In-Depth Exploration of Stable Transfection Techniques
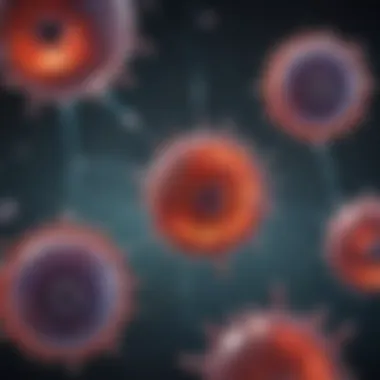
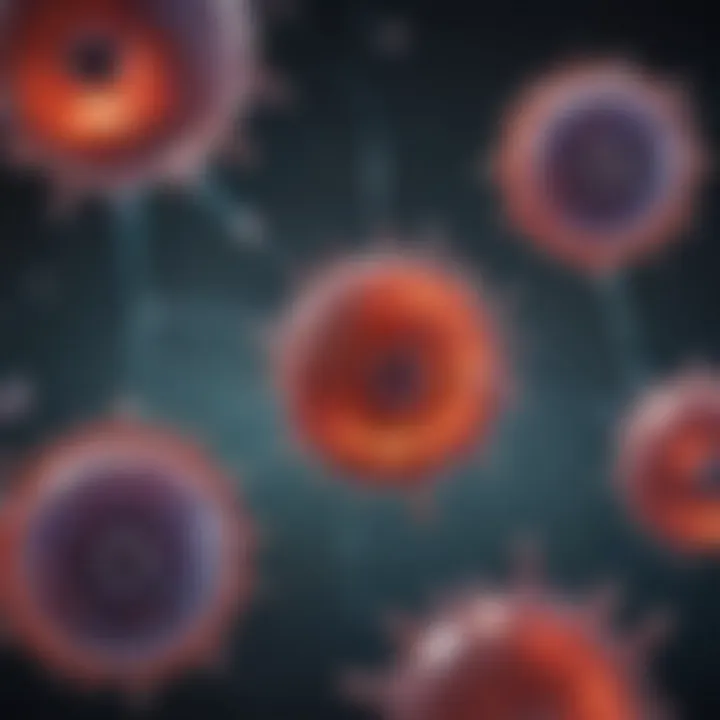
Intro
Stable transfection is a fundamental technique in the fields of molecular biology and biotechnology. By allowing the introduction of foreign DNA into host cells, this method plays a crucial role in research and therapeutic applications. Researchers can manipulate gene expression, study cellular processes, and produce recombinant proteins, making stable transfection a cornerstone of modern biological studies.
Stable transfection is distinct from transient transfection, where introduced DNA typically only persists for a short time. The key to stable transfection lies in the ability of the transfected DNA to integrate into the host cellβs genome, allowing for lasting expression and manipulation. It's like planting a seed that can grow into a sturdy tree rather than just a flower that blooms for a season. The stability of gene expression over time opens up numerous possibilities for research and development in various domains.
As we delve into this topic, we'll explore various methodologies, examine challenges that researchers face, and highlight the importance of stable transfection in gene expression studies and therapeutic advancements. Each section will unpack intricate details to empower students, researchers, educators, and professionals to harness this potent technique effectively.
Preface to Stable Transfection
Stable transfection is more than just a scientific technique; it represents a cornerstone in modern biological research and biotechnology. By enabling the enduring introduction of exogenous DNA into host cells, this method not only advances our understanding of gene function but also drives several applications, from gene therapies to the production of vital proteins. Researchers and professionals alike find themselves increasingly drawn to stable transfection due to its potential to facilitate long-term gene expression, offering benefits that transient methods simply cannot match.
The importance of discussing stable transfection cannot be overstated, given its relevance in various fields, including molecular biology, pharmacology, and genetics. For instance, in drug discovery and development, utilizing stably transfected cell lines allows scientists to investigate therapeutic targets over extended periods. This ongoing expression of new genetic material provides critical insight into protein behavior, disease mechanisms, and much more. Moreover, in the realm of biologic therapies, establishing a steady supply of therapeutic proteins or even cell lines that replicate specific conditions is invaluable.
Definition and Importance
Stable transfection is defined as the process by which foreign DNA is integrated into the chromosomal DNA of a host cell, resulting in a permanent change to the cell's genetic makeup. This integration can lead to continuous expression of the foreign gene, which can be crucial for experimental assays and therapeutic applications. Notably, the importance of stable transfection lies in its ability to create a cell line that expresses a specific gene longitudinally, thus offering a stable platform for studying gene function and protein interactions.
