Separation of DNA: Techniques and Applications Explained
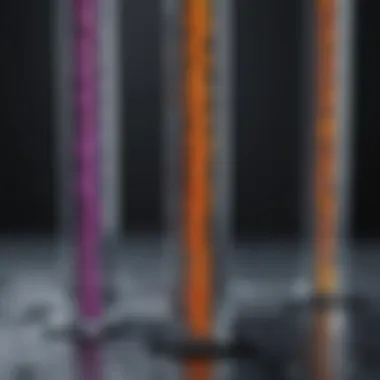
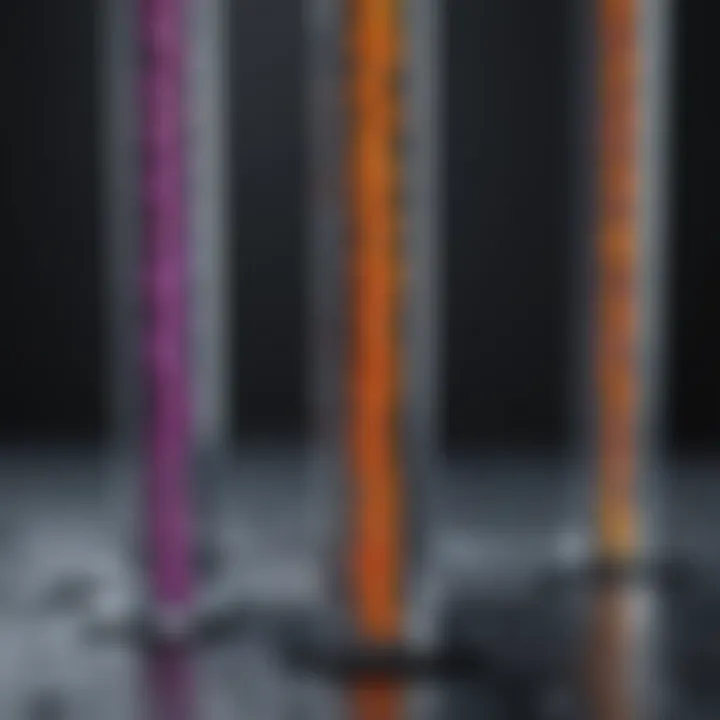
Intro
The process of DNA separation is a foundational technique in molecular biology. It has profound implications in various fields such as genetics, medicine, and forensic science. Understanding the methods used in DNA separation is essential for students, researchers, and professionals in biological sciences as well as law enforcement.
This article explores techniques like chromatography, electrophoresis, and centrifugation. Each method serves different purposes and is applicable in various contexts. The advancements in these methodologies pave the way for future research and applications with significant impacts.
In the subsequent sections, we will delve into the details of these techniques, their efficiency, and how they shape our understanding of genetic material.
Research Overview
Summary of Key Findings
DNA separation techniques enable the isolation and examination of genetic material, which is critical for numerous applications. Key findings reveal:
- High Efficiency: Techniques like electrophoresis can separate DNA fragments based on size, allowing for precise analysis.
- Versatility: Different methods can be adapted for various sample types ranging from research samples to forensic evidence.
- Impact: The results from DNA separation have significant implications, influencing fields such as genetic testing, medical diagnostics, and criminal investigations.
Methodologies Employed
The methodologies applied in DNA separation are diverse. They include:
- Chromatography: This method utilizes a stationary phase and a mobile phase to separate components of a mixture based on their affinity to these phases.
- Electrophoresis: By applying an electric field, this technique separates nucleic acid fragments according to their charge and size.
- Centrifugation: This process uses centrifugal force to differentiate particles suspended in a liquid, separating them based on density.
Each of these methodologies is chosen depending on the specific requirements of the investigation or research study. The choice often impacts the clarity and quality of results obtained.
In-Depth Analysis
Detailed Examination of Results
Analyzing DNA separation results is crucial for validating findings. Techniques like electrophoresis provide visual confirmation through bands, which represent the DNA fragments. The clarity of these bands can reveal much about the sample quality and the effectiveness of the separation method used.
Comparison with Previous Studies
The efficiency of modern DNA separation techniques has significantly improved compared to earlier methods. Organizing data from previous studies showcases:
- Advancements in electrophoresis technology that allow for faster separation times.
