failures.
Comparison with Previous Studies
Comparing current research findings with earlier studies indicates progress in understanding the CTE of alumina. Previous work predominantly focused on theoretical models, whereas recent empirical approaches have provided concrete data that reaffirms or challenges existing theories. For example, while earlier studies suggested broader ranges for CTE, many contemporary investigations have narrowed these down, highlighting the importance of specific processing techniques.
"The coefficient of thermal expansion isn't just a number—it's a critical factor that interlinks design, functionality, and reliability across various disciplines."
This understanding is pivotal for engineers and researchers as they seek to innovate and optimize applications involving alumina and other materials that exhibit similar thermal properties.
Prolusion to Alumina
Understanding alumina is crucial for grasping its thermal properties, particularly the coefficient of thermal expansion (CTE). As a highly versatile material made primarily from aluminum oxide, alumina serves as a backbone in various industries, from ceramics to electronics. Its adaptability and thermal stability make it a significant subject of study. This section gives an insight into why alumina is so valued, touching on its composition and physical properties.
Chemical Composition
Alumina, chemically represented as Al₂O₃, is primarily composed of aluminum and oxygen atoms. The purity of alumina can vastly alter its thermal properties and overall performance. For instance, industrially produced alumina might contain various impurities such as silicon and iron, which can influence the CTE. When talking about its chemical traits, it’s not just the elements at play, but how they are arranged in the crystal lattice that matters.
This lattice structure can result in different polymorphs, each exhibiting unique thermal expansion behaviors. In high-purity alumina, the strong ionic bonds between aluminum and oxygen contribute to low thermal expansion, making it ideal for applications that require thermal stability.
Physical Properties
When discussing alumina, its physical properties leap into focus. These characteristics aren’t merely academic; they underpin much of alumina’s real-world usage. Alumina typically appears as a white powder or a crystalline solid, depending on its manufacturing and treatment process. Its hardness and durability are hallmarks that can be traced back to its structure. In fact, lame say it’s one tough nut to crack, with a Mohs hardness of about 9.
Thermal conductivity and electrical insulation are other important features. While alumina doesn’t conduct electricity, it does conduct heat, albeit at a moderate level. This makes it useful in applications that require both thermal stability and insulation from electrical currents. Additionally, alumina is resistant to chemical corrosion, which is a plus for it in harsh environments.
"Alumina's resistance to both thermal and chemical degradation makes it indispensable in many engineering contexts."
Its physical characteristics influence how it expands when heated, which brings us back to the significance of understanding CTE. This metric can ultimately dictate how alumina will interact with other materials in composite structures, making a deep dive into these properties not just interesting, but essential.
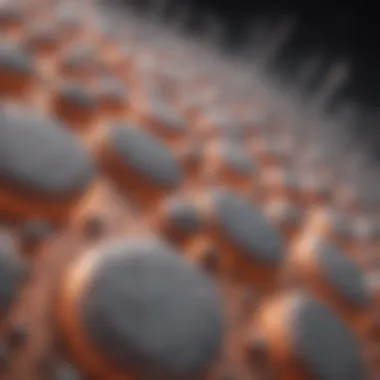
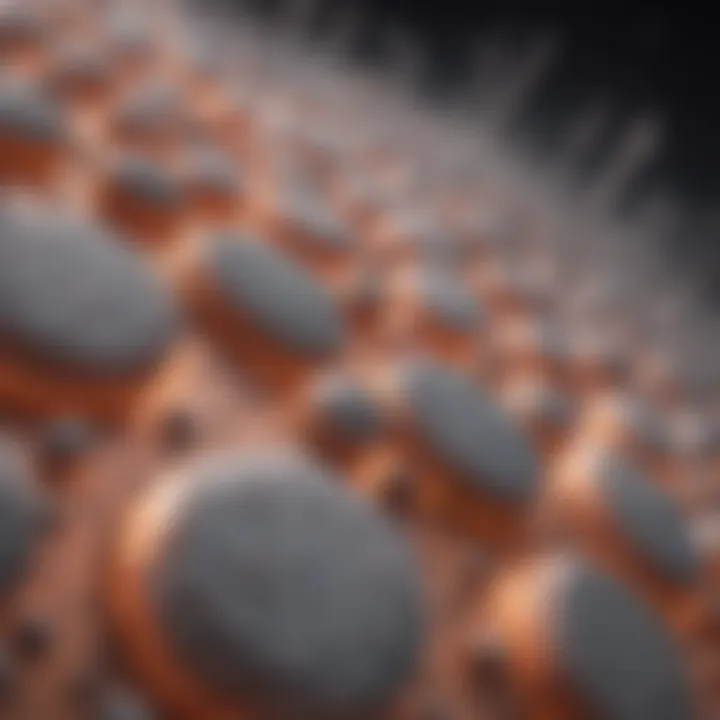
Understanding the Coefficient of Thermal Expansion (CTE)
Understanding the Coefficient of Thermal Expansion (CTE) is pivotal for grasping how materials react to temperature changes. This aspect becomes particularly relevant for alumina because its applications span a wide range of industries, from ceramics to electrical insulators. The significance of CTE lies in its role in predicting material behavior under thermal stress, which can lead to significant implications in design and manufacturing processes.