One of the distinct advantages of this technique is that it enables the analysis of genetic regulation over time, revealing dynamic interactions that might be missed with transient transfection techniques, where gene expression is temporary. In a nutshell, stable transfection allows the researcher to create a βliving archiveβ of genetic modifications, making it easier to track changes and correlations in biological processes over extended periods.
Overview of Transfection Techniques
In the landscape of genetic engineering, several transfection techniques exist, each with particular strengths, weaknesses, and specific use cases. Understanding these methods is critical for researchers aiming to utilize stable transfection effectively. Here we will explore two broad categories of transfection techniques:
- Viral Vectors:
Viral vectors are commonly used for stable transfection due to their efficiency in delivering genetic material into cells. Techniques such as lentiviral transduction have become foundational, representing a versatile approach suitable for various types of cells, including those that are typically difficult to transfect. - Non-Viral Methods:
Non-viral methods are gaining traction, with various approaches showcasing different efficacies. Key techniques include:
- Calcium Phosphate Transfection: A classical method benefiting from simplicity, often used in a lab setting for DNA delivery.
- Lipid-Based Transfection: Utilizes lipid formulations that encapsulate DNA, promoting cellular uptake. This method is particularly valued for its versatility.
- Electroporation: A physical method involving the application of electrical fields, making cell membranes temporarily permeable to DNA. It's especially useful for hard-to-transfect cells but requires precise optimization.
Grasping these diverse transfection techniques and their particularities sets the stage for effectively employing stable transfection in research and development. As the pursuit of advanced methodologies continues, understanding what works best, given specific circumstances, is essential.
"In the evolving arena of molecular biology, stable transfection stands out as a beacon of innovation, bridging the gap between scientific inquiry and practical application."
This narrative not only highlights the definition and importance of stable transfection but also provides a comprehensive overview of the available techniques, setting a solid foundation for further exploration of mechanisms and applications in the upcoming sections.
Mechanisms of Stable Transfection
Understanding the mechanisms of stable transfection is crucial for leveraging this technique effectively in research and therapeutic applications. These mechanisms not only provide insights into how foreign DNA is integrated into host cells but also reveal the underlying considerations that impact transfection efficiency and stability. By grasping these mechanisms, scientists can optimize protocols and minimize challenges associated with transfection, ultimately enhancing experimental outcomes.
Cellular Uptake of DNA
The first step in stable transfection is the cellular uptake of DNA. This process can be likened to a key finding its lock; only when conditions are just right can the DNA effectively enter the cell. Various factors influence how efficiently DNA is taken up. For instance, the type of transfection method used plays a pivotal role. Methods may include calcium phosphate precipitation, lipid-mediated transfection, or electroporation. Each method has its nuances, impacting how effectively the DNA can breach the cell membrane.
Furthermore, the properties of the DNA itself make a difference. Factors such as the size of the DNA molecule, its conformation, and even the presence of modifications can affect its uptake. Interestingly, certain cell types are inherently more amenable to DNA uptake than others. For example, suspension cells often exhibit higher uptake rates compared to adherent cells due to less membrane obstruction. Thus, understanding the dynamics of cellular uptake is vital for researchers aiming to enhance transfection efficacy.
Integration into Host Genome
Once inside the cell, the journey doesnβt end. A critical aspect of stable transfection is the integration of the introduced DNA into the host genome. Itβs similar to laying down roots in a new gardenβif the roots are healthy and deep, the plant thrives. Through various integration mechanisms, the foreign DNA seeks a permanent place within the host's genetic framework.
There are several integration strategies at play. For example, some vectors like those derived from retroviruses can insert their genetic material into the host genome with high efficiency. This becomes particularly important when aiming for long-term expression of transgenes. However, while this method offers stability, it's not without risks, as it might disrupt essential genes or regulatory regions, leading to unintended consequences.
On the opposite end, non-viral methods typically result in temporary transfection unless specifically designed for integration. Making use of integrase enzymes or employing homologous recombination can improve the chances of successful integration. Overall, the consideration of how integration occurs can shape the choice of transfection strategy and influence the outcomes of experiments involving gene expression or therapeutic applications.
"Mastering the mechanisms of stable transfection is akin to understanding the rules of a complex game; without this knowledge, the chances of success diminish significantly."
In summary, both the cellular uptake of DNA and its subsequent integration into the host genome are integral mechanisms that underpin the success of stable transfection. By improving approaches in these areas, researchers can enhance the reliability and effectiveness of stable transfection for a myriad of applications, from gene therapy to industrial biotechnology.
Methods of Stable Transfection
Understanding the methods of stable transfection is critical for anyone diving into the realm of genetic modifications. These techniques serve as the backbone of various applications in research, such as gene expression, therapeutic development, and protein production. The successful execution of stable transfection not only relies on the choice of method but also on the specific goals of the experiment and the characteristics of the host cells.