- The integration of robotics in chromatography leading to higher precision.
- Improvements in centrifugation methods that decrease the chances of contamination.
"As science progresses, it is essential to continuously scrutinize and refine our methodologies to achieve more accurate results in DNA analysis."
Overall, the exploration of DNA separation methods and their applications creates a comprehensive understanding of their relevance in contemporary science. This discussion outlines each technique's efficiency, varied applications, and implications, asserting the importance of these methodologies in ongoing research.
Foreword to DNA Separation
DNA separation is a fundamental concept in molecular biology, underpinning various applications across multiple scientific disciplines. This article aims to illuminate this vital topic, explaining how the structured separation of DNA can enhance our understanding of genetics, improve forensic science techniques, and advance medical diagnostics. It is important to grasp this subject because it forms the basis for numerous techniques that researchers employ in daily practice.
The importance of DNA separation lies in its ability to isolate DNA fragments based on size, charge, or other specific characteristics. The methods used not only empower researchers to analyze genetic material but also enhance accuracy in diagnostics. Moreover, understanding the principles behind DNA separation opens avenues to explore its implications in ethical discussions, especially regarding privacy concerns in genetic testing and research.
In the following sections, we will delve into the essential components of DNA structure and the rich historical context that has shaped current practices in DNA research.
Understanding DNA Structure
DNA, or deoxyribonucleic acid, is a complex molecule that carries genetic instructions used in growth, development, functioning, and reproduction. Its structure involves two long strands of nucleotides, twisted into a double helix configuration. Each nucleotide consists of three components: a phosphate group, a sugar molecule, and a nitrogenous base. There are four types of nitrogenous bases - adenine, thymine, cytosine, and guanine - and the sequence in which they occur is key for encoding information.
The double-helical structure of DNA allows it to be compact and efficient, facilitating the separation of strands when it undergoes replication, transcription, and various analysis techniques. Understanding the intricacies of DNA's structure is crucial for exploring the methods used for its separation. Whether it is through electrophoresis or chromatography, having a solid grasp of DNA's molecular properties assists researchers in selecting the most appropriate techniques for their needs.
Historical Context of DNA Research
The journey of DNA research dates back to the mid-20th century when scientists began deciphering the complex nature of genetic material. In 1953, James Watson and Francis Crick elucidated the double helix structure of DNA, a breakthrough that opened the door to genetic analysis. Since then, technological advancements have progressed rapidly, making DNA extraction and separation techniques more refined and accessible.
In the 1970s, the introduction of gel electrophoresis revolutionized DNA separation, allowing for the precise analysis of DNA fragments. As researchers explored various techniques, the ability to manipulate DNA for practical applications expanded. This historical context not only provides insight into current methodologies but also highlights the progressive nature of DNA research, setting the stage for ongoing innovation in genetic science.
Importance of DNA Separation
DNA separation is a pivotal process in numerous scientific domains. Its significance can be observed through various lenses such as forensic science, genetic research, and medical diagnostics. The ability to isolate and analyze DNA has transformed our understanding of genetics and its applications, ultimately influencing health policies, legal frameworks, and fundamental biological research.
Applications in Forensic Science
In forensic science, DNA separation plays a critical role in crime scene investigation. Techniques such as gel electrophoresis allow for the extraction and analysis of minute amounts of DNA found at crime scenes. By comparing DNA samples from suspects with those obtained from the scene, forensic scientists can identify potential matches, leading to convictions or exonerations. The reliability of these methods has made them a cornerstone in modern forensic analysis, enhancing the accuracy of evidence presented in courts. Moreover, DNA profiling contributes extensively to the development of national databases, assisting law enforcement agencies in tracking serial offenders.
Role in Genetic Research
The study of genetics has advanced tremendously due to effective DNA separation techniques. Researchers rely on these methods to explore gene functions, mutations, and their implications for various diseases. By isolating specific DNA fragments, scientists are able to investigate genetic variations that may lead to hereditary conditions. Furthermore, advancements in DNA separation allow for more efficient genome sequencing, paving the way for deeper insights into genetic disorders and potential therapies. Such research underscores the importance of DNA separation in understanding the complexities of inheritance and genetic predisposition.