By examining the CTE, engineers and researchers can make informed decisions regarding material selection and processing methods that minimize risks of failure or inefficiencies.
Definition of CTE
The Coefficient of Thermal Expansion (CTE) quantitatively measures how much a material expands per degree of temperature increase. This property is usually expressed in units of length per length per degree Celsius (°C). Essentially, it tells us how much longer or wider a material will become as it heats up. For alumina, the typical CTE ranges from 7 to 8.5 x 10^-6 °C^-1 at room temperature, but this value can vary based on several factors, including purity and phase changes. Understanding CTE is crucial not just for theoretical considerations but also for practical applications where temperature changes are commonplace.
Importance of CTE in Materials
The importance of CTE in materials can hardly be overstated, especially in a world dealing with complex thermal environments.
- Structural Integrity: Materials often encounter fluctuations in temperature during their operational life. For alumina, a high or low CTE can lead to thermal stress that compromises structural integrity. This can provoke cracks or unwanted deformation.
- Joining and Assemblies: When joining alumina with other materials, differences in thermal expansion can cause issues. Inconsistent CTEs may result in bonds failing due to stress during heating or cooling cycles. Therefore, knowledge of CTE is essential when choosing materials for assemblies.
- Thermal Shock Resistance: Many applications demand that materials withstand rapid temperature changes. In such cases, understanding the CTE of alumina helps in assessing its thermal shock resistance and suitability for specific applications.
- Manufacturing Processes: When designing manufacturing processes involving alumina, considering its CTE helps tailor temperature regulation accordingly to ensure quality control and prevent defects.
"The CTE is a fundamental property that influences not just physical characteristics but also applications across a variety of fields, laying the groundwork for effective material utilization."
In summary, the Coefficient of Thermal Expansion is more than just a number; it is a crucial aspect influencing material performance, influencing everything from everyday products to sophisticated technologies.
Measuring CTE of Alumina
When it comes to alumina and its applications, understanding how to measure the coefficient of thermal expansion (CTE) is of paramount importance. The CTE is not merely a number; it reflects how materials behave under temperature changes. This has significant implications for the reliability and performance of alumina in various settings, from industrial manufacturing to advanced scientific research. Accurate CTE measurements help engineers and researchers predict how alumina will react when exposed to varying temperatures, which in turn allows for better design choices and enhances material longevity. Without accurate measurements, one risks unexpected failures or performance issues in their applications.
Techniques Used for Measurement
To gauge the CTE of alumina effectively, several techniques are employed, each bringing its unique strengths and weaknesses to the table. Understanding these methodologies can not only simplify the measurement process but also enhance the accuracy of CTE assessments.
Thermomechanical Analysis
Thermomechanical Analysis (TMA) stands out as a favored method for measuring the CTE of alumina. This technique involves subjecting the material to a controlled temperature change while monitoring any dimensional changes. One of the core advantages of TMA is its precision; it can detect minute changes in length even as temperatures fluctuate.