Viral Vectors
Viral vectors are among the most efficient methods for stable transfection. They exploit the natural ability of viruses to deliver genetic material into host cells. The selection of the right viral vector depends on factors like cell type, desired level of expression, and long-term stability.
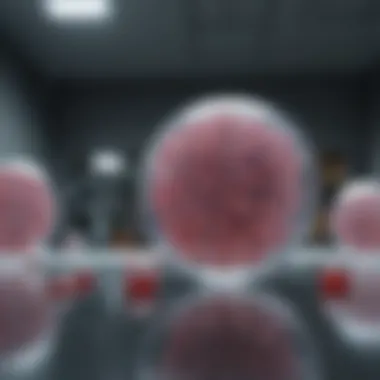
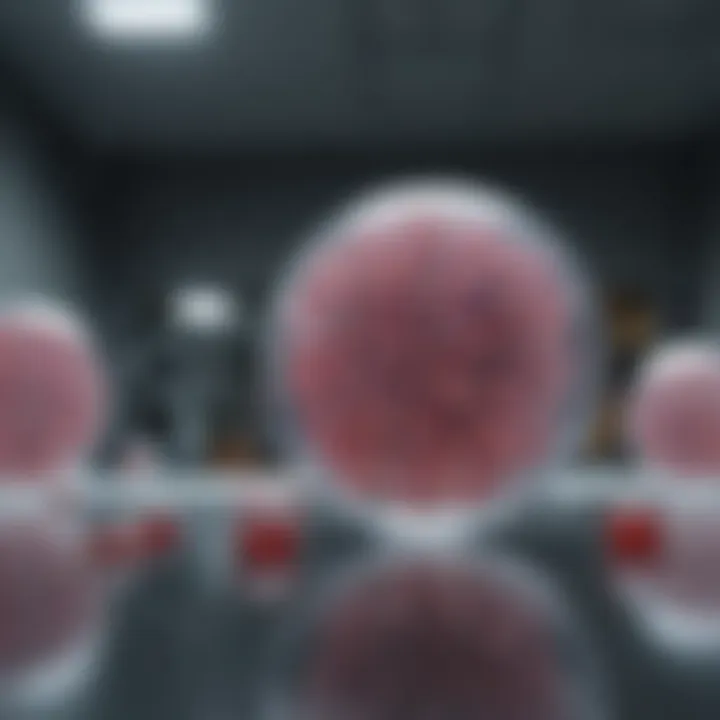
Common types of viral vectors include retroviruses, lentiviruses, and adenoviruses. These viruses can integrate into the host genome, ensuring stable expression across generations of cells. However, the safety concerns associated with using live viruses cannot be overlooked. The potential for insertional mutagenesis and immune responses poses significant challenges. Thus, a thorough understanding of virology and vector design is necessary to mitigate these risks.
Non-Viral Methods
Non-viral methods encompass a variety of techniques that allow researchers to introduce DNA into cells without using viral systems. They are increasingly popular for their relative safety and versatility. Hereβs a closer look at the most commonly used non-viral methods:
Calcium Phosphate Transfection
Calcium phosphate transfection is a classic method that has stood the test of time. It involves the formation of a precipitate with calcium phosphate, into which DNA is co-precipitated. When introduced into cells, this complex is taken up through endocytosis. The key characteristic of calcium phosphate transfection is its simplicity and low cost.
What makes it a favored choice is its efficiency in certain cell types, especially when optimized conditions are utilized. However, this method does come with its own set of challenges, such as suboptimal transfection rates in hard-to-transfect cell types, and its efficacy can be influenced by the cellular environment, requiring meticulous adjustments in procedures.
"Calcium phosphate transfection bridges the gap between simplicity and effectiveness, making it a staple in many laboratories."
Lipid-Based Transfection
Lipid-based transfection utilizes liposomes or lipid nanoparticles to encapsulate and deliver genetic material into cells. This approach relies on the fusion of the lipid bilayers with the cell membrane, thereby facilitating the entry of DNA.
One of the main attractions of lipid-based transfection is its versatility. It can be adapted for a wide array of cell types, including neuronal and primary cells. Additionally, these methods often lead to higher transfection efficiencies compared to some other non-viral methods. Nonetheless, the formulation optimization is necessary, as not all lipid types will work with every cell line, and toxicity can be a concern at higher concentrations of lipids.
Electroporation
Electroporation stands out as a physical method that employs electrical pulses to increase the permeability of the cell membrane. The electrical field transiently disrupts the membrane structure, enabling DNA molecules to enter the cell.
