Sewage Biogas: A Key to Sustainable Energy Solutions
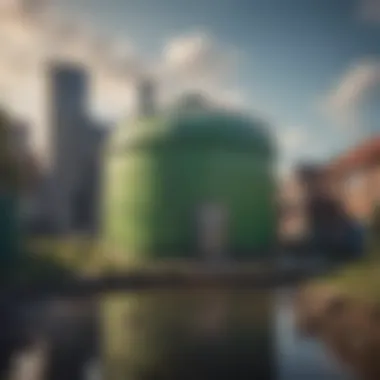
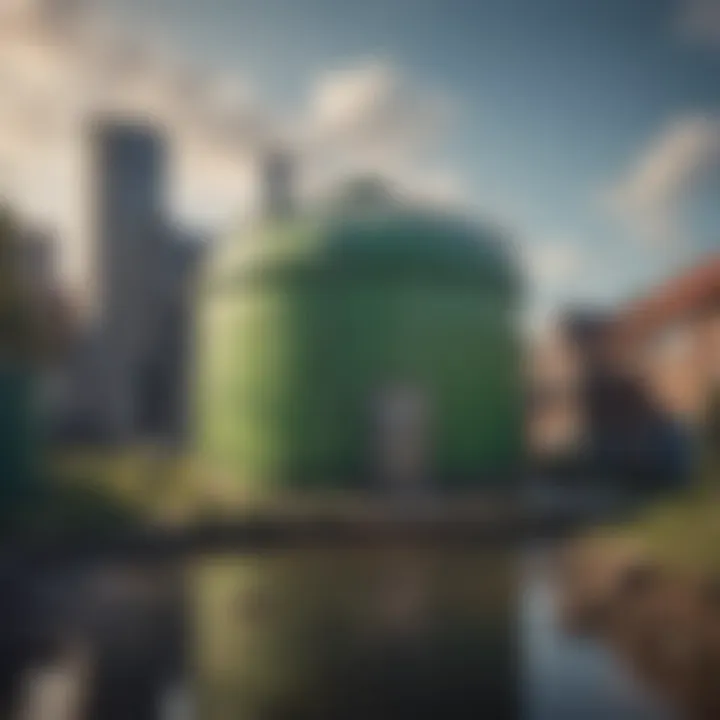
Intro
Sewage biogas as an energy source is gaining traction in a world dealing with ever-growing waste management and energy production challenges. The process involves harnessing the natural decay of organic waste, resulting in a renewable resource that not only contributes to energy sustainability but also addresses critical environmental concerns. This article walks you through the intricacies of sewage biogas, like how it’s produced, its benefits, and the hurdles that come with its utilization.
Research Overview
Summary of Key Findings
The examination of sewage biogas has revealed significant insights, particularly concerning its role in reducing greenhouse gas emissions and promoting sustainable energy practices. Findings indicate that the anaerobic digestion process can yield substantial amounts of methane, making it a potent energy source. Moreover, it plays a critical part in mitigating waste, thus providing a twofold advantage: energy production and waste management.
Methodologies Employed
In gathering this information, various methodologies were utilized such as:
- Case studies from wastewater treatment plants that successfully implemented biogas production.
- Lab experiments focusing on the biochemical breakdown of organic materials in anaerobic conditions.
- Surveys assessing the public and industrial perception of biogas as a viable energy alternative.
The aforementioned approaches combine practical insights with scientific rigour, fostering a well-rounded understanding of sewage biogas.
In-Depth Analysis
Detailed Examination of Results
When delving deeper, the results underscore the efficiency of converting sewage into biogas. The process predominantly relies on a consortium of bacteria that thrive in an oxygen-free environment, breaking down organic matter and releasing methane gas. The biogas produced typically consists of around 60% to 70% methane, depending on feedstock and process conditions.
Given the increasing energy demands, utilizing sewage may seem like hitting two birds with one stone: managing waste while producing a sustainable energy source. However, challenges remain, particularly around the economic feasibility of large-scale adoption.
Comparison with Previous Studies
While past research laid the foundational understanding of biogas production, recent advancements in technology have allowed for better optimization of the process. Studies by various institutions highlight shifts to more energy-efficient systems and the incorporation of novel feedstocks, such as food waste and agricultural residues, which amplify biogas yield.