Another characteristic of TMA is its ability to deliver insight into the viscoelastic properties of alumina. This provides an added layer of understanding regarding how the material behaves under different thermal conditions. While TMA is incredibly beneficial, the process requires calibration and a skilled operator to ensure results are trustworthy. Mismatches in calibration can lead to inaccuracies.
X-Ray Diffraction
X-Ray Diffraction (XRD) is a powerful tool often used in crystallography, but it also plays a vital role in measuring the CTE of alumina. This technique analyzes how X-rays interact with the material's internal structure, allowing researchers to determine changes in lattice parameters as temperature varies. The key feature of XRD is its non-destructive nature, making it ideal for obtaining reliable data without compromising the sample's integrity.
Despite its benefits, XRD does have its limitations; it can be less effective for polycrystalline samples than for single crystals. Also, interpreting the data could require expert knowledge, rendering it a complex approach for some.
Interferometry
Interferometry offers another high-precision technique for measuring the CTE of alumina. This optical method takes advantage of the interference patterns created by light waves, which can reveal even the smallest changes in length due to thermal expansion. One of the defining characteristics of interferometry is its ability to achieve extreme accuracy, enabling researchers to detect changes in the order of nanometers.
The beauty of interferometry lies in its speed; it can provide real-time measurements without the need for complex setups. However, this method can be sensitive to environmental factors such as vibrations, which could potentially skew the results if not adequately controlled.
Factors Affecting Measurement Accuracy
Measurement of the CTE does not come without its challenges. Several factors can affect the accuracy of results, making it essential to pay close attention to details. Some of these factors include:
- Sample Preparation: The way alumina is prepared prior to measurement can significantly influence results. Imperfections or inconsistencies can introduce errors.
- Temperature Control: Fluctuations in temperature during tests can lead to inaccuracies. Ensuring a stable temperature environment is crucial.
- Environmental Interference: External conditions such as humidity and vibration can affect measurement techniques like interferometry.
To mitigate these challenges, meticulous experimental setups and protocols should always be prioritized. Gathering data and understanding its limitations will aid in achieving the most accurate outcomes possible, lending credibility to the process.
Factors Influencing CTE of Alumina
Understanding the factors that affect the coefficient of thermal expansion (CTE) of alumina is key in various fields, such as materials science and engineering. CTE is a critical property that not only affects how materials behave under thermal stress but also has significant implications for design and application in real-world situations. When considering alumina, a ceramic material known for its versatility and robustness, it becomes crucial to analyze how specific elements such as temperature and chemical composition influence its thermal expansion behavior.
Temperature Dependence
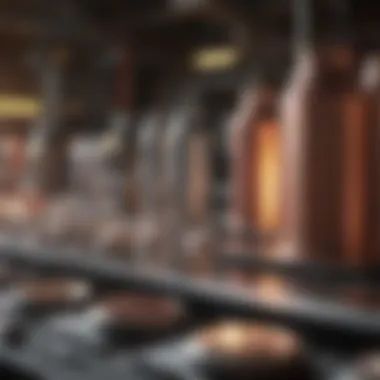
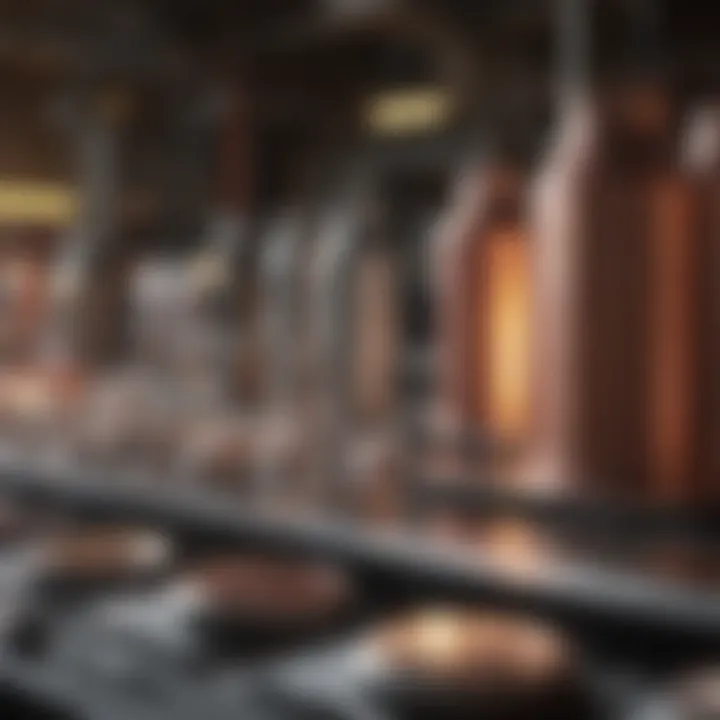
The CTE of alumina is not a fixed value; it varies with temperature. This dependency showcases the dynamic nature of materials under varying conditions. Typically, as the temperature rises, materials tend to expand more. However, alumina exhibits a more complex behavior, which can be attributed to the crystal structure and bonding forces within the material. Understanding this temperature dependence assists engineers and researchers in predicting how alumina components will behave in situations where temperature fluctuations are common, such as in processing equipment or aerospace applications.