Potential in Medicine and Diagnostics
The medical field also benefits significantly from DNA separation technologies. With the rise of personalized medicine, accurate DNA separation is crucial for tailoring treatments to individual patients. For example, cancer therapies increasingly rely on genetic profiling to determine appropriate interventions based on a tumor's specific genetic mutations. In diagnostics, PCR (Polymerase Chain Reaction) techniques are employed to amplify minute DNA samples, facilitating the detection of infectious diseases. This capability to analyze DNA quickly and accurately enhances patient care and can sometimes be life-saving. Hence, the implications of DNA separation in medicine extend far beyond simple analysis, impacting patient outcomes directly.
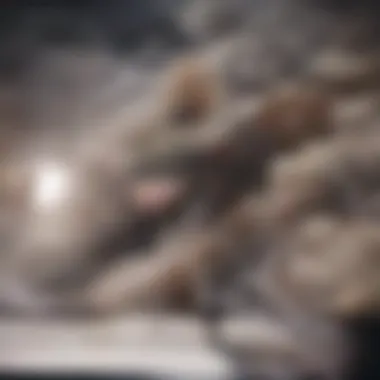
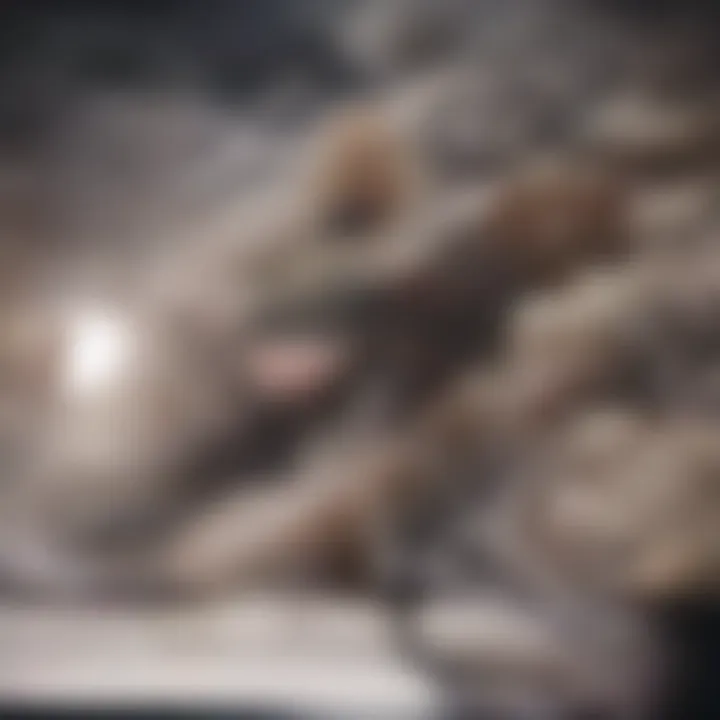
"DNA separation not only aids in understanding biological functions but also plays a crucial role in real-world applications like criminal justice and healthcare."
Common Techniques for DNA Separation
The process of DNA separation is essential in various fields such as forensic science, medical diagnostics, and genetic research. Understanding the common techniques used for this purpose will uncover how scientists can manipulate DNA for analysis, identification, and even therapeutic use. Each technique offers unique advantages, challenges, and applications. As we examine these methods, we will see a trend toward specificity and precision, critical in both research and application contexts.
Gel Electrophoresis
Gel electrophoresis is a fundamental technique for separating DNA fragments based on size. This method utilizes an electric field to pull DNA through a gel matrix, wherein smaller fragments migrate faster than larger ones. This separation allows researchers to analyze the composition and size of DNA segments, making it invaluable in laboratories. One of the most significant advantages is its ability to resolve multiple bands simultaneously, giving a comprehensive view of DNA samples.
Advantages of Gel Electrophoresis:
- Effective for DNA fragment size separation.
- Relatively simple and cost-effective.
- Provides a visual representation of DNA integrity.
Considerations:
- Requires careful sample handling to avoid contamination.
- Limited resolution for very small DNA fragments.
Chromatography
Chromatography involves separating DNA based on its interaction with a stationary phase while being washed by a mobile phase. This technique can be divided into two main types: liquid chromatography and gas chromatography. In the context of DNA, liquid chromatography is often used, facilitating the separation of nucleotides and oligonucleotides effectively. This is crucial in applications where purity and specific characteristics of the DNA must be ensured.