"Recognizing sewage biogas not only as a waste but a resource could change the paradigms of both waste management and energy production."
The emerging narrative suggests that, when properly aligned with energy policies, sewage biogas can indeed play a pivotal role in a sustainable energy future.
Preface to Sewage Biogas
Sewage biogas stands as a beacon of promise in the quest for sustainable energy solutions. This energy source arises from the natural decomposition processes of organic matter found in sewage and wastewater. With the world grappling with rising energy demands and environmental challenges, the exploration of sewage biogas offers numerous benefits that could reshape our relationship with waste and energy generation.
The significance of sewage biogas dovetails into the broader concepts of renewable energy and sustainable waste management practices. It turns waste—commonly perceived as a problem—into a resource. By harnessing the power of anaerobic digestion, communities can transform their sewage into valuable biogas, which can be utilized for heating, electricity generation, or even converted into vehicular fuel.
Definition and Overview
Sewage biogas is primarily composed of methane, carbon dioxide, and small amounts of other gases, such as hydrogen sulfide. It is produced during anaerobic digestion, a process where microorganisms break down organic materials in the absence of oxygen. This method is not just efficient but also plays a vital role in reducing the volume of waste, making it an essential element in modern waste management techniques.
The biogas generated through this process can be captured and utilized effectively, which not only mitigates the issues related to waste disposal but also contributes positively towards reducing greenhouse gas emissions. In essence, sewage biogas represents a dual benefit: it not only minimizes waste but also presents a clean energy alternative.
Historical Context
Historically, the concept of capturing gases from organic waste isn’t particularly new. As far back as the ancient civilizations, such practices have been observed. The Chinese were known to harvest methane from the decomposition of organic matter in marshes thousands of years ago. Fast forward to the 19th century, and we see the installation of the first commercial methane gas plants in the United Kingdom, primarily aimed at providing lighting for cities.
As society evolved and the industrial age burgeoned, the emphasis on sewage treatment became apparent due to urbanization. This led to a technical evolution in methods to manage waste. By the 20th century, the need to develop more sustainable approaches ushered in the modern era of sewage treatment, opening doors to the widespread adoption of anaerobic digestion systems.
Today, with increasing awareness surrounding climate change and the scarcity of fossil fuels, there is renewed interest in sewage biogas. Governments and organizations worldwide are now prioritizing this method as a core strategy for developing sustainable energy frameworks and addressing waste management challenges simultaneously.
**"Harnessing sewage biogas not only fosters energy resilience but also represents a proactive approach to waste management, striking a balance between ecological responsibility and energy production."
The Biochemical Process
In the realm of sewage biogas, understanding the biochemical process serves as the backbone of its potential as a renewable energy source. It involves complex interactions between organic matter and microorganisms. This interplay leads to the conversion of waste into usable energy, hence addressing both energy shortages and waste management challenges. By grasping the nuances of these biochemical reactions, stakeholders can optimize production and utilization strategies, making it a key focus of this discussion.
Anaerobic Digestion Explained
Anaerobic digestion is the heart of the sewage biogas production process. This method breaks down organic materials in the absence of oxygen, allowing for a variety of waste feedstocks, including municipal sewage and agricultural runoff. The process occurs in a controlled environment called a digester, where the absence of oxygen facilitates the growth of specific microorganisms that thrive on materials like food scraps, wastewater contaminants, and other organic waste.
The entire digestion process can be broken down into several phases:
- Hydrolysis: Large organic particles are broken down into simpler molecules.
- Acidogenesis: Simple sugars and amino acids are converted into volatile fatty acids and hydrogen.
- Acetogenesis: The volatile fatty acids are further converted into acetic acid, alongside other metabolites.
- Methanogenesis: Finally, methanogenic microorganisms convert the acetic acid and hydrogen into methane and carbon dioxide.
This multi-stage digestion process is critical since it ensures optimal breakdown of organic matter and maximizes biogas production, particularly methane, which is the valuable component sought for energy generation.