According to recent studies, CTE values for alumina can change significantly across different temperature ranges, making precise measurements essential for applications requiring thermal stability.
By recognizing the relationship between temperature and CTE, one can better design systems that utilize alumina in a way that minimizes stress and enhances durability.
Chemical Composition Variations
Alumina is not a single entity; it can possess various chemical compositions depending on the impurities present and the synthesis methods used. These variations will significantly influence its CTE.
Impurities
Impurities refer to the unintended elements or compounds that become mixed with alumina during its synthesis. The presence of these impurities can modify the thermal expansion properties significantly. This is because impurities can disrupt the crystalline lattice of alumina, causing more variability in its thermal behavior. One key characteristic of impurities is that they can either enhance or reduce the CTE depending on their nature and concentration.
For example, the inclusion of materials such as silica can have a moderating effect on CTE, creating a more stable structure. However, too much impurity may lead to defects, which often results in erratic thermal expansion responses. Understanding the role of impurities is crucial for achieving desired qualities in alumina, especially for critical applications requiring precise thermal properties.
Phase Transitions
Phase transitions in alumina can also affect CTE. When alumina undergoes a transition from one crystalline form to another—such as from alpha-alumina to gamma-alumina—its thermal expansion properties can shift drastically. This shift is notable, as the phase transition can signify a change in bonding characteristics and symmetry of the crystalline structure.
A unique feature of phase transitions is the potential for altering thermal management strategies in applications. For instance, the ability to control or predict phase transitions offers the opportunity for engineers to design better thermal barrier coatings for aerospace components. However, reliance on phase transitions must be tempered with caution, as they can introduce instabilities and surprises in CTE that may not be anticipated.
In summary, both temperature dependence and chemical composition variations—including impurities and phase transitions—play vital roles in influencing the CTE of alumina. Addressing these factors can significantly enhance practical applications, making alumina a material of choice in many high-tech industries.
CTE in the Context of Alumina Applications
Understanding the coefficient of thermal expansion (CTE) in the realm of alumina applications unfolds a significant chapter in materials science. The CTE signifies how much a material expands or contracts with changes in temperature, and this property is particularly vital for alumina due to its widespread usage in various domains. As industries integrate alumina into different applications, awareness of CTE instigates a deeper comprehension of potential challenges and advantages that arise.
Throughout this section, we will touch on a few key areas where alumina’s CTE is particularly impactful, underscoring its importance in both industrial and scientific realms.
Industrial Applications
Ceramics
Delving into the world of ceramics, alumina stands tall as a preeminent choice. The key characteristic of ceramics is their ability to withstand high temperatures while maintaining stability. When it comes to alumina ceramics, their low CTE means they can endure temperature fluctuations without significant deformation. This is vital in applications such as kiln furniture and dental prosthetics, where structural integrity must be preserved under varying thermal conditions. The unique feature of alumina ceramics is their hardness, which translates to better wear resistance. However, while they shine in durability, they can be a tad brittle, posing challenges in environments with sudden impacts.