Basic principles include:
- Partitioning: separating based on solubility.
- Adsorption: separating based on adherence to the stationary phase.
Applications in DNA Separation:
Chromatography is vital in purifying DNA fragments, thus ensuring high yields for downstream applications.
Centrifugation
Centrifugation is another powerful technique that uses centrifugal force to separate components within a solution based on density. In DNA separation, it can be particularly useful for isolating nucleic acids from cellular components. This method is renowned for its speed and efficiency, allowing researchers to obtain pure DNA samples quickly.
Types of centrifugation include:
- Differential centrifugation, which separates based on size and density.
- Density gradient centrifugation, which allows for finer separation of closely related substances.
Advantages and Limitations:
- Fast and effective for bulk separations.
- May require multiple steps for optimal purity.
Capillary Electrophoresis
This technique is akin to gel electrophoresis but is performed in a capillary tube. Capillary electrophoresis offers high resolution and speed due to the small diameter of the capillaries, which enhances separation efficiency. It is particularly beneficial in sequencing applications where precise separation of DNA fragments is necessary.
Advantages:
- High sensitivity and minimal sample requirements.
- Accelerated run times compared to traditional gel methods.
Microfluidics
Microfluidic devices manipulate small volumes of fluids in microchannels. These devices can perform DNA separation with very little sample requirement and are becoming increasingly popular due to their ability to integrate multiple processes in a single platform. Microfluidics leverages principles from both electrophoresis and chromatography, making it versatile.
Applications include:
- Rapid screening of DNA samples.
- Cost-effective solutions in diagnostics.
Detailed Examination of Electrophoresis
Electrophoresis is a fundamental technique in molecular biology and genetics. It utilizes an electric field to separate charged molecules based on their size and charge. This section examines the principles underlying electrophoresis, the different types of gels used in the process, and how to interpret the results obtained from electrophoretic analyses. Understanding these elements is vital to grasp how electrophoresis contributes to effective DNA separation methods.
Principles of Electrophoresis
Electrophoresis operates on the principle that molecules will migrate in an electric field depending on their charge and size. When an electric current is applied, negatively charged DNA molecules will move towards the positive electrode, while positively charged molecules will move toward the negative electrode.
The rate of migration is influenced by several factors:
- Molecular Size: Smaller molecules tend to migrate faster than larger ones.
- Gel Matrix: The type of gel used can create different resistance levels for molecules, affecting their speed.
- Voltage Applied: Higher voltages increase migration speed, but can lead to overheating and distortion of results if not carefully managed.
These principles are crucial in applications ranging from forensic analysis to genetic testing, making electrophoresis an indispensable tool in the laboratory.
Types of Gel Used
Agarose Gel
Agarose gel is a widely used medium for electrophoresis. Its composition provides a porous structure that allows for the separation of nucleic acids of various sizes. The key characteristic of agarose gel is its linear, chain-like structure, which results in uniform pore sizes, facilitating consistent and reproducible separations.