This method is particularly beneficial for hard-to-transfect cells and is widely used in primary cell cultures. The unique feature of electroporation is its effectiveness across a variety of cell types and its ability to handle larger DNA constructs. However, the critical aspect of electroporation is the optimization of electrical parameters. High voltage or improperly tuned conditions can lead to cell death and, thus, necessitate careful calibration.
Applications of Stable Transfection
Stable transfection plays a critical role in a variety of scientific disciplines, mainly because it offers a reliable method for introducing foreign DNA into host cells. This is particularly important for researchers looking to conduct long-term studies on gene function and regulation. The applications of stable transfection are not limited to a single field; instead, they span across various sectors in biology, biotechnology, and medicine, enabling advancements that impact numerous aspects of life sciences.
Gene Expression Studies
When it comes to gene expression studies, stable transfection is indispensable. Researchers often need to analyze how genes respond to different stimuli or how they interact with other cellular components. By integrating a specific DNA sequence into the genome of a host cell, scientists can create a constant expression system. This means that the gene of interest can be expressed continuously over time, allowing for repeated measurements and more robust conclusions.
For instance, a researcher might wish to study the behavior of a gene involved in cancer pathways. By stably transfecting a cell line with this cancer-related gene, they can observe its effects on cell growth, division, and apoptosis under various experimental conditions. The resulting data yields deep insights into the mechanisms by which this gene influences tumorigenesis, effectively paving new pathways for therapeutic options. Moreover, with a stable transfection system, the variability that might arise from transient methods is minimized, leading to more repeatable experimental outcomes.
Production of Recombinant Proteins
In the sphere of biotechnology, stable transfection is crucial for the production of recombinant proteins. These proteins are engineered to express a particular polypeptide with desirable traits, such as therapeutic proteins for disease treatment. Through stably transfected cell lines, large quantities of proteins can be harvested and purified for various applications, ranging from drug development to academic research.
One prominent example is in the production of monoclonal antibodies, which have become essential components in modern therapeutics for conditions like cancer and autoimmune diseases. Utilizing stable transfection methods allows researchers to maintain a consistent supply of specific antibodies. Not only does this enhance the understanding of their therapeutic benefits, but it also streamlines the process needed for clinical applications.
In addition to antibodies, enzymes and hormones can also be produced using stably transfected cell lines. This not only helps in scientific study but also serves commercial interests, thus bridging academia and industry's needs.
Gene Therapy Approaches
The implications of stable transfection reach into the realm of gene therapy, where it's employed to correct genetic disorders by delivering healthy copies of genes into patient cells. Given that many diseases result from malfunctions in genes, stably transfecting cells with therapeutic genes offers a straightforward strategy to redress these abnormalities.
For example, in certain genetic disorders like cystic fibrosis or hemophilia, stable transfection can be utilized to introduce functional copies of the defective gene into patient-derived cells. This permanent alteration can potentially lead to lasting corrections within the affected tissues, offering hope to those who suffer from inherited disorders.
However, while the potential is immense, so are the challenges. Ensuring that these gene-corrected cells thrive and perform optimally within the human body is a significant hurdle. Issues such as off-target effects and immune responses might arise, complicating the efficacy of these therapies. Despite these hurdles, ongoing advancements in stable transfection techniques continue to shed light on the practical applications and safety measures necessary for successful gene therapy.
Key Takeaway: Stable transfection is not merely a laboratory technique; it is a crucial gateway to advancements in gene studies, protein production, and groundbreaking gene therapy approaches. Through understanding and further improving these applications, researchers can unlock vast potential in medicine and biotechnology.
Challenges in Stable Transfection
Stable transfection is a complex procedure, and like any scientific method, it is not free from its own set of challenges. Understanding these obstacles is crucial for researchers aiming to harness the full potential of stable transfection techniques in their work. Acknowledging the difficulties allows for better planning and troubleshooting strategies. As the field moves forward, addressing these challenges becomes essential in refining methodologies and achieving more reliable outcomes.
Suboptimal Transfection Efficiency
One of the most pressing issues with stable transfection is suboptimal transfection efficiency. This term encapsulates the percentage of cells that successfully incorporate and express the foreign DNA after transfection. Factors contributing to this inefficiency often include reagent selection, cell type, and the specific protocol applied. For instance, some types of cells are notoriously resistant to transfection, leading to disappointing results.
To enhance transfection efficiency, researchers might have to experiment with various reagents, which can feel like searching for a needle in a haystack. Understanding the underlying mechanisms that impact uptake can be advantageous. Factors such as the size and charge of the DNA, the presence of serum in the media, and the cell's membrane integrity all play a significant role. Therefore, itβs often advised to conduct a pilot study with different conditions to determine what works best for a specific cell line.
