RNA Sequencing Library Preparation: Key Insights
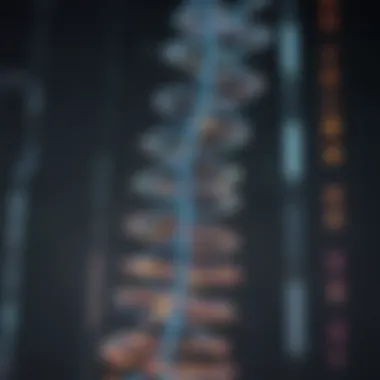
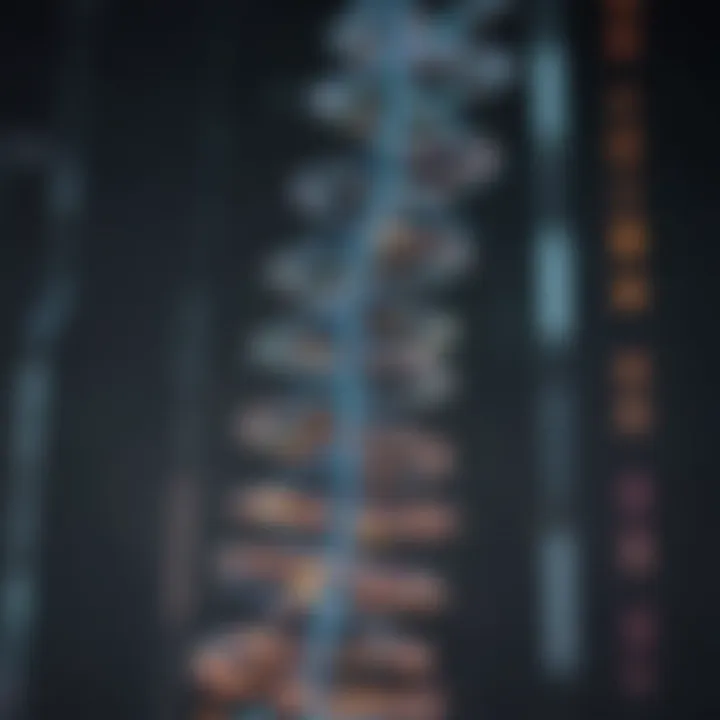
Intro
The landscape of genomics has been profoundly transformed by RNA sequencing, which provides an intricate view into the transcriptome. This technique is no longer a mere option in a researcherβs toolbox; it has harnessed the limelight due to its unmatched ability to elucidate gene expression patterns and their variations across conditions. In this article, we will weave through the layers of RNA sequencing library preparation, showcasing the steps involved, the technological advancements that have emerged, and the significance of these processes in biological research.
As we delve deeper into the substance, we must recognize the relevance of this exploration. The seamless integration of RNA sequencing into genomic and transcriptomic studies highlights a growing need for a comprehensive understanding. This article caters to students, researchers, educators, and professionals keen on grasping the nuances of RNA Seq technologies. Through an unvarnished exploration of the methodologies, findings, and future trajectories, we aim to provide not only clarity but also a robust foundation for further learning and advancement in this dynamic field.
Research Overview
Summary of Key Findings
The initiatives in RNA sequencing have birthed several critical discoveries and methodologies. These findings are not confined to mere technical enhancements; they reflect groundbreaking insights into how genes are expressed, regulated, and how they interact with one another.
- Increased Sensitivity: High-throughput sequencing technologies have enhanced the sensitivity of RNA detection across a broader dynamic range.
- Alternative Splicing: RNA sequencing reveals diverse isoform expressions, shedding light on alternative splicing events that were previously elusive.
- MicroRNA Discovery: The methodology has facilitated identifying numerous microRNAs, crucial players in gene regulation.
"Understanding RNA sequencing is pivotal for unraveling the complexities of gene expression and its implications in health and disease."
Methodologies Employed
The road to proficient RNA sequencing begins with meticulous planning of the experimental setup, involving several recommended techniques:
- Sample Preparation: Initially, the quality of the RNA sample is paramount. Using kits like the RNeasy Plus Mini Kit or the TRIzol method facilitates extraction with minimal degradation.
- Library Construction: This step involves fragmenting the RNA and adding adapters. Various approaches, such as poly-A enrichment for mRNA or total RNA-seq for non-coding regions, are commonly employed.
- Sequencing Techniques: Platforms like Illumina NextSeq or Ion Torrent offer varying read lengths and throughput levels, catering to different project scales.
In-Depth Analysis
Detailed Examination of Results
The evolution of RNA sequencing methodologies has yielded rich datasets that invite critical analyses. As researchers sift through mounds of data, the focus lies on interpreting biological significance rather than just numeric outputs. Recent studies indicate an unexpected correlation between gene expression levels and disease states, opening new avenues for therapeutic interventions.
Comparison with Previous Studies
Historically, methods like microarray analysis had limited sensitivity and specificity. In contrast, RNA sequencing lays bare the complexities of the transcriptome, offering a granular view previously unattainable. Discerning the differences:
- Resolution: RNA seq allows detection of low-abundance transcriptsβessential for understanding subtle biological changes.