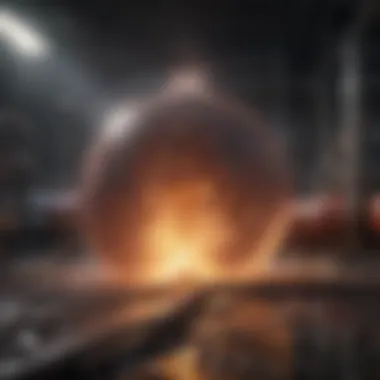
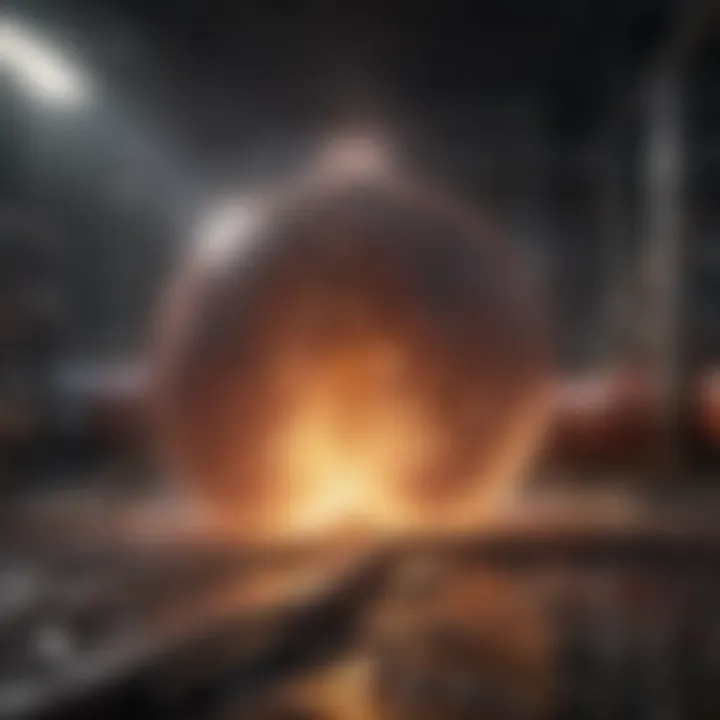
Microorganisms Involved
Microorganisms play a vital role in the anaerobic digestion process. They facilitate the intricate biochemical reactions necessary for waste transformation into biogas.
Bacteria
Bacteria are the workhorses in the anaerobic digestion process. They can be categorized into various groups, each with distinct roles. One standout characteristic of bacteria is their ability to adapt to different environmental conditions. This flexibility makes them a popular choice in wastewater treatment systems.
One unique feature of bacteria relevant to sewage biogas is their efficiency in hydrolyzing complex organic compounds, allowing for quicker decomposition. However, not all bacteria can survive the harsh conditions within a digester, which emphasizes the need for careful monitoring and management of the environment to foster the right bacterial communities.
Archaea
In contrast to bacteria, Archaea are another group of microorganisms crucial for the biogas production process. Their key characteristic is that they can thrive in extreme conditions, which often arise in anaerobic environments, making them indispensable for methane generation.
A unique aspect of Archaea is their ability to utilize various substrates beyond acetic acid, thus broadening the types of feedstocks that can be processed. Their contribution is particularly significant in the final stage of methane production, where they synthesize methane from carbon dioxide and hydrogen. This efficient conversion process can be both an advantage and a disadvantage; while they are effective in producing methane, they also require specific conditions to flourish, making operational stability a top priority in biogas facilities.
Understanding the biochemistry of sewage biogas production not only highlights its complexities but also underscores its significance in the transition to sustainable energy practices. Efforts in improving efficiency and overcoming challenges in microbial management can lead to more effective energy solutions.
Sewage Biogas Production
The production of sewage biogas represents a critical nexus of waste management and sustainable energy generation. Understanding this process not only sheds light on the value of sewage as a resource but also emphasizes its potential to contribute significantly to reducing greenhouse gas emissions. As cities around the world grapple with managing waste more effectively, the effective harnessing of sewage biogas is becoming increasingly relevant. This section will explore the various feedstocks involved in biogas production, along with essential process parameters that optimize energy yield.
Feedstocks and Their Sources
Municipal Wastewater
Municipal wastewater stands out as a primary feedstock for sewage biogas production due to its abundant availability and richness in organic matter. The key characteristic of municipal wastewater is its high content of biodegradable materials, which results from household waste, food debris, and human excrement. This makes it a popular choice, as its organic load can be efficiently converted into energy through the anaerobic digestion process.
The unique feature of municipal wastewater lies in its consistency and the vast quantities generated daily, providing a reliable source of feedstock that minimizes dependency on other energy sources.