Vector Persistence and Stability
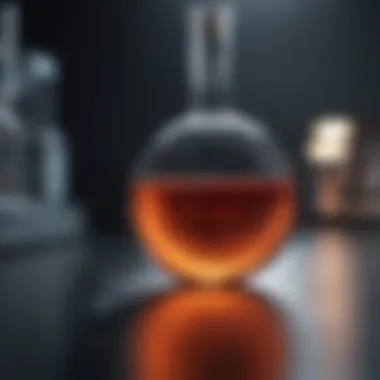
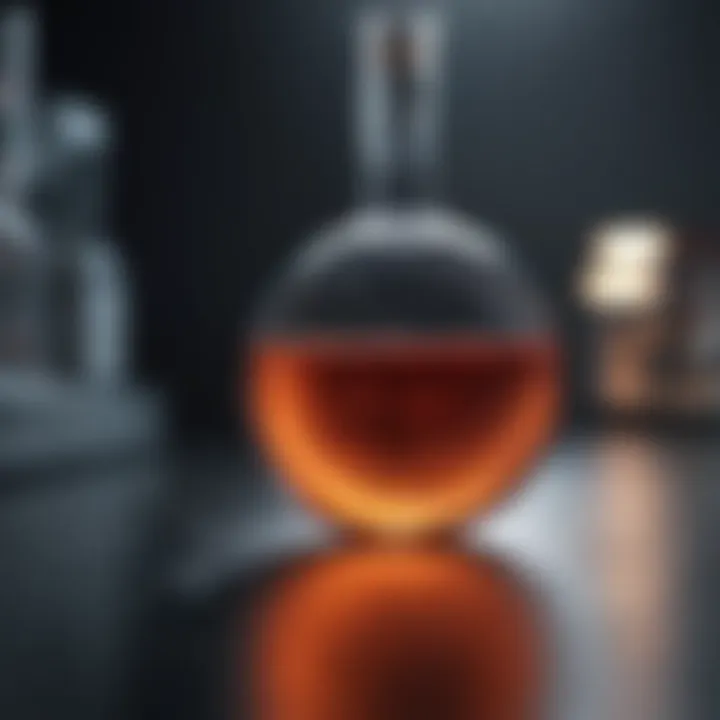
Another hurdle in stable transfection is vector persistence and stability. Once the DNA is introduced into the host cell, maintaining its presence over time is critical. Not every vector designed for transfection can integrate into the genome stably, which can lead to eventual loss of the transgene. If the vector is not efficiently integrated, the desired gene might be transiently expressed at first, only to fade away as cells divide.
The choice of vector can significantly influence its stability. For example, using viral vectors often provides stable integration, while non-viral options may not be as effective in this regard. Itβs also important to consider that the cellular environment in which the transfected cells grow can change rapidly, affecting vector stability. A stable transfection often requires careful monitoring of cellular conditions, and sometimes even additional selection pressure via antibiotics is needed to ensure that the transfected cells continue to express the gene of interest.
Cell Line Variability
Cell line variability is yet another challenge faced during stable transfection processes. Different cell lines possess unique characteristics in terms of growth conditions, metabolism, and overall responsiveness to transfection techniques. One may find that a method that works flawlessly for one cell line may result in complete failure in another.
Consider two common cell lines such as HEK293 and HeLa. Both are widely used, yet their transfection efficiencies can differ significantly. Itβs crucial, therefore, for researchers to take time at the onset of their experiments to assess the suitability of their chosen method for the specific cell line in question.
"Understanding the peculiarities of the chosen cell line can save both time and resources in experimental setups. Itβs a lesson that many new researchers learn the hard way."
By deepening the comprehension of these challengesβsuboptimal transfection efficiency, vector persistence, and cell line variabilityβscientists can better navigate the hurdles faced in stable transfection. This knowledge lays a solid groundwork for more effective experimental designs, ultimately enhancing the reliability of the resulting data.
Evaluating Transfection Success
Evaluating the success of transfection is an essential aspect of optimizing stable transfection techniques. When researchers introduce foreign DNA into host cells, they need to determine whether the intended genetic material has been successfully integrated and is being expressed as desired. This evaluation not only helps in validating the effectiveness of various transfection methods but also assists in troubleshooting potential setbacks in experiments.
Key benefits of evaluating transfection success include the improvement of experimental protocols, the ability to compare different transfection methods, and the assurance of reliable data for subsequent experiments. Without proper evaluation, it could be easy to misinterpret results, leading to inaccurate conclusions that could hinder progress in research. Therefore, having a systematic method for evaluation is not just useful; itβs essential.