- Scope: Unlike its predecessors, it offers insights into both known and novel transcripts, broadening the canvas for discovery.
Prelude to RNA Sequencing
RNA sequencing, or RNA-seq, has become a cornerstone in modern biological research, offering a powerful means to delve into the complexities of gene expression, regulation, and the overall dynamics of the transcriptome. The rise of RNA sequencing methodologies has transformed how scientists approach tasks like understanding diseases or studying developmental biology, making this section essential for grasping both the historical context and the relevance of RNA-seq in contemporary studies.
The Evolution of RNA Sequencing Techniques
The journey of RNA sequencing techniques is as fascinating as it is revolutionary. Initially, traditional methods like Northern blotting and RT-PCR provided limited insights into gene expression. However, these techniques often fell short in terms of high throughput and sensitivity. Then came the advent of next-generation sequencing (NGS), which dramatically changed the landscape of molecular biology. With NGS, scientists could sequence millions of RNA fragments simultaneously, allowing for comprehensive snapshots of cellular transcriptional activity.
In the early days of RNA-seq, the focus primarily lay in measuring gene expression levels. As the techniques matured, researchers began to explore other dimensions. For instance, approaches started to evolve that provided insights into alternative splicing, the identification of novel transcripts, and the exploration of non-coding RNAs. Today, techniques have advanced to include single-cell RNA sequencing, which examines gene expression at an individual cell level, paving the way for unprecedented discoveries in cell heterogeneity and function.
One pivotal aspect in the evolutionary timeline is the rise of automation in library preparation and sequencing. This aspect has led to a reduction in costs and increased accessibility, empowering more labs to engage in RNA-seq experiments. The ingenuity of scientists to adapt to and improve RNA sequencing tools has kept the field vibrant and dynamic.
Significance of RNA Sequencing in Modern Biology
In modern biology, RNA sequencing's significance cannot be overstated. It serves as a critical tool not just for studying basic cellular processes, but also for exploring complex biological phenomena. For example, in the realm of biomedical research, RNA-seq has shed light on the molecular underpinnings of various diseases, including cancer and neurodegeneration. By analyzing what genes are active β or silenced β within diseased tissues, researchers can identify potential therapeutic targets.
Moreover, RNA sequencing is instrumental when studying developmental biology. Understanding how gene expression changes over time can provide insights into crucial processes such as differentiation and morphogenesis. Environmental factors affecting gene expression can also be studied through RNA-seq, linking genetics to ecological adaptations.
As highlighted,
"RNA sequencing isnβt just a tool; itβs a window into the living systems, showing us the intricate tapestry of life at a molecular level."
Furthermore, RNA-seq has applications beyond academia, extending into agricultural and environmental science. It plays a role in developing crop varieties with enhanced traits, such as drought resistance or improved nutritional content, thus contributing to food security.
In summary, the introduction of RNA sequencing opens a gateway to multifaceted exploration in biology. Its evolution from basic gene expression studies to a multifarious tool integrating various biological questions marks a significant advancement in understanding life itself.
Understanding RNA and Its Types
The role of RNA in modern biology cannot be understated. It is essential for understanding how cells function, how genes are expressed, and how various biological processes are orchestrated through nuanced interactions within cellular environments. In RNA sequencing studies, grasping the types of RNA and their respective functions is crucial. RNA doesn't just act as a messenger. It has various facets and influences on the biological landscape, making it a subject of intrigue for many researchers.
Types of RNA: mRNA, tRNA, rRNA, and Beyond
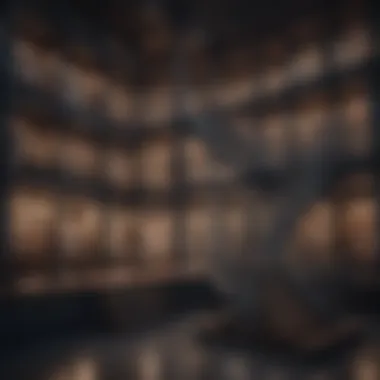
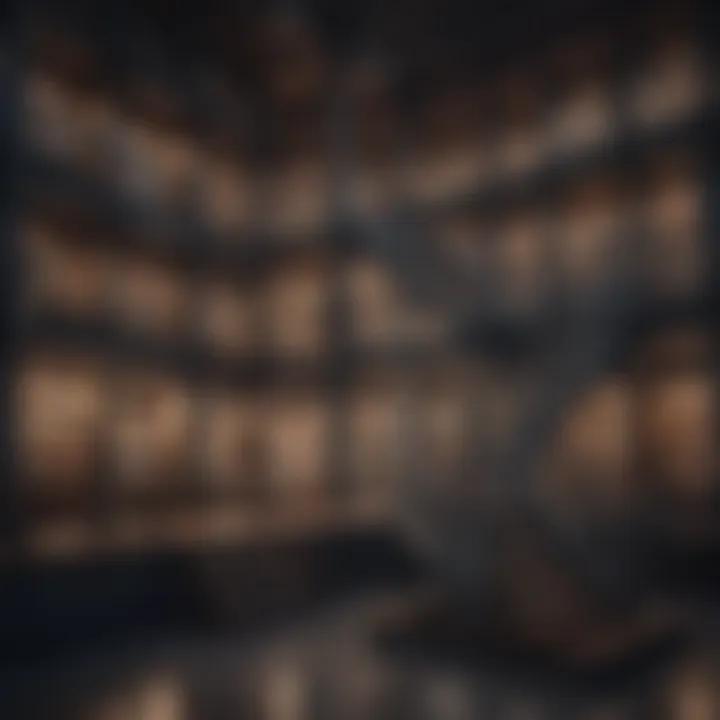
To really dissect RNA's role in cellular functions, one must consider its diverse types. The three principal types of RNA that grab headlines in many studies are messenger RNA (mRNA), transfer RNA (tRNA), and ribosomal RNA (rRNA). Each of these has distinct roles in the grand scheme of life.