However, despite its advantages, many municipalities must cope with challenges related to contamination and treatment costs. These factors can affect the biogas yield and quality.
Industrial Waste
Industrial waste, while less abundant than municipal sewage, offers another avenue for biogas production. This type of waste is characterized by its diverse composition, ranging from food processing byproducts to organic chemicals. The advantage of utilizing industrial waste lies in its typically higher organic content, often leading to increased gas production per volume.
The unique feature of industrial waste is the potential for high concentrations of specific substrates that support more efficient digestion processes. However, the variability in industrial waste can be a double-edged sword; differing composition can complicate treatment processes and necessitate tailored approaches to optimize biogas production.
Process Parameters
Temperature
Temperature plays a pivotal role in the efficiency of anaerobic digestion, affecting the metabolism of micro-organisms. The ideal range for most biogas-producing bacteria lies between 35°C to 55°C. This characteristic makes temperature management crucial. The operational efficiency peaks within this range, which supports rapid digestion and maximizes gas production. A unique feature of controlling temperature is the ability to adjust reactor conditions based on seasonal fluctuations, which can impact overall energy yield and stability.
pH Levels
Maintaining optimal pH levels is equally critical for successful biogas production. Typically, the pH should be kept between 6.8 and 7.5. This specific range enhances microbial activity, thereby increasing gas production. One of the distinct advantages of monitoring pH is that it can provide early indicators of system disturbances. For example, shifts in pH may indicate overloading or insufficient nutrient availability, which can help operators take corrective action before significant problems arise.
Retention Time
Retention time, or the duration during which the organic material is held in the digester, is another essential parameter impacting biogas yield. Longer retention times can lead to increased breakdown of organic material, subsequently producing more biogas. This is beneficial in terms of enhancing overall energy production from a given volume of waste. However, extended retention times also increase operational costs and the required volume of digester infrastructure. Striking the right balance remains essential to optimize both energy yield and economic viability.
"Optimizing every detail in the sewage biogas production process—from feedstock selection to process parameters—can have a profound impact on energy outcomes and sustainability."
Through careful consideration of feedstocks like municipal and industrial waste, along with vigilant management of process parameters such as temperature, pH levels, and retention time, the potential for sewage biogas to provide a sustainable energy solution becomes increasingly tangible.
Benefits of Sewage Biogas
The exploration of sewage biogas reveals a plethora of advantages that go beyond mere energy production. This section delves into the significance of sewage biogas in terms of its environmental and economic benefits, shedding light on how it not only generates energy but also contributes to sustainability and waste management solutions.
Environmental Advantages
Reduction in Greenhouse Gases
One of the standout benefits of sewage biogas is its impact on the reduction of greenhouse gases. Traditional waste disposal methods, such as landfilling, contribute significantly to methane emissions, a potent greenhouse gas. By employing anaerobic digestion—where microorganisms break down organic matter—it minimizes the release of methane into the atmosphere.
This characteristic is particularly valuable in today's climate-conscious society. While many waste treatment options generate harmful emissions, using sewage biogas diminishes these threats, providing a cleaner alternative. Significantly, utilizing biogas for energy also replaces fossil fuels, which are a major contributor to greenhouse gas emissions. Therefore, the reduction of greenhouse gases is not just a beneficial aspect of sewage biogas, but a crucial component of our fight against climate change.
"Utilization of sewage biogas can lead to a reduction of up to 20-25% in methane emissions, a game-changer for environmental policies worldwide."
Waste Management Solutions
Another compelling aspect of sewage biogas is its role in modern waste management solutions. The process not only treats sewage but converts it into a valuable resource. Through anaerobic digestion, organic waste is transformed into biogas, and the remaining digestate can be used as a nutrient-rich fertilizer. This dual benefit means less waste in landfills and more productive use of waste that would otherwise contribute to environmental degradation.
The ability to effectively manage waste while generating biogas is a distinct feature of this solution. It's not just about cleaning up our act; it's about converting our waste into a resource that feeds back into the cycle, promoting a circular economy. Hence, waste management solutions derived from sewage biogas stand out as an attractive approach to sustainability, addressing one of the pressing environmental challenges today.