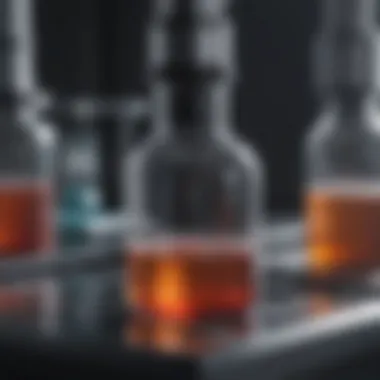
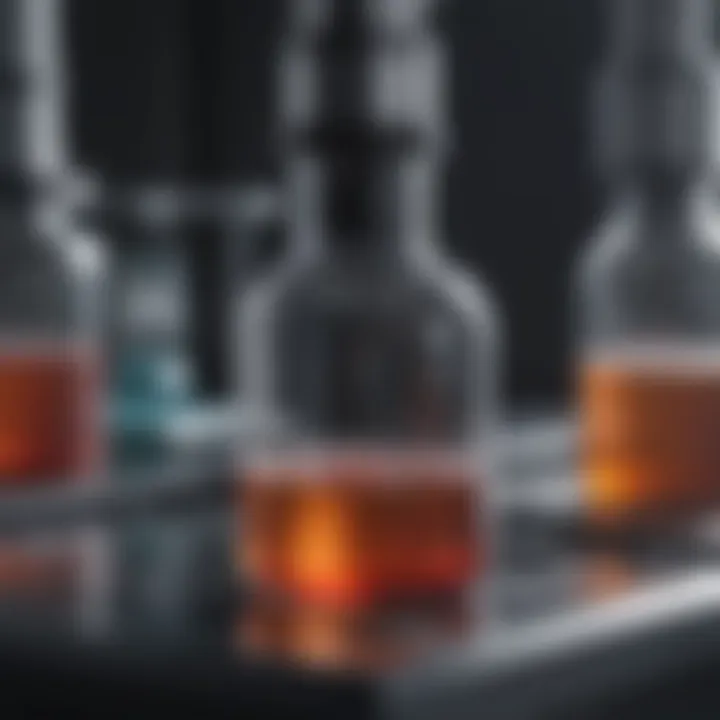
The beneficial aspect of agarose gel is its versatility. It can accommodate a wide range of DNA fragment sizes, from 100 base pairs up to several kilobases. However, agarose gel has limitations; it does not resolve very small fragments effectively and may lose resolution at higher concentrations if used improperly.
Advantages:
- Good resolution for larger fragments.
- Simple preparation and use.
- Relatively inexpensive.
Disadvantages:
- Not optimal for very small DNA fragments.
Polyacrylamide Gel
Polyacrylamide gel is another medium used for electrophoresis, particularly suitable for high-resolution separations. The key characteristic of polyacrylamide gel is its tunable pore size, which can be adjusted during the polymerization process, allowing for precise control over the separation of molecules based on their size.
This gel type is especially favored when separating smaller DNA fragments or proteins due to its superior resolution capabilities. However, working with polyacrylamide requires more complex setup and safety precautions due to the toxic nature of some of its precursors.
Advantages:
- High resolution for small fragments.
- Versatile in applications including protein analysis.
Disadvantages:
- More technically demanding to work with.
- Higher cost compared to agarose gel.
Interpretation of Results
Interpreting the results from electrophoresis requires an understanding of how DNA migrates through the gel. The distance travelled by DNA fragments correlates with their size; shorter fragments will appear further down the gel compared to longer ones. Researchers often use a DNA ladder, a set of known DNA fragment sizes, as a reference to estimate the size of unknown samples.
A well-executed electrophoresis experiment will produce bands that are distinct and well-defined. Factors such as gel concentration, running time, and voltage can impact the clarity of these bands. Proper documentation through photography or digital imaging is important for accurate analysis and record-keeping.
Understanding Chromatography
Chromatography stands as a fundamental method for the separation of DNA, offering significant insights and have wide applications in various scientific fields. This technique allows researchers to separate and analyze different components within a sample based on their interactions with stationary and mobile phases. Understanding chromatography is essential in the context of DNA separation, as it plays a key role in purifying nucleic acids which is crucial for further analysis and experimentation.
The benefits of chromatography include its precision and ability to separate complex mixtures, making it advantageous for applications in genetics, forensic science, and biochemistry. Additionally, the versatility of chromatography allows it to be adapted for different types of analysis, which is beneficial in research settings where varied sample types are encountered.
Basic Principles of Chromatography
Chromatography operates on fundamental principles of partitioning and adsorption. The technique involves passing a mixture through a medium, where different components move at different rates. This differential movement occurs due to varying affinities for the stationary phase, which can be solid or liquid, and the mobile phase, which is usually liquid or gas. The principle underlining this separation is that molecules will travel through the medium based on their size, charge, and other chemical properties, leading to the formation of distinct layers or bands.
Different Types of Chromatography
Chromatography has evolved into several distinct types, tailored to specific research needs. Two prominent forms are:
- Liquid Chromatography: This form uses liquid as the mobile phase. Liquid chromatography is particularly significant in DNA separation due to its effectiveness in isolating nucleic acids with high resolution. A key characteristic of this method is its adaptability to various sample sizes and compositions. It is beneficial for separating small and large DNA fragments alike, making it a popular choice in molecular biology labs. The unique feature of liquid chromatography lies in its capacity to incorporate various detection methods, such as UV absorbance and fluorescence, enhancing its utility in complex analyses. However, one of its disadvantages may include longer run times compared to other methods, requiring careful consideration in high-throughput environments.