- mRNA (Messenger RNA): This type of RNA carries genetic information from DNA to the ribosomes, where proteins are synthesized. Itβs often described as the blueprint for protein synthesis. When a gene gets activated, it while be transcribed into mRNA, leading the cellular machinery to produce specific proteins which are required for various functions within the body.
- tRNA (Transfer RNA): Think of tRNA as the worker bees of protein synthesis. They transport amino acids to the ribosome, where they match with the mRNA template. This pairing ensures that amino acids are added in the correct order, crafting polypeptides and ultimately proteins.
- rRNA (Ribosomal RNA): This type forms the core of ribosome's structure and also aids in the catalytic process of protein synthesis. Surprising? Yes, but rRNA is not merely structural; it's essential for the functionality of ribosomes, serving as a facilitator that coordinates mRNA and tRNA interaction during protein synthesis.
Beyond these, there are numerous other RNAs, such as small interfering RNA (siRNA) and long non-coding RNA (lncRNA), each contributing in distinct ways. For example, siRNA plays a vital part in gene silencing and regulation, whereas lncRNA is more of a regulatory element that can influence various pathways and processes.
Functional Roles of RNA in Cellular Processes
The functionality of RNA extends far beyond simple protein coding. RNA molecules are involved in numerous cellular mechanisms, serving as intermediaries and regulators in complex biological pathways.
RNA is not merely a passive messenger; it's a dynamic player in cellular orchestration.
- Gene Regulation: Various RNA types interact with DNA and other RNA molecules to modulate gene expression. They can enhance or silence the expression of genes based on the needs of the cell.
- Catalysis: Certain RNA molecules can even participate in catalysis, making them quite creative in functions traditionally thought to be limited to proteins. For instance, some ribozymes, which are RNA molecules with catalytic functions, can catalyze specific biochemical reactions.
- Signal Transduction: RNA can act as a signaling molecule, which influences various pathways. This is a growing area of research that uncovers how RNA serves functions similar to hormones and other signaling molecules within cells.
The understanding of these roles aids scientists in leveraging RNA for innovations in medicine and biotechnology. With advancements in RNA sequencing techniques, it's becoming ever clearer how these molecules dictate a vast array of biological processes, shaping the way we comprehend cell biology and genetics.
Library Preparation: An Overview
Library preparation in RNA sequencing is a critical step that sets the stage for accurate, reproducible results. It entails transforming RNA samples into a form that can be amplified and sequenced, allowing for detailed analysis of gene expression and transcriptomic variations. In recent years, as technologies and methodologies have advanced, the significance of this process has become more pronounced, making it essential for researchers and practitioners alike.
Key Steps in RNA Seq Library Preparation
The library preparation process can be broken down into several fundamental steps, each with a direct impact on the quality and clarity of the data obtained. Understanding these components is key for those looking to maximize the output of RNA sequencing experiments.
Sample Collection and RNA Extraction
Sample collection and RNA extraction are the cornerstones of any RNA sequencing project. The reliability of downstream analyses heavily depends on the quality of the RNA extracted. A key characteristic in this phase is the timing and method of sample collection. Freshness is paramountβsamples need to be collected and processed quickly to minimize RNA degradation, which can skew results.
Different extraction techniques, such as TRIzol extraction or column-based methods, offer varied benefits. For example, TRIzol is advantageous because it can yield high RNA purity, but this might come at the cost of time and labor.
- Pros: High purity and yield with proper techniques.
- Cons: Requires immediate processing to prevent degradation.
RNA Quality Assessment
After extraction, RNA quality assessment becomes vital. Techniques like spectrophotometry and gel electrophoresis are commonly employed to evaluate RNA integrity. One of the standout features of this step is the use of the RNA integrity number (RIN), which provides a quantifiable measure of RNA quality.
Assessing RNA quality isn't just about ensuring that the sample is intactβit impacts the entire sequencing exercise. A low-quality RNA sample can lead to poor library construction and ultimately deceptive results. Researchers often favor this assessment as it allows for quality control before proceeding to the next phase.
"Assessing RNA quality can save researchers from embarking on potentially flawed experiments, ensuring results are both reliable and reproducible."