Refractories
Shifting focus to refractories, alumina's role becomes increasingly crucial. Refractories are often tasked with handling extreme heat in industries like metal casting and glass production. Their main advantage lies in their thermal stability and resistance to thermal shock, both influenced by CTE. This characteristic allows refractories to perform efficiently even when temperatures swing wildly. Alumina refractories are also valued for their chemical resistance. Additionally, the high melting point of alumina ensures that these materials remain effective at high operational temperatures, though they may lack some mechanical strength compared to other materials under specific conditions.
Electrical Insulators
In electrical applications, the utilization of alumina is celebrated for its insulating properties. Electrical insulators made from alumina exhibit a relatively low CTE, which prevents failure due to thermal expansion when subjected to heating cycles, as seen in power plants or transmission lines. This characteristic is a boon for ensuring longevity in electrical component life. Furthermore, alumina’s high dielectric strength adds to its appeal. Nevertheless, the challenge here is maintaining cost-effectiveness, as high-purity alumina can drive expenses up significantly.
Scientific Research Applications
Nanomaterials
Transitioning to scientific endeavors, alumina’s CTE is critical in the development of nanomaterials. The fascinating aspect of nanomaterials is their unique properties that evolve from their small-scale structures, with thermal expansion becoming an essential factor in their prediction and manipulation. The relatively stable CTE of alumina-based nanomaterials allows researchers to explore various applications, from drug delivery systems to energy storage solutions. The notable advantage here is their lightweight and strength. However, fine control over size and uniformity can be challenging, potentially complicating large-scale manufacturing.
Thermal Barrier Coatings
In the realm of high-temperature aerospace and automotive applications, thermal barrier coatings (TBCs) made from alumina stand pivotal. The essential characteristic of these coatings is their ability to withstand extreme temperatures, protecting substrates from heat damage. Their low CTE allows TBCs to expand and contract with the materials they're protecting without leading to cracks or material fatigue. This feature proves advantageous during intense thermal cycling, as seen in jet engines. Still, the longevity of these coatings can wane if not properly engineered to adhere to various substrates, which is one consideration for further research and development in TBC applications.
"The coefficient of thermal expansion is not just a number; it’s a key to understanding how materials behave under heat, influencing choices across industries."
Through the lens of both industrial and scientific applications, the coefficient of thermal expansion for alumina emerges as a vital property. It influences everything from production processes to everyday usages, shaping innovations, and driving future trends.
Challenges Related to CTE in Alumina
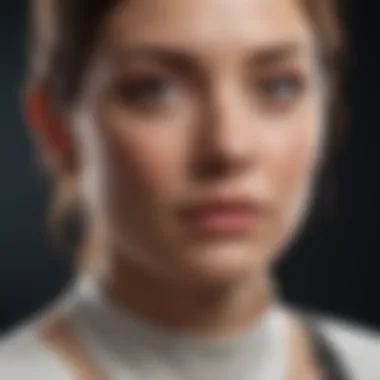
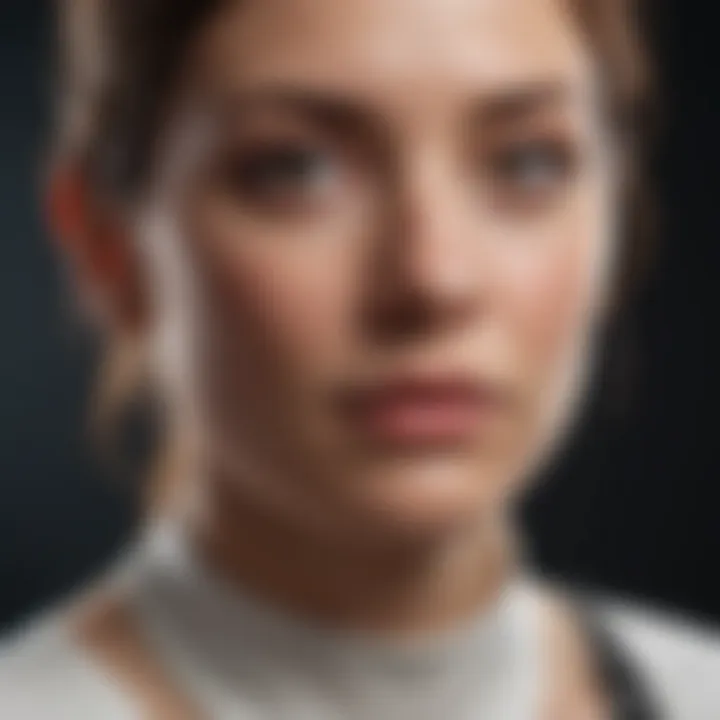
Understanding the coefficient of thermal expansion (CTE) in alumina presents numerous challenges that are deeply intertwined with the material's performance in practical applications. These challenges can affect how alumina is used in various industries, making it imperative to consider them thoroughly. Not only do thermal stresses arise from temperature variations, but compatibility between alumina and other materials also proves to be a critical concern. Both of these factors can dictate the longevity and reliability of products made with alumina.