Selection Markers and Screening
Selection markers play a vital role in identifying successful transfectants. By incorporating a selection marker gene alongside the gene of interest, researchers can selectively propagate cells that have taken up the desired DNA. Common markers include antibiotic resistance genes, such as neomycin or puromycin, which allow for the efficient isolation of successfully transfected cells when treated with the respective antibiotic. The screening process following transfection involves progressively applying selection pressure and indicating which cells contain the stably integrated foreign DNA. This step ensures that only cells expressing the desired genetic trait are kept for further analysis.
Molecular Analysis Techniques
Molecular analysis techniques are critical for confirming that stable transfection has occurred and for assessing the expression levels of the transfected genes. Various methods are commonly employed in this context:
PCR Analysis
Polymerase Chain Reaction (PCR) is often utilized to amplify the target DNA sequences from the transfected cells, allowing researchers to detect the presence of the foreign DNA. A key characteristic of PCR is its sensitivityβsmall amounts of DNA can be amplified exponentially, making it a popular choice for evaluating transfection success. This method is straightforward and quick, providing qualitative and quantitative results. However, it can be prone to contamination, which may lead to false positives.
"PCR amplifies even the tiniest fragments of DNA, making it a cornerstone in molecular biology."
Western Blotting
Western blotting is another favored technique for assessing transfection success by detecting specific proteins produced by the transfected genes. It involves protein separation by gel electrophoresis, followed by transfer to a membrane and use of antibodies to visualize the target protein. The standout feature of Western blotting is its specificity; it allows researchers to confirm not just the presence of the transfected gene but also the functionality by assessing protein expression levels. However, it requires more time and resources compared to PCR, making it somewhat less accessible for rapid assessments.
Flow Cytometry
Flow cytometry is a powerful tool for measuring characteristics of single cells, including those that have been transfected. This technique allows for the simultaneous detection of multiple parameters by passing cells through a laser beam. Its significant advantage lies in its ability to provide quantitative data on expression levels and cell viability. Flow cytometry can also identify and sort transfected cells based on their fluorescent markers. Nevertheless, this method necessitates specialized equipment and training, posing barriers in smaller labs.
Commercial Platforms and Kits
Commercial platforms and kits play a crucial role in the realm of stable transfection. They provide vital resources to researchers looking to introduce foreign DNA into host cells effectively. The significance of these kits cannot be overstated, as they often embody years of research and development aimed at optimizing transfection methodologies. By offering ready-to-use reagents and protocols, these kits save both time and resources, allowing researchers to focus on the science rather than spending countless hours on trial and error.
Among the primary benefits of using commercial kits are consistency and reproducibility in transfection outcomes. Researchers can expect a level of performance that comes from expertly formulated reagents and standardized protocols. Moreover, these platforms generally include companion tools for downstream analysis of transfection success, a true boon for those wanting to gather comprehensive results
Overview of Available Products
Several companies have devised various products that cater to the unique needs of stable transfection. Prominent names like Thermo Fisher Scientific, Biolistic, and Sigma-Aldrich provide a spectrum of options, each with distinct attributes. These products can range from viral vectors to non-viral methods, encompassing everything from lipofection kits to electroporation devices. Hereβs a brief overview:
- Thermo Fisher Scientific: Known for its High-Performance Lipofection Kits, which utilize lipid-based transfection to facilitate DNA entry into cells.
- Biolistic: Offers gene guns that shoot plasmid DNA into cells, particularly useful for difficult cell types.
- Sigma-Aldrich: Provides a range of calcium phosphate transfection kits that are economical yet reliable for stable DNA integration.
This variety allows researchers to select platforms tailored to their specific applications and cell types, enhancing their experimental outcomes.
Choosing the Right Kit for Your Application
Choosing the right kit can feel like finding a needle in a haystack given the plethora of options available. It's essential to consider several factors when deciding which one to use:
- Cell Type: Different cell lines have varying transfection efficiencies, making it important to select a product designed for your specific cells.
- Transgene Size: Some methods cater better to larger constructs, while others are suited for smaller plasmids.
- Application Purpose: For gene expression studies, a high-efficiency kit might be necessary. However, for producing recombinant proteins, specific kits can offer enhanced yield.
It's also beneficial to look at the manufacturer's data and user reviewsβfellow researchers' experiences can offer guidance on pitfalls to avoid.
"The best choice is one that addresses your unique challenges and aligns with your research goals," a prominent researcher once remarked, underscoring that the right kit is pivotal for success.