- Pros: Clear and quantifiable measure of quality.
- Cons: Additional time and resources needed for assessment.
Library Construction Techniques
Library construction techniques vary widely and can significantly affect the outcomes of RNA sequencing. Common methods include the ligation of adapters to RNA fragments or using poly-A selection to enrich for mRNA. Each technique has its own implications for the sensitivity and specificity of RNA detection.
The uniqueness of library construction can help tailor experiments for specific goals. For instance, specific methods may be chosen for single-cell RNA sequencing versus bulk sequencing due to differences in the goals of the analysis. The selection process, therefore, requires careful consideration of the experimental aims.
- Pros: Tailored approaches can enhance sensitivity.
- Cons: Some methods may introduce biases that affect comprehensiveness.
Choosing the Right Method for Library Preparation
Selecting the most suitable method for library preparation is an essential decision that can influence the success of an RNA sequencing project. Factors to consider include compatibility with the sample type, research objectives, and the required sensitivity levels. Thus, weighing the characteristics and pros and cons of each technique will equip researchers to make informed choices that enhance the reliability of their findings.
Advancements in Library Preparation Technologies
The arena of RNA sequencing library preparation has seen a whirlwind of innovations over recent years. These advancements are not just minor tweaks but pivotal changes that can redefine the efficacy and efficiency of sequencing projects. The significance of these improvements cannot be overstated, as they can greatly impact research outcomes, influencing everything from data quality to cost-effectiveness.
Next-Generation Sequencing Platforms
The advent of next-generation sequencing (NGS) platforms has revolutionized the way we approach RNA sequencing. Unlike traditional Sanger sequencing, which is labor-intensive and time-consuming, NGS allows for massively parallel sequencing. It means that thousands to millions of fragments can be sequenced simultaneously.
- High Throughput: This capability enables researchers to delve deeper into the transcriptome, capturing a broader spectrum of RNA species, including rare transcripts that might otherwise go unnoticed.
- Cost Efficiency: In the past, sequencing came with a hefty price tag. With NGS, costs have relaxed significantly, making large-scale studies more feasible.
- Speed: The turnaround time for sequencing results has drastically reduced, allowing for quicker data analysis and interpretation.
The adoption of specific NGS platforms such as Illumina, Ion Torrent, or Pacific Biosciences further illustrates the diversity of options available to researchers today. Each platform comes with its unique attributes, so understanding factors such as read length, error rates, and throughput becomes essential to choose the right platform for a given project.
Innovative Techniques in Library Preparation
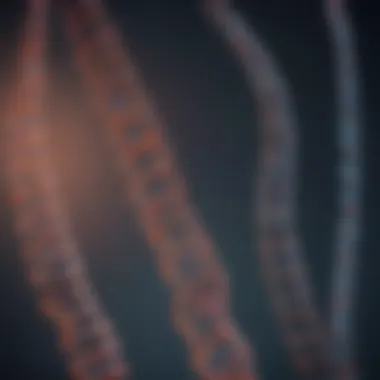
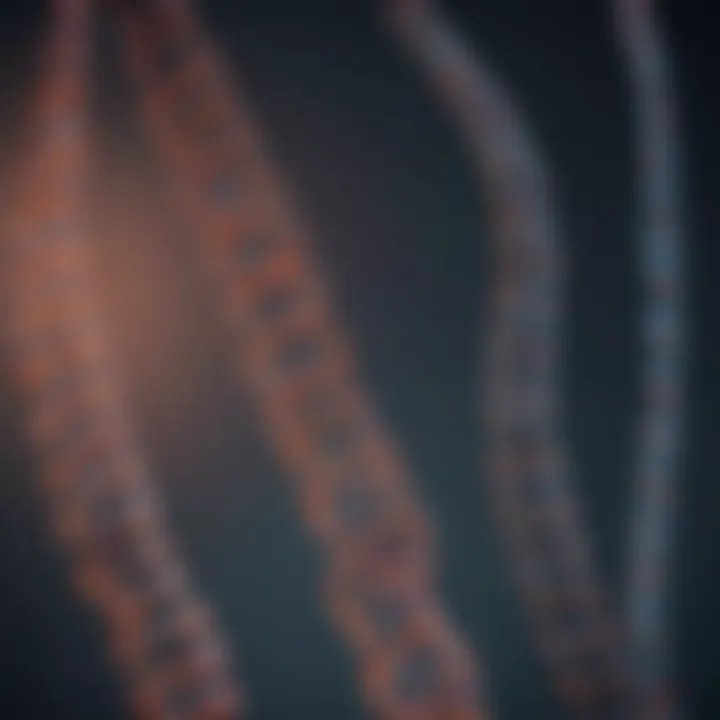
Not just the sequencing speeds or costs have taken a leap, but the methods for library preparation themselves have evolved significantly. Different innovative techniques in library preparation are now at oneβs disposal, ensuring that the library is well-prepared to yield informative results.
- Poly(A) Enrichment vs. Ribosomal RNA Depletion: The choice between these methods depends largely on the study's goals. For studies focused on coding regions, poly(A) enrichment could be preferred. Conversely, if one is interested in the broader landscape of RNA, including non-coding RNAs, ribosomal depletion may be more appropriate.