Thermal Stress and Material Failure
Thermal expansion is a natural occurrence where materials change shape or size when subjected to varying temperatures. For alumina, this behavior can lead to thermal stress, especially in configurations where different components experience divergent expansion rates. This is crucial because thermal stress can lead to cracking or failure of alumina parts, which is detrimental in high-stakes environments, including aerospace and electronics.
When the temperature fluctuates, one can imagine alumina components expanding at differing rates, much like an overcooked piece of pizza melting unevenly in the oven. If one section expands more than another, it creates tension that can lead to fractures—or worse, catastrophic failure. Thus, understanding the peculiarities of thermal stress in alumina is essential for engineers who design parts subjected to such conditions. Choosing the right form of alumina and taking precautions in design can help mitigate these issues.
"Material failure often originates from changes in temperature that were not adequately considered during the design phase."
Compatibility with Other Materials
In today's multifunctional designs, materials are often bonded or together, creating joints where alumina may meet metals, polymers, or ceramics. This raises another set of challenges related to compatibility. As different materials expand at different rates, mismatches can lead to stress concentrations at the interfaces. If not accounted for, these mismatches can raise havoc, potentially leading to delamination, warping, or even fractures in the structure.
For instance, when alumina is used alongside metals in circuit boards, the differential expansion during heating and cooling processes can generate significant stress. This often influences the durability and performance of electronic components. Engineers need to carefully consider material choices and design approaches, perhaps employing transition materials or using adhesives designed to compensate for these different CTEs.
Innovations and Future Trends
The landscape of material science is ever-evolving, and alumina is no exception. It’s crucial to be aware of the latest innovations and potential future trends that could shape the usage of alumina across various industries. Understanding these progressions pushes the boundaries of how we can utilize alumina more effectively, particularly focusing on its thermal properties, specifically the coefficient of thermal expansion (CTE).
Advancements in Measurement Techniques
Recent strides in measurement techniques have significantly enhanced the accuracy and reliability of CTE evaluations for alumina. Traditional methods like thermomechanical analysis and X-ray diffraction are becoming increasingly sophisticated.
- High-Resolution Instruments: The introduction of high-resolution interferometry systems allows for more precise measurements. This means any subtle changes in thermal expansion can be captured with much greater detail, aiding manufacturers in developing materials that can withstand extreme temperature variations without sacrificing integrity.
- Integrative Technologies: Systems that integrate different measurement techniques provide a comprehensive approach to assessing alumina's thermal properties. When thermomechanical analysis is combined with non-destructive testing methods, it offers not just a snapshot of the material’s behavior under heat but also insights into how changes manifest over time in actual use conditions.
These advancements help researchers and manufacturers refine their designs, making alumina not only a versatile choice but also more reliable in high-stress applications.
New Applications in Emerging Technologies
As industries shift towards more innovative solutions, the applications for alumina are expanding in intriguing directions. Two significant sectors stand out: electronics and biomaterials.
Electronics
Electronics rely heavily on materials that can handle varying heat levels without compromising functionality. Alumina, with its favorable thermal expansion properties, plays a vital role in this sector.