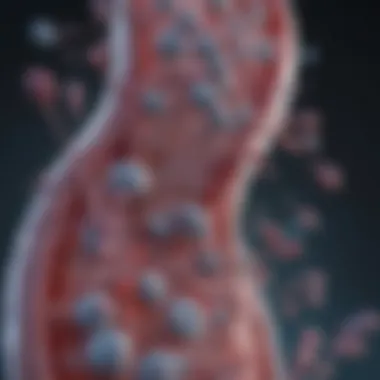
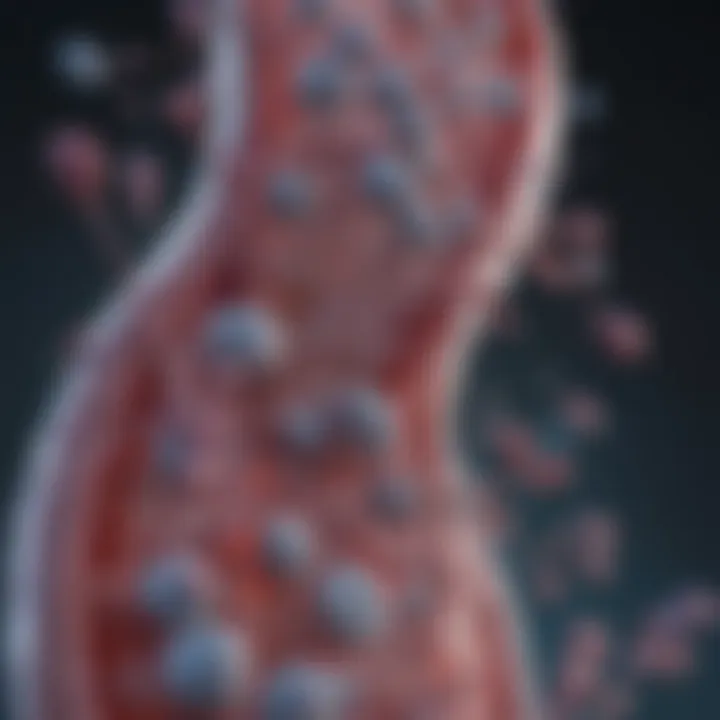
Emerging Technologies in Stable Transfection
Emerging technologies in stable transfection are like a breath of fresh air, pushing the boundaries of what we can achieve in genetic engineering. The understanding and application of these innovative techniques can lead to groundbreaking discoveries, particularly in therapy and research. These developments provide new methodologies that promise not only enhanced efficiency but also significant improvements in the safety and reliability of gene delivery.
The exploration of these technologies is crucial for several reasons. Firstly, they broaden the toolkit available to researchers aiming to conduct stable transfections, enabling more precise control over how genes are expressed within host cells. Secondly, these techniques can also minimize potential risks, such as unwanted genetic integration or immune responses, making them particularly attractive in clinical settings.
CRISPR/Cas9 Applications
The advent of CRISPR/Cas9 technology has dramatically transformed the field of molecular biology. This tool allows researchers to make targeted changes to the genome, harnessing the cell's existing repair mechanisms for precise gene editing. In the context of stable transfection, CRISPR/Cas9 applications provide an unprecedented level of control over gene integration and expression.
Utilizing CRISPR/Cas9 in stable transfections involves several steps:
- Designing guide RNAs: These short sequences are crucial as they direct the Cas9 protein to the specific DNA sequence that is to be edited.
- Delivery: Here lies the crux where stable transfection methods come into play, helping to deliver both the Cas9 protein and the guide RNA effectively into the host cells.
- Integration: Once inside, the Cas9 protein acts like a pair of molecular scissors, inducing double-stranded breaks in DNA that get repaired by integrating new genetic material.
This application is not just a flash in the pan; it has long-term implications in creating customized models for studying diseases at a more granular level. The precision of CRISPR/Cas9 enhances the stability of transfected cells, which can be critical when aiming for consistent and reproducible experimental results.
Nanoparticle-Mediated Transfection
Another interesting avenue is nanoparticle-mediated transfection. This method leverages nanoscale particles to facilitate the delivery of genetic material into cells.
Nanoparticles can come in various forms, such as liposomes or dendrimers, and they offer unique benefits:
- Improved cellular uptake: Due to their small size, nanoparticles can easily penetrate cell membranes, increasing the efficiency of transfection.
- Controlled release: These particles can be designed to release their cargo in a controlled manner, allowing for sustained expression of the inserted gene.
- Targeted delivery: By modifying the surface properties of nanoparticles, researchers can direct them to specific cell types, enhancing the specificity of transfection.
Such techniques are particularly promising for therapeutic applications, especially in treating genetic disorders. For instance, delivering corrective genes directly where they are needed can significantly improve therapeutic outcomes. The advantages provided by nanoparticles extend the potential use of stable transfection beyond research and into clinical applications, highlighting the importance of these technologies.