- Single-Cell RNA Sequencing: This technique has emerged as a game-changer, allowing researchers to study gene expression at the single-cell level. It uncovers heterogeneity that is often masked in bulk RNA sequencing where the average expression profile of many cells is captured.
- Targeted Sequencing: Another new avenue is target enrichment techniques, enabling researchers to focus on specific genes or genomic regions of interest, which can be incredibly useful in clinical and translational research settings.
Epilogue of Advancements
Advances in library preparation technologies not only streamline workflows but also enhance analytical power. As RNA sequencing continues to mature, keeping abreast of these technologies is not just beneficial; it is essential for staying ahead in the competitive world of genomic research. Through judicious selection of technologies and methods, researchers can unleash the full potential of RNA sequencing, paving the way for ground-breaking discoveries in biology.
Quality Control in RNA Seq Libraries
Quality control in RNA sequencing libraries is a critical aspect of the research process, serving as a gatekeeper for ensuring the reliability and accuracy of downstream results. Proper quality assessment prevents the leakage of erroneous data into studies. When researchers invest considerable time and resources to gather samples and prepare libraries, one misstep can throw everything into disarray. This section will delve into the specific elements that make quality control indispensable in RNA sequencing, the benefits it bestows, and key considerations that researchers must keep in mind.
Importance of Quality Assessment
The strength of conclusions drawn from RNA sequencing experiments hinges on the quality of the libraries generated. The assessment of RNA quality is paramount; it dictates whether the library will yield meaningful insights or if it will be mired in inaccuracies. Poor-quality RNA can lead to low sequencing yield, uneven representation of transcripts, and can obscure essential biological information.
Moreover, quality assessment provides a comparative backdrop. For instance, in experiments where multiple samples are sequenced, discrepancies in quality can skew interpretations. It's essential for something as intricate as transcriptional profiling to ensure that each sample is treated on an equal footing.
In this context, one often hears seasoned researchers remark, "Garbage in, garbage out." This adage rings especially true for RNA sequencing; only high-quality input can produce high-quality data.
Methods for Quality Control
Quality control in RNA sequencing entails several methodologies to ensure that the prepared libraries meet established standards. Below are some of the most widely employed techniques:
- Spectrophotometry - This technique allows researchers to assess RNA concentration and purity. By measuring absorbance at specific wavelengths (typically 260 nm for RNA), they can estimate the quality of the RNA in question.
- Bioanalyzer or TapeStation - These platforms provide a graphical visualization of RNA quality by generating an electropherogram. Users can ascertain RNA integrity number (RIN), a quantitative measure of RNA quality, enabling better decision-making regarding which samples to proceed with.
- qPCR Analysis - Quantitative PCR can evaluate the efficiency of cDNA synthesis from RNA samples, thus indirectly assessing the quality of the initial RNA. If cDNA amplification is erratic or below expectations, this indicates problems in the RNA source material.
- Gel Electrophoresis - Although traditional, this method is still a robust way to visualize RNA integrity and size distribution. It allows researchers to see the presence of ribosomal RNA bands, indicating whether RNA samples are intact.
- Libraries' Coverage Assessment - After sequencing, evaluating the read distribution across genomic regions helps identify biases. An adequate and uniform coverage of transcriptomes is a hallmark of a reliable sequencing library.
"Quality control isnβt just a box to check; itβs a fundamental part of ensuring the integrity of scientific inquiry."
In summary, the importance of quality assessment and the methods employed to maintain these standards forms the bedrock of credible RNA sequencing research. It's an essential investment that pays off by providing researchers with reliable data and insights, which ultimately advances the frontiers of biological science.
Experimental Design in RNA Sequencing
The realm of RNA sequencing is not merely confined to the technical aspects of data generation; the experimental design plays a pivotal role in ensuring the reliability and interpretability of results. A well-thought-out experimental design helps delineate clear objectives, ensures the validity of findings, and maximizes the potential for insightful analyses. From choosing the right sample characteristics to the methods employed for sequencing, each decision impacts the overall success of RNA sequencing projects.
Factors to Consider in Experimental Design
Sample Size and Replication
Sample size and replication are two cornerstones of effective experimental design in RNA sequencing. When it comes to sample size, it directly influences the statistical power of the experiment. A larger sample size generally increases the robustness of the conclusions drawn, as it minimizes the impact of outliers and random variance. For instance, imagine studying the expression levels of a set of genes across various treatments. If the sample size is too small, the results may paint a misleading picture, populating your findings with noise instead of clarity.
The key characteristic here is reliability. By increasing the sample size, researchers can have greater confidence in identifying true biological effects. Additionally, replication β repeating experiments with separate biological samples β is equally vital as it reinforces results, establishing reproducibility.
However, there's a unique feature to consider: the logistical and financial constraints that accompany larger sample sizes. It can be a double-edged sword. While larger samples yield better results, they also mean more effort and possibly higher costs. Balancing these aspects often requires careful planning and resource management to achieve beneficial outcomes without unnecessary strain on resources.