- Key Characteristic: Its excellent thermal conductivity coupled with low CTE makes it a prime candidate for substrates in high-performance electronic components. Rather than risking failure during thermal cycling, the stability that alumina affords is a major asset.
- Unique Feature: The durability of alumina against thermal shock sets it apart from alternatives such as polymer-based substrates. Its resistance to warping or structural failure when exposed to extreme temperatures enhances the longevity of electronic devices.
- Advantages: The integration of alumina in advanced electronics provides benefits like enhanced performance and greater operational safety. However, challenges remain; the cost of production and processing of alumina can be high, which may limit its widespread adoption.
Biomaterials
The application of alumina in the context of biomaterials opens a different yet equally fascinating avenue. Its unique attributes make it a compelling choice in medical devices, such as implants.
- Key Characteristic: Alumina's bioinertness allows it to be safely used in the human body without eliciting adverse reactions. This characteristic is paramount when considering materials for implants that must integrate seamlessly with biological systems.
- Unique Feature: The strength and hardness of alumina impart mechanical reliability in load-bearing applications within the body. Unlike metals, which may corrode or degrade over time, alumina maintains its integrity.
- Advantages: The long-term stability of alumina offsets the material’s typically higher initial costs, especially given the benefits in longevity and compatibility. Yet, it comes with challenges, such as the difficulty in working with alumina in forming intricate shapes for complicated surgical applications.
In summary, the future for alumina looks bright, especially in its evolving measurement techniques and burgeoning applications across electronics and biomaterials. Keeping a finger on the pulse of these trends will be essential for maximizing its potential and addressing the challenges that come along.
Concluding Remarks
The conclusion of this article serves as a pivotal moment to encapsulate what we have explored regarding the coefficient of thermal expansion (CTE) of alumina. In understanding the thermal properties of this versatile material, we notice how CTE is not just a number; it’s a critical factor that impacts the reliability and performance of alumina in various applications. The relevance of comprehending CTE cannot be understated, as it governs how alumina responds to temperature changes during its lifecycle.
Summary of Key Points
- Definition and Role of CTE: Throughout the article, we delved into what CTE truly represents within the context of alumina. It quantifies how much the material expands or contracts with temperature shifts. This trait is vital for engineers, particularly when designing components that must maintain structural integrity during thermal cycling.
- Measurement Techniques: We examined several methodologies for accurately measuring CTE. Techniques such as thermomechanical analysis, X-ray diffraction, and interferometry were discussed, all of which enable precise evaluation amid varying conditions and material formulations.
- Influencing Factors: The temperature dependence and the chemical composition of alumina play substantial roles in affecting CTE values. Factors like impurities or phase transitions can substantially alter performance, necessitating careful selection and quality control in alumina applications.
- Application Insights: The applications of alumina stretch across numerous industries, from ceramics to electronics. In each sector, the implications of CTE are profound, influencing how products are manufactured and their long-term reliability in service.
- Challenges and Innovations: We highlighted the challenges involved in dealing with thermal stress and compatibility with other materials. However, there are ongoing innovations that address these concerns, paving the way for improved applications in emerging technologies.
Implications for Further Research
The field of material science is ever-evolving. Continuous exploration into the thermal properties of alumina, particularly CTE, is critical. Here are some aspects worth considering for future studies:
- Extended Range of Conditions: Investigating how alumina behaves under more extreme temperature ranges can yield valuable data for specific applications, such as aerospace or automotive sectors, where performance under stress can differ significantly.
- Nanostructuring Effects: The application of nanoparticle inclusions may alter CTE in innovative ways. Understanding these effects will improve the design of next-generation materials that harness the benefits of both alumina and nanotechnology.
"By pushing the boundaries of how we perceive and utilize alumina, we can enter realms previously deemed impossible in material science."
- Integration with Smart Technologies: With the rise of smart materials, the intersections between CTE and responsive design are ripe for research. Future studies should seek to explore how alumina can be modified for applications that require adaptive thermal properties.
In summary, alumni's thermal properties, especially the CTE, open a plethora of pathways for exploration. As the demand for novel applications grows, so too does the need for a deeper understanding of the pivotal role CTE plays in alumina’s performance and versatility.