- Gas Chromatography: This method, as the name suggests, uses gas as the mobile phase. While not traditionally used for DNA due to stability concerns, it does have significant applications in analyzing volatile and low-boiling compounds in biological samples. Gas chromatography is distinguished by its extreme efficiency and speed over liquid methods. However, its main limitation in DNA applications is the requirement for vaporization, which can alter sensitive nucleic acid structures.
Applications in DNA Separation
The applications of chromatography in DNA separation are vast and impactful. In forensic science, chromatography allows the extraction and analysis of DNA from crime scene samples, ensuring reliable results. In genetic research, it provides a means to purify DNA fragments needed for cloning or sequencing. In clinical diagnostics, chromatography assists in identifying genetic disorders by enabling the separation of DNA variants that may indicate specific conditions. Understanding these applications highlights the critical role chromatography plays in advancing both scientific discovery and practical applications in health and law enforcement.
Centrifugation Techniques
Centrifugation is a critical technique in the field of molecular biology and biochemistry. It involves the use of a centrifuge to separate components of a mixture based on their density. This section provides a detailed examination of centrifugation techniques, highlighting their principles, types, and considerations. Understanding these elements is essential for researchers and practitioners who engage in various scientific investigations involving DNA separation.
Principles of Centrifugation
Centrifugation operates on the principle of centrifugal force. When a sample is spun in a centrifugal machine, denser components move outward and settle at the bottom, while lighter components remain suspended in the liquid. This process can effectively isolate specific DNA fragments or cellular components, depending on the density differences among substances.
The fundamental equation that captures this principle is:
F = m * a,
where F represents the force applied to the sample, m is its mass, and a is the acceleration. By increasing the rotor speed and duration, one can enhance the separation efficiency, but care must be taken to prevent damage to the samples.
Types of Centrifugation
There are several distinct types of centrifugation techniques, each tailored to specific applications:
- Differential Centrifugation: This process sequentially applies different force levels and is often utilized for cell fractionation.
- Density Gradient Centrifugation: Here, the sample is placed in a tube that contains a gradient of density, allowing for precise separation based on buoyancy.
- Isopycnic Centrifugation: A more advanced technique, it separates particles until they reach a point within the gradient where their density matches the surrounding medium.
Centrifugation can also be classified based on equipment features:
- Tabletop Centrifuges: Common in labs for routine experiments.
- Ultracentrifuges: These are used for high-speed applications that require extreme conditions for separation.
Advantages and Limitations
Centrifugation techniques offer several advantages:
- High Efficiency: Allows for rapid separation of components, saving time in research and clinical settings.
- Versatility: Suitable for diverse samples, from blood to environmental samples.
- Scalability: Methods can be adjusted to handle small or large quantities of material.
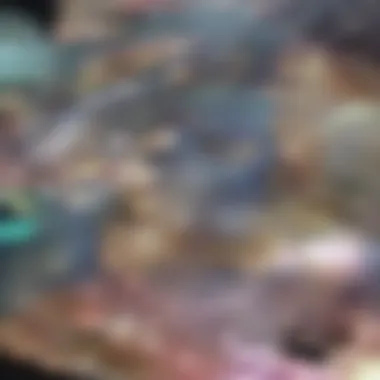
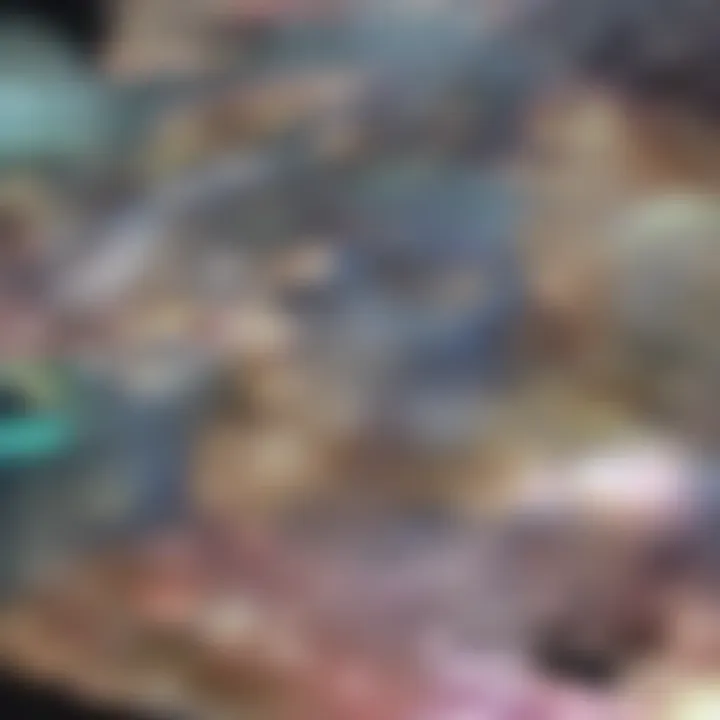
However, there are limitations to consider:
- Potential for Shear Stress: The mechanical forces can damage sensitive biological materials, particularly DNA and proteins.