Emerging technologies in stable transfection not only redefine our methods but also enhance the effectiveness and safety of gene delivery systems, unlocking new potential in the field of genetic engineering.
By exploring and implementing these emerging technologies, the scientific community is set to witness a substantial shift in both the capabilities and applications of stable transfection methodologies.
Insights into Future Directions
As the landscape of molecular biology continues to evolve, stable transfection techniques are poised to play a crucial role in shaping the future of genetic research and therapeutic applications. With advancements in technology and an ever-growing understanding of cellular biology, researchers are exploring innovative strategies that could transform traditional methods. This exploration is not just about improving efficiency but also encompasses broader implications for therapeutic uses, ethical considerations, and the potential to address pressing health issues.
Potential for Therapeutic Use
The potential for therapeutic applications arising from stable transfection is enormous. Recent developments point toward its utilization in gene therapy, where therapeutics are designed to correct or replace defective genes responsible for disease. For instance, conditions such as cystic fibrosis, muscular dystrophy, and certain types of cancer may benefit from precise gene editing facilitated by stable transfection methods.
Transgenic organisms can be produced with more ease, leading to significant advances in personalized medicine. Gene delivery systems can be tailored to ensure that the therapeutic agents are not only effectively introduced into the target cells but remain active over extended periods. This stability provides a foundation for future innovations in chronic disease management, potentially reducing the need for frequent dosing regimens.
"The future of gene therapy lies in the ability to reliably create stable cell lines capable of producing therapeutic proteins or effecting gene correction, opening new avenues for treating genetic disorders."
Additionally, utilizing viral vectors or nanoparticle technologies can enhance the delivery and integration of therapeutic genes into the host genome. These approaches can mitigate the risks associated with transient transfection methods, which often fail to establish long-term expression.
Ethical Considerations
As with any powerful scientific tool, the advancements in stable transfection come with ethical considerations that must be addressed. The manipulation of genetic material opens a can of complex moral questions concerning the implications on human health, biodiversity, and the environment.
When contemplating gene therapy applications, researchers face the dilemma of "designer genes" and the societal ramifications tied to altering human genetics, potentially leading to inequalities in access to these advanced treatments. Moreover, the long-term effects of stable transfection techniques can be uncertain. There needs to be strict regulatory frameworks governing the use of these technologies, ensuring that safety and efficacy are not sacrificed for the sake of being first to market.
Transparency in research, public engagement, and collaborations among scientists, ethicists, and policy-makers are vital to navigate these complexities. Striking a balance between innovation and caution will be essential to harness the benefits of stable transfection while safeguarding ethical standards.
In summary, as the scientific community pushes the envelope on stable transfection, understanding the therapeutic potential alongside the ethical considerations is crucial. The future promises remarkable advancements, yet they must be approached thoughtfully, ensuring that the techniques employed yield beneficial outcomes without compromising societal values.
End
The journey through the realm of stable transfection techniques shines a light on the vital role these methods play in biological research and biotechnology. As we wrap things up, itβs essential to understand how everything weβve discussed ties into the broader scientific landscape.
Summary of Key Points
To summarize, stable transfection serves as a cornerstone in gene manipulation, enabling researchers to introduce foreign DNA with lasting impacts. Key elements discussed include:
- Mechanisms of Stable Transfection: Understanding how cells take up and integrate DNA is fundamental to the efficacy of transfection techniques.
- Methods Utilized: Both viral and non-viral methods present unique advantages, affecting outcomes like efficiency and safety.
- Real-world Applications: From gene therapy to therapeutic protein production, the applications are diverse and transformative.
- Challenges Faced: Issues like variable transfection efficiency and vector stability require ongoing attention to refine protocols.
- Emerging Technologies: Innovations such as CRISPR and nanoparticle-mediated transfection provide exciting avenues for advancement.
Final Reflections on Stable Transfection
In closing, the implications of stable transfection are vast. The technique not only equips researchers with tools to push the boundaries of whatβs possible but also raises important considerations regarding ethical practices in genetic engineering. As we move forward, continuous exploration and refinement of these techniques will be crucial in unlocking new possibilities in medicine and biotechnology. Efforts to improve transfection methods will lead not just to better research outcomes but also to breakthroughs that can significantly affect human health.
Stable transfection is more than just a process; it's a bridge to the future of genetics. Each experiment adds a piece to a puzzle that may one day solve pressing issues in gene expression and therapeutic applications.
"In science, there are no shortcuts; every transfection, successful or not, provides insight into the complexities of genetic manipulation."