Control Conditions
Control conditions are the backbone of any scientific experiment and RNA sequencing is no exception. The presence of control groups allows researchers to establish a baseline, making it easier to interpret how experimental conditions affect RNA expression profiles. Consider the scenario β when evaluating the impacts of a new drug on gene expression, having a control group that does not receive the treatment helps attribute changes specifically to the drug rather than other external factors.
The significance of control conditions lies in their ability to eliminate confounding variables, providing a clearer picture of the treatment effects. In this context, controls facilitate direct comparisons, enhancing the validity of findings. One unique aspect is the incorporation of negative and positive controls, which together ensure that any observations can be thoroughly examined and validated.
While controls provide an essential part of the research design, there can be challenges as well. In some cases, establishing appropriate control conditions may be cumbersome or complex, particularly in heterogeneous biological systems. Researchers must navigate the potential inadequacies in sampling and setup to establish a reliable control that truly reflects the conditions being studied.
Challenges in Experimental Design
Despite the thoughtful considerations pertaining to sample size, replication, and control conditions, experimental design in RNA sequencing is fraught with challenges. One major consideration is the variability within biological systems. Biological samples can be influenced by numerous factors such as age, health status, and even environmental conditions. This variability can lead to inconsistency, requiring extensive pilot studies to grasp the nuances of the samples being utilized.
Another significant challenge is the integration of advanced technology. As sequencing techniques evolve, keeping up with rapidly changing methodologies and ensuring the best practices can strain resources and expertise.
Furthermore, the complexity of data analysis paired with an intricate experimental setup can sometimes lead to unforeseen complications. Careful navigation through these obstacles is essential to maximize the output of RNA sequencing endeavors. It's a tightrope walk, requiring clarity of thought, precision in execution, and adaptability to changing circumstances to yield meaningful results.
Data Analysis in RNA Sequencing
When it comes to RNA sequencing, data analysis is an absolutely pivotal part of the process. It's not just about the sequence itself; it's what can be learned from it that truly matters. This section will unpack the essentials of data analysis, diving into the steps involved and highlighting its role in elucidating complex biological phenomena. Proper analysis transforms raw data into meaningful insights, which can lead to groundbreaking discoveries in genomics and beyond.
Overview of Data Analysis Pipeline
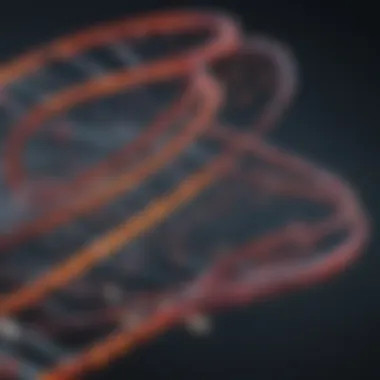
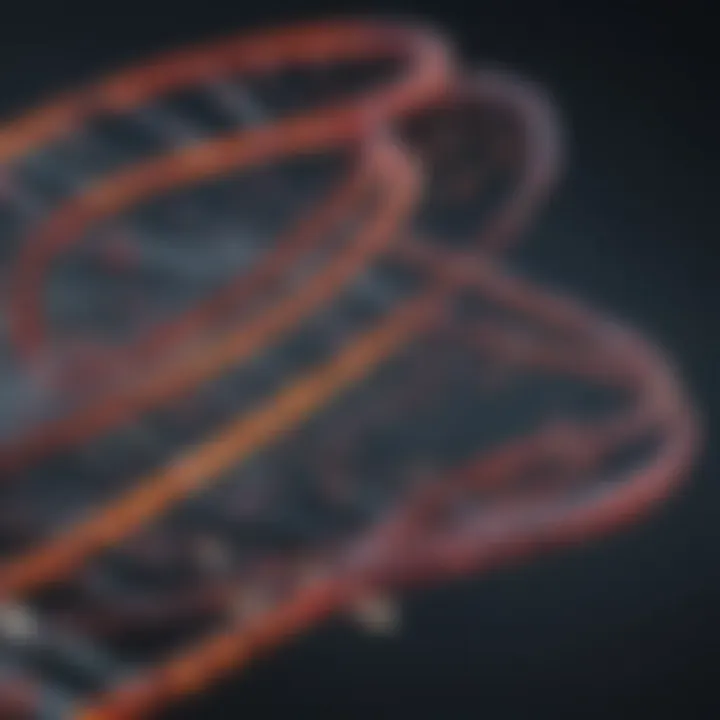
The data analysis pipeline in RNA sequencing encompasses several critical stages. Each step plays a role in refining raw sequence data into actionable information. Hereβs a breakdown of these major components:
- Quality Control: Before diving deep into the analysis, it's crucial to check the quality of the raw data. Tools like FastQC can provide a snapshot of potential issues, such as low-quality bases or contamination.
- Trimming and Filtering: Data often requires trimming to remove adapter sequences and low-quality reads. This step enhances the overall quality of the input data to subsequent analyses.