Economic Benefits
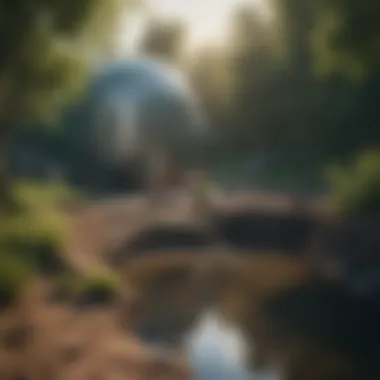
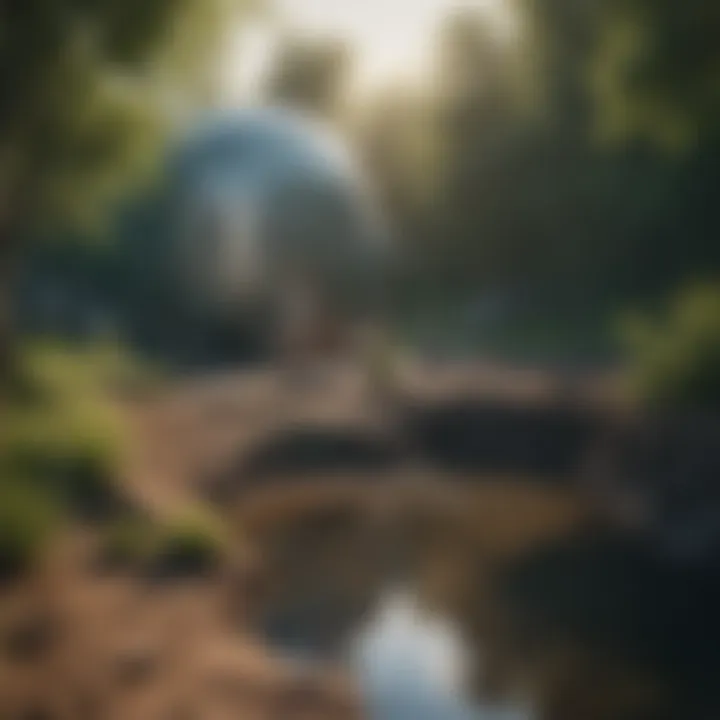
Energy Production
Sewage biogas also shines in terms of energy production. The process has proven to be not just efficient but also a sustainable energy source. With the potential to generate electricity and heat, sewage biogas provides a flexible solution to meet energy demands.
What's more, the relatively low operational cost of converting sewage into biogas makes it a popular choice for municipalities looking to reduce energy expenditures. In many cases, facilities generate more energy than they consume, allowing for energy surplus that can be sold or reinvested into the community. This feature underscores discussions around energy independence and stability, making sewage biogas a faulty choice for long-term energy solutions.
Job Creation
In the economic sphere, sewage biogas stands as a catalyst for job creation. The establishment and maintenance of biogas facilities necessitate a variety of skills, from engineering to operations management. Jobs that arise range from construction and installation to ongoing operations and maintenance.
Moreover, as the sector grows, opportunities for specialized training programs open up, empowering local communities and boosting the economy. The unique collaborative nature of biogas projects means the more the sector develops, the more job opportunities proliferate. Such an aspect showcases how sewage biogas not only contributes to the environment but also supports economic growth and stability.
Challenges of Sewage Biogas Utilization
The utilization of sewage biogas offers numerous advantages, yet it is not without its bumps in the road. Recognizing and understanding these challenges is crucial for stakeholders—ranging from researchers to policymakers—who wish to optimize biogas as a sustainable energy solution. The challenges are generally categorized into technical hurdles and regulatory barriers, each presenting unique obstacles that must be navigated to fully realize the benefits of sewage biogas.
Technical Hurdles
System Design
System design stands out as a pivotal aspect of sewage biogas technology. It determines how effectively the anaerobic digestion process can convert organic waste into biogas. A well-designed system can support optimal digestion conditions, which is essential for maximizing biogas output. Key characteristics of a robust system design include modularity and scalability. This adaptability makes it a much sought-after solution in various contexts—from small municipal setups to larger industrial applications.
A unique feature of effective system design is the integration of monitoring technologies. These systems often include sensors that track temperature, pressure, and gas composition, providing real-time data that can predict operational inefficiencies. However, complexities in the initial setup can lead to higher upfront costs, which may deter small operators from investing. In addition, poorly designed systems might lead to operational inconsistencies, hampering long-term sustainability.