- Equipment Cost: Ultracentrifuges, in particular, can be expensive and require regular maintenance.
- Skill Requirement: Proper execution of techniques often necessitates trained personnel to ensure optimal results.
"Centrifugation can significantly influence the purity of DNA samples, affecting the success of downstream applications such as PCR and sequencing."
Understanding both the advantages and limitations is crucial for those engaged in DNA research. Knowledge in this area can enhance experimental design and reliability in results.
Challenges in DNA Separation
The process of DNA separation is fundamental to various scientific domains, yet it is not without its challenges. Understanding these challenges is crucial, as they can impact the reliability and effectiveness of DNA analysis. Addressing issues like contamination and technical limitations can significantly improve outcomes in fields such as genetics, forensic science, and medical research.
Contamination Issues
Contamination is a major concern in DNA separation. It can stem from multiple sources, including environmental factors, laboratory practices, and even the materials used in the separation process. As DNA is highly sensitive and can easily degrade, even minute traces of other DNA can compromise results. This interference can lead to misleading conclusions, particularly in forensic investigations where accuracy is vital.
To minimize contamination:
- Implement strict laboratory protocols: Regularly sterilizing equipment and using dedicated tools for each sample can help prevent cross-contamination.
- Employ proper storage techniques: Samples should be stored under controlled conditions to prevent degradation or contamination from external sources.
Trained personnel can also significantly reduce the risk of contamination, emphasizing the importance of human factors in the laboratory setting.
Technical Limitations
Technical limitations can further hinder effective DNA separation. Various separation techniques may come with their own set of constraints, affecting the purity and yield of the separated DNA. For example, the choice of method, such as gel electrophoresis or centrifugation, can influence both the resolution and separation time. Some methods may not be suitable for specific DNA fragments, leading to incomplete separation or poor resolution.
Moreover, the equipment quality and maintenance are critical. Outdated or improperly calibrated equipment can produce unreliable results. Additionally, there may be challenges related to:
- Resolution power: Some techniques may not distinguish between DNA fragments that are very close in size.
- Scalability: In workflows that require handling numerous samples, some methodologies may prove inefficient or resource-intensive.
Organizations must carefully evaluate the techniques they utilize to ensure they align with research objectives. Being aware of these technical considerations can help researchers choose the most appropriate method for their specific needs.
Ethical Considerations
In the field of DNA separation, ethical considerations are critical. As techniques evolve and become more sophisticated, the potential for misuse and ethical dilemmas increases. Ethical guidelines help safeguard individuals' rights and maintain trust in scientific practices. It's essential to address issues surrounding consent, privacy, and the broader implications of genetic testing and research.
Privacy and Consent
Privacy is a fundamental concern when dealing with personal genetic information. The collection of DNA samples often occurs in sensitive contexts, such as medical diagnostics or forensic investigations. Individuals may not always fully understand how their DNA will be used, shared, or stored.
Consent must be informed. Participants in research or medical settings should be aware of the purposes of DNA extraction. They must consent voluntarily without coercion. It is crucial that researchers and practitioners explain the risks and benefits clearly. For example, a person might agree to provide a DNA sample for research but later regret it if that data gets shared publicly or used in unexpected ways.