- Alignment: The high-quality reads are then aligned to a reference genome or transcriptome using algorithms like STAR or HISAT2. This is a significant step that dictates how well your data interprets the biological scenario.
- Quantification: Once aligned, the next stage involves quantifying the expression levels of genes or transcripts. Tools such as featureCounts or HTSeq count reads that align to specific features, which lets researchers gauge gene activity.
- Normalization: To compare across samples fairly, normalization techniques adjust for systematic biases in sequencing depth, allowing for more accurate comparisons between samples.
- Statistical Analysis: This step assesses differential expression among groups. Bioconductor's DESeq2 offers robust methods for identifying genes that are expressed at different levels between conditions.
- Visualization: Finally, visual representation of the findings is key. Tools like ggplot2 in R or even specialized platforms can help illustrate complex data through heatmaps, volcano plots, and other formats.
In brief, this multi-step pipeline is a finely tuned machine. Each cog in the gear works in conjunction to ensure data integrity and reliability, which is foundational for trustworthy conclusions.
Interpreting RNA Seq Data
Interpreting RNA sequencing data is an art as much as it is a science. After all the hard work of aligning and quantifying reads, the real challenge lies in making sense of what it all means. Here are some factors that come into play:
- Biological Context: The interpretation should always consider biological relevance. Gene expression levels can vary significantly based on contextual factors like tissue type, developmental stage, or environmental conditions. For instance, a gene that is highly expressed in cancer tissue may have a minor role in healthy tissue.
- Functional Annotation: Using databases like Gene Ontology (GO) or KEGG can help researchers understand what the significant changes in expression may imply functionally. Being able to link expression data to known pathways can provide insight into disease mechanisms or biological processes.
- Comparative Analysis: Comparing data across different conditions enhances understanding. What differences emerge when a particular gene is knocked out? Or how does gene expression fluctuate under stress? These insights can lead to pivotal breakthroughs.
- Integrative Approaches: Often, integrating RNA-seq data with other omics data such as proteomics can offer a more comprehensive view. By marrying the expression data with protein level information, one can identify discrepancies that might indicate regulation at various levels.
Understanding RNA sequencing data isn't just technical; it's about infusing meaning into the numbers. By dissecting and contextualizing results, researchers can pave the way for advancements in both basic science and clinical applications.
Applications of RNA Sequencing
RNA sequencing serves as a powerful tool in molecular biology, providing a window into the intricacies of gene expression and regulation. The ability to analyze the RNA components of a cell offers unique insights into how organisms adapt, develop, and interact with their environment. The applications of RNA sequencing are vast, encompassing various fields that significantly impact both our understanding of biology and the potential for advancements in medicine.
Biomedical Research and Disease Understanding
In the realm of biomedical research, RNA sequencing is indispensable. It allows scientists to uncover how different diseases, like cancer, manifest at the molecular level. By examining the differences in gene expression between healthy and diseased tissues, researchers can identify specific genes or pathways that become dysfunctional. This understanding paves the way for:
- Targeted Therapies: Knowing which genes are overexpressed or underexpressed in a tumor can guide the development of targeted treatments. For instance, if a specific mutation drives cancer cell growth, therapies can be designed to inhibit this mutation.
- Biomarkers for Disease: RNA-seq can identify novel biomarkers. These markers can assist in early diagnosis or prognosis of diseases, providing critical information for treatment decisions.
- Insights into Disease Mechanisms: Exploring differential gene expression can reveal how diseases progress. This knowledge helps in formulating new hypotheses and understanding disease pathways.
"RNA sequencing not only uncovers the what but also delves into the why and how of gene regulation in health and disease."
Environmental and Ecological Studies
The applications of RNA sequencing extend beyond human health, reaching into environmental and ecological realms. Here, RNA sequencing helps scientists understand the responses of organisms to environmental changes, including climate fluctuations and habitat alterations. Key considerations include:
- Microbial Ecology: Through transcriptomics, researchers can analyze microbial communities in various habitats. This enables the assessment of biodiversity and the roles of different organisms in ecological processes. For example, RNA-seq can reveal how specific microorganisms respond to pollutants or nutrient variations in soil or water.
- Evolutionary Biology: By studying the RNA profiles of different species, scientists can gain insights into evolutionary adaptations. Such information can be critical in understanding how species might adapt to changing ecosystems.
- Conservation Efforts: RNA sequencing can aid in identifying endangered species and understanding their genetic diversity. This can inform conservation strategies by highlighting which species are more resilient to environmental changes.
In summary, the applications of RNA sequencing are essential in both biomedical research and environmental studies, offering pathways for innovation and discovery. Evolving methodologies continue to expand its utility, ensuring this technique remains at the forefront of genetic and ecological research.
Future Trends in RNA Sequencing
As we look ahead in the realm of RNA sequencing, it is crucial to understand not only the innovations on the horizon but also the profound implications these developments may carry. The future of this field goes beyond mere technical upgrades; it signifies a shift towards more versatile, efficient, and insightful approaches to biological research. This section delves into emerging technologies and potential impacts on research and medicine, emphasizing their relevance in shaping the landscape of genomic studies.