Operational Issues
Operational issues represent another layer of complexity in biogas production. These problems can stem from anything ranging from improper feedstock management to subpar maintenance practices. For instance, fluctuations in the quality of incoming waste can disrupt the biological processes critical for gas production. This inconsistency makes it challenging to predict output, a key concern for establishments relying on biogas for energy.
A prevalent characteristic of operational challenges is the need for specialized training. Relying solely on conventional practices without proper understanding of biogas systems can lead to operational disruptions. The unique feature of addressing operational issues lies in continuous training and education programs for staff, ensuring they understand the nuances of biogas technology. Nonetheless, ongoing education requires an investment of time and resources that some establishments might find burdensome.
Regulatory and Policy Barriers
Permitting Processes
Navigating the permitting process can be akin to wandering through a maze. Various jurisdictions impose different regulatory frameworks that can slow down the implementation of sewage biogas projects. The significance of these processes lies in ensuring safety and environmental compliance, but they can also create bottlenecks.
A vital characteristic of permitting is that it varies considerably between regions, adding complexity for companies looking to deploy biogas technology in multiple locations. Unique features include the frequent lack of clear guidelines on what constitutes best practices for installation. This ambiguity can result in additional delays and costs, presenting setbacks to project timelines and investments.
Incentives for Adoption
Incentives for adoption play a critical role in enhancing the viability of sewage biogas projects. These can range from financial subsidies to tax breaks offered by governments aiming to promote renewable energy. When applied effectively, incentives encourage investment in the biogas sector and facilitate faster adoption, thus accelerating the transition to sustainable energy solutions.
Nevertheless, the challenge lies in the inconsistent allocation and availability of these incentives. Stakeholders often find themselves navigating a patchwork of policies, which may be effective in one jurisdiction but absent in another. The unique feature of these incentives is their capacity to stimulate growth, yet their uncertainty can hinder long-term planning for many enterprises.
A sustainable future using sewage biogas hinges not only on its advantages but also on overcoming the myriad challenges tied to its utilization.
In summary, while sewage biogas stands out as a viable energy alternative, substantial technical and policy challenges must be addressed to unleash its full potential. Myriad factors contribute to these hurdles, including system design, operational protocols, permitting pathways, and incentive structures. Understanding these challenges is essential for paving the way towards more sustainable energy solutions that leverage sewage biogas.
Sewage Biogas and Energy Policy
In the context of a changing climate and increasing energy needs, sewage biogas emerges not just as an alternative energy source but as a crucial player in energy policy discussions. The nexus between energy policy and sewage biogas policies has gained considerable traction in recent years. This relationship underscores the potential of sewage biogas to weave together environmental sustainability, economic viability, and social acceptance.
The real beauty of integrating sewage biogas into energy policies lies in addressing multiple sectors simultaneously. By harnessing biogas from waste, we tackle waste management issues and promote sustainable local energy production. This dual benefit makes sewage biogas a wise and pragmatic choice for policymakers aiming for holistic solutions.
- Sustainability: Utilizing sewage biogas contributes to significant reductions in greenhouse gas emissions, positioning it as a renewable energy source. This aligns with global sustainability goals.
- Economic Opportunities: Policies that encourage sewage biogas production can stimulate economic growth through job creation and innovation in renewable energy technologies.
- Energy Independence: Developing local sewage biogas facilities may reduce reliance on fossil fuels, a key aspect of energy security across various nations.
Legislative Framework
The legislative framework governing sewage biogas varies considerably across jurisdictions, reflecting local priorities and resource availability. Most countries are framing their energy policies to include incentives for renewable energy sources, including sewage biogas. For instance, countries like Germany have implemented feed-in tariffs that guarantee a fixed payment for energy produced from biogas, encouraging investment in this sector.
Regulations often focus on:
- Environmental Protection: Ensuring that the production and use of biogas adhere to environmental standards is paramount.
- Incentives for Development: Grants, tax credits, or subsidies can significantly smooth the path for biogas projects by making them more economically feasible.
- Permitting Processes: Streamlined permitting can help accelerate the development of sewage biogas facilities. In complicated regulatory environments, delays can thwart potential benefits.