To address these issues, clear protocols need to be established.
Some key points for ethical management include:
- Transparent communication about how DNA will be used.
- Options for individuals to withdraw their consent at any time.
- Secure storage practices to prevent unauthorized access to sensitive information.
Implications for Genetic Research
The impact of ethical considerations extends beyond consent and privacy. They play a significant role in shaping the direction of genetic research. Researchers must tread thoughtfully when interpreting the results of genetic studies. The potential for genetic discrimination is a concern. For instance, employers or insurance companies may misuse genetic data to deny opportunities based on an individual's genetic predisposition to certain diseases.
Adhering to ethical standards fosters a responsible approach to research. It promotes trust between researchers and participants, enhancing the quality and integrity of the research. Continued dialogue among scientists, ethicists, and the public creates a transparent atmosphere. This transparency is vital for navigating the complex landscape of genetic research and ensuring equitable treatment of individuals.
"The ethical dimensions of genetic research cannot be overstated. They shape the future of science and public perception."
Overall, ethical considerations in DNA separation and genetic research are paramount. They ensure that as the field advances, it does so with respect for individuals and society. Establishing a foundation of trust and integrity is essential for the future of genetic studies. Addressing these concerns thoughtfully will influence public acceptance and the ethical progress of science.
Future Directions in DNA Separation
Future directions in DNA separation hold great promise for advancements across various fields. As technology improves, the potential applications and implications of these methods expand significantly. This section will explore key technological innovations and their impact on precision medicine.
Technological Innovations
Several exciting technological innovations are on the horizon in DNA separation techniques. These innovations aim to enhance efficiency, accuracy, and ease of use. Some notable developments include:
- Next-Generation Sequencing (NGS): NGS methods are revolutionizing DNA separation by allowing scientists to sequence entire genomes rapidly and accurately. This prompts easier data analysis and broader applications in research.
- Microfluidics: This method uses tiny channels to manipulate small volumes of fluids. Microfluidics can improve the speed and precision of DNA separation, which is crucial in clinical settings.
- Automation: Lab automation technologies are streamlining DNA separation processes, reducing human error and increasing throughput. Automated systems can handle various samples with minimal human intervention, improving consistency.
- Real-time monitoring: Techniques that allow for real-time observation of separation processes are becoming more prevalent. This provides researchers valuable data on separation efficiency and integrity.
These innovations are not just about enhancing existing techniques. They may lead to the development of entirely new methodologies that could redefine how DNA is analyzed and understood.
Impact on Precision Medicine
The implications of advancements in DNA separation techniques for precision medicine are significant. Precision medicine seeks to tailor treatments based on individual genetic profiles. Thus, effective DNA separation is vital to accurately analyze patients' genetic information.
- Targeted therapies: With improved DNA separation methods, doctors can identify specific mutations that drive certain diseases. This enables them to prescribe targeted therapies rather than general treatments, increasing the likelihood of success.
- Personalized diagnostics: Advanced separation techniques can enhance diagnostic accuracy. By reliably isolating specific DNA segments, healthcare providers can develop more precise tests that inform treatment decisions.
- Genomic data integration: Innovations in technology can facilitate the integration of DNA separation results with broader genomic data. This is powerful for understanding complex diseases and developing comprehensive treatment strategies.
"The transformation in DNA separation technologies will drive the next wave of personalized medicine, providing tailor-made therapies that were previously unimaginable."
The End
The exploration of DNA separation techniques is essential for understanding their relevance and application across various fields. This article has provided a comprehensive overview of the methodologies utilized in DNA separation, focusing on their significance in biological research and forensic science. By summarizing critical techniques such as gel electrophoresis, chromatography, and centrifugation, we highlight not only how these methods work but also why they are vital to scientific inquiry.
Summary of Key Points
- Techniques Overview: Various methods, including gel electrophoresis and chromatography, play key roles in isolating and analyzing DNA.
- Applications Across Fields: DNA separation is not confined to a single discipline; it impacts forensic science, medicine, and genetic research.
- Ethical Considerations: As DNA technology progresses, we must address ethical concerns regarding privacy and consent.
- Future Innovations: Advancements in technology will likely enhance the precision and efficacy of DNA separation protocols, influencing precision medicine significantlly.