Emerging Technologies and Innovations
The pace of advancement in RNA sequencing technology is nothing short of remarkable. With every passing year, newer methodologies are emerging, fundamentally altering how researchers conduct their work. Key innovations currently capturing attention include:
- Single-Cell RNA Sequencing: As biologists increasingly focus on cellular heterogeneity, single-cell RNA sequencing enables the analysis of individual cells. This method reveals insights that bulk sequencing simply cannot provide, such as distinct cellular states and activities within heterogeneous populations.
- Spatial Transcriptomics: This cutting-edge technology combines RNA sequencing with histological information, allowing researchers to explore how the spatial arrangement of cells influences gene expression. Itβs a leap forward in understanding the complex interaction between cells within tissues, shedding light on developmental biology and disease mechanisms.
- RNA Sequencing on Portable Devices: Miniaturization and portability are making RNA sequencing more accessible. Tools that can sequence RNA on-siteβsuch as device innovations resembling handheld gadgetsβcould democratize genomic studies in remote locations, leading to a broader applicability in ecological studies and personalized medicine.
"The drive for portable sequencing solutions could lead to real-time disease diagnostics in field settings, where traditional approaches fall short."
With the emergence of these technologies, the horizon looks promising for RNA sequencing. Researchers are poised to tackle questions previously deemed too complex or large-scale.
Potential Impacts on Research and Medicine
The advancements in RNA sequencing are set to revolutionize fields ranging from basic biology to clinical applications. Here are some noteworthy areas where RNA sequencing may yield significant impacts:
- Personalized Medicine: By analyzing patient-specific RNA profiles, healthcare providers can tailor treatments to individuals, matching therapies with the underlying molecular mechanisms of diseases. This level of customization may enhance the efficacy of treatments and reduce adverse effects.
- Disease Mechanisms: RNA sequencing will likely continue to illuminate the intricate pathways involved in various diseases, including cancer and neurodegenerative disorders. Understanding these pathways aids in developing targeted interventions that address the root causes rather than just the symptoms.
- Ecosystem Monitoring: As environmental concerns grow, RNA sequencing can help monitor biodiversity and ecosystem health. By studying the RNA of various organisms, scientists can track species changes and ecological responses to stressors like pollution or climate change.
- Synthetic Biology: As techniques in RNA manipulation advance, synthetic biology stands to gain tremendously. Innovations in RNA design could allow for the creation of bespoke RNA molecules, potentially leading to breakthroughs in agricultural science and food security.
As we move forward, the implications of these trends are vast. They promise not just progress in methodologies but a renaissance in our understanding of biology and medicine as a whole.
Closure
The conclusion serves as a pivotal component in solidifying the core themes presented throughout this article. It encapsulates the multitude of insights and nuances surrounding RNA sequencing library preparation, enhancing the readerβs comprehension of its significance in genomic research. With each facet carefully dissected, the conclusion allows for a cohesive synthesis of information, emphasizing the benefits and considerations inherent in this field.
Summary of Key Insights
In reviewing the journey from the foundational concepts of RNA sequencing to the advanced methodologies that have emerged, several key insights stand out:
- Importance of Precision: The meticulous nature of library preparation is crucial to ensure accurate representation of RNA populations, directly influencing the outcomes of genomic analyses.
- Technological Advancements: Innovations in sequencing technologies, such as Illumina and PacBio, have significantly enhanced throughput and accuracy, making previously inaccessible analyses feasible.
- Quality Control Protocols: Rigorous quality assessments are indispensable, as they safeguard the integrity of data, ultimately impacting biostatistical analyses and biological interpretations.
- Experimental Design Considerations: Thoughtful planning, including sample size and control conditions, is essential to derive statistically significant insights from RNA sequencing experiments.
Each of these elements weaves together to create a tapestry of understanding that reveals how deeply integrated RNA sequencing is within the future of biological research.
The Evolution of RNA Sequencing's Role in Science
Tracing back to its inception, RNA sequencing's role in science has undergone a remarkable transformation. Initially viewed as a niche tool for specialized research, it has burgeoned into a cornerstone for many fields. Here are a few notable landmarks in its evolution:
- From Microarrays to Sequencing: The transition from microarray technology to high-throughput sequencing revolutionized transcriptomics. This shift allowed scientists to move beyond predefined gene sets, enabling a more comprehensive analysis of the transcriptome.
- Integration with Genomic Analysis: RNA sequencing enables a more detailed understanding of gene expression regulation and alternative splicing. The synergy between RNA sequencing and genomic technologies has ushered in new avenues of research, fostering breakthroughs in areas like cancer genomics and developmental biology.
- Rise of Single-Cell Sequencing: Recent advancements have pioneered the field of single-cell RNA sequencing, allowing researchers to uncover cellular heterogeneity within tissues. This approach holds promise in comprehending complex diseases and tailoring personalized medicine.
- Data-Big and Bioinformatics: The exponential growth in the datasets generated through RNA sequencing has led to an increased emphasis on bioinformatics. The evolution of computational tools reflects the necessity for sophisticated data analysis techniques, making the role of bioinformaticians more critical than ever.