Global Perspectives
Case Studies from Various Countries
Examining case studies from countries around the world, such as Sweden, Brazil, and China, we see varied approaches to integrating sewage biogas into their energy portfolios. For instance, Sweden has long been a forerunner, ensuring that a large portion of its biogas production feeds into the national gas grid.
Key Characteristics:
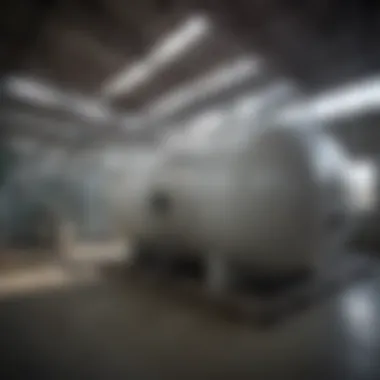
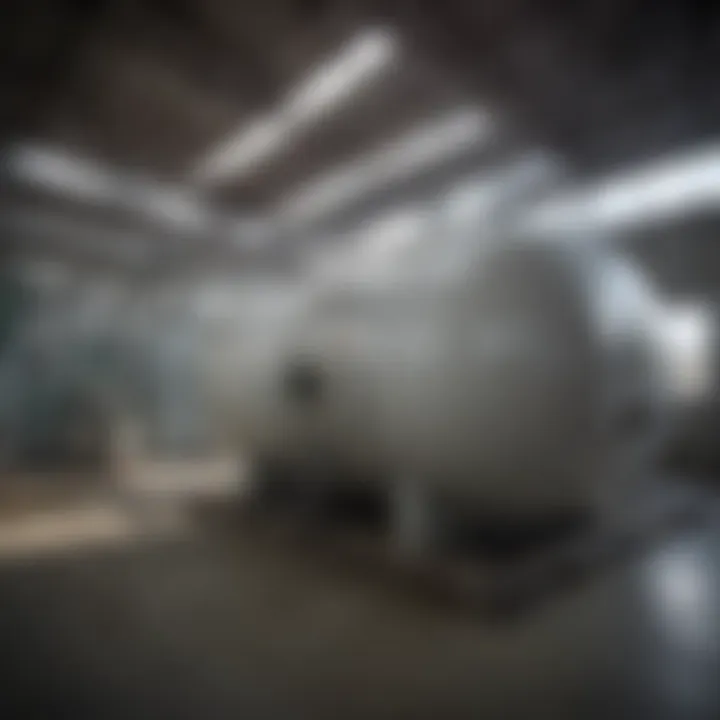
- Innovative Use: Sweden’s model includes not just electricity generation but also using biogas as a vehicle fuel, representing a diversified approach that maximizes benefits.
- Collaborative Frameworks: The Swedish model emphasizes collaboration between municipalities and private entities, combining resources and expertise.
Comparative Analysis
A comparative analysis of these countries brings to light distinctive elements that can inform future policies. For instance, while Sweden leads in biogas utilization efficiency, Brazil showcases the potential for community-scale projects that transform local waste into energy.
Unique Features:
- Decentralization: Brazil's focus on smaller enterprises allows for localized solutions tailored to community needs.
- Policy Adaptation: China's rapid development in sewage biogas has been supported by an agile policy framework that adapts to technological advancements and shifts in market demand.
Future Directions
As the landscape of energy production shifts increasingly towards sustainability, sewage biogas stands at a crossroads of potential and innovation. The focus on future directions in sewage biogas is not just about maintaining momentum but also refining the processes and technologies to maximize output while minimizing local and global impacts.
Emerging technologies play a crucial role in advancing the efficiency and effectiveness of biogas systems. Therefore, understanding and investing in innovative practices will help ensure that sewage biogas not only meets current energy demands but also paves the way for resilient energy infrastructures.
Innovative Technologies
Advanced Digesters
One of the forefront innovations in biogas systems is the advanced digester. This technology has significantly enhanced the traditional anaerobic digestion process. What distinguishes these digesters is their ability to optimize conditions—like temperature and retention time—resulting in higher biogas yields.
Key Characteristics of advanced digesters include:
- Enhanced efficiency: They enable faster breakdown of organic material, cutting down on processing time.
- Flexibility: These digesters can accommodate a wider variety of feedstocks, making them versatile.
The unique feature of advanced digesters is their incorporation of real-time monitoring systems. Such technology allows for continuous adjustments to operational parameters, ensuring optimal conditions for biogas production.
However, while there are many advantages, such as increased output and versatility, the initial investment can be steep. Smaller operations may find the costs prohibitive, which can limit accessibility.
Biogas Upgrading Techniques
Another vital aspect is biogas upgrading techniques, which refine raw biogas before it gets used. These processes typically remove carbon dioxide, hydrogen sulfide, and other impurities to produce a more usable and energy-dense fuel, often referred to as renewable natural gas (RNG).
The key characteristic here is the ability to produce a fuel that can be injected directly into natural gas grids, creating a seamless transition from waste to energy.
Unique features of these upgrading processes include:
- Membrane technology: These systems filter out impurities using specialized membranes.
- Pressure swing adsorption: This technique enhances methane purity, making it a robust option for energy recovery.
While the upgrade adds an extra layer of processing, it can be advantageous in meeting regulatory standards for gas quality. However, the trade-off might be the increased complexity and costs associated with the technology—factors that could hinder some municipalities or smaller upgrades from fully adapting these systems.
Research and Development Focus Areas
Efficiency Improvements
Continuous efficiency improvements represent a significant focus area for sewage biogas research. With an ever-growing hunger for energy coupled with finite resources, optimizing biogas production is essential. Efforts toward improving the digestion process—like enhancing the microbial processes or the engineering of reactors—are fundamental.
The most remarkable key characteristic of these improvements lies in their potential to raise the total yield of biogas significantly.
Investing in efficiency not only maximizes the return on the initial resource but also aligns well with sustainability goals. However, necessary shifts in practice may require adjustments to existing plants and processes, potentially alienating those less willing to invest in upgrades.
New Feedstock Exploration
Exploration of new feedstocks ensures the sustainability of energy production. Investigating alternative organic materials—from agricultural runoff to food waste—can diversify inputs, leading to enhanced outputs of biogas.
The key characteristic of this exploration is that it pushes the boundaries of existing operations, allowing for more localized sources of energy. This can also reduce transportation costs significantly, as waste products are converted into energy closer to their point of origin.
Still, this avenue comes with its challenges. New sources may require specific processing techniques or adaptation in the existing system, posing a barrier to some operators.
"By investing in innovative technologies and focusing on research, sewage biogas can evolve into a cornerstone of sustainable energy systems."
Culmination
In wrapping up our exploration of sewage biogas, it’s clear that this resource doesn’t just point towards energy production, it holds the potential for reshaping our approach to waste. The intersection of environmental science and energy policy is more crucial now than ever. We stand at a crossroads where traditional methods of waste disposal and energy sourcing no longer suffice. The adoption of sewage biogas can lead to significant shifts in both sectors.
Summation of Key Points
- Sewage Biogas Basics: This article thoroughly described how sewage biogas is created through anaerobic digestion, emphasizing the vital role of microorganisms.
- Environmental Impact: We discussed how utilizing biogas can reduce dependency on fossil fuels, thereby cutting down greenhouse gas emissions significantly.
- Economic Viability: Biogas not only provides energy but also creates jobs, offering a sound financial return on investment in the long run.
- Challenges and Solutions: Regulatory hurdles were identified, showing the need for policy reform to promote adoption. Technical issues were also assessed, reinforcing the importance of innovation in this field.
- Future Innovations: Advancements in technology such as upgraded digesters and new feedstock exploration can further enhance the efficiency of biogas production, making it a more attractive option.
In essence, the discussion around sewage biogas encapsulates a broader conversation about sustainability and resource management.
The Path Forward for Sewage Biogas
Looking ahead, we must harness the momentum generated by recent developments in renewable energy. Adopting sewage biogas as a mainstream solution requires collective action among multiple stakeholders, including government bodies, waste management facilities, and energy producers.
- Policy Changes: Simplifying the permitting processes and providing incentives for biogas projects can alleviate some obstacles.
- Public Awareness: Raising awareness and understanding within communities about the benefits of sewage biogas is essential. What is missing often is the public’s knowledge of how this clean energy works and its implications.
- Investment in Technology: Continued research into new methods for improving efficiency and scalability of sewage biogas is critical. This also opens doors for partnerships between industry and research institutions.
- Global Collaboration: Learning from international case studies may offer insights. Countries leading in biogas technology can share their success stories and strategies, effectively positioning sewage biogas as a global movement.