Pharmacokinetics in Clinical Trials: Key Insights
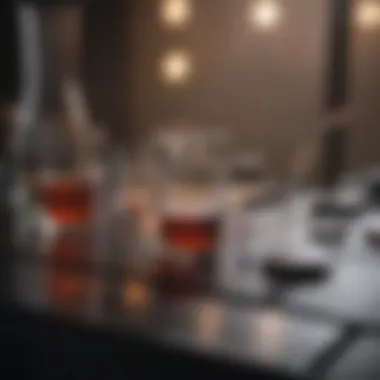
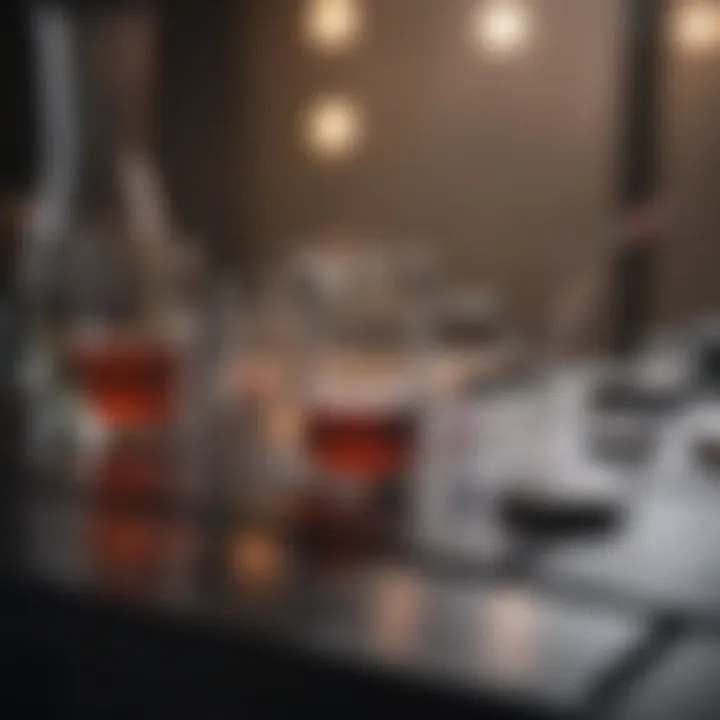
Intro
Pharmacokinetics (PK) is a cornerstone of clinical trials, providing critical insights into how drugs are absorbed, distributed, metabolized, and excreted in the human body. These processes are not merely academic; they carry immense implications for drug efficacy, safety, and overall patient health outcomes. In the rapidly evolving landscape of medicine, understanding PK can help researchers navigate the labyrinthine complexities of drug development. This article aims to shed light on the methodologies employed in PK studies and the downstream effects these studies have on clinical practice and therapeutic advancements.
Throughout this discussion, readers will encounter an array of topics, including the importance of carefully designed PK studies, the analytical techniques used to derive meaningful data, and the regulatory landscape that guides these efforts. By dissecting these elements, the narrative offers a detailed, nuanced understanding tailored for students, researchers, educators, and professionals alike.
As we embark on this exploration, it's essential to recognize the multifaceted role of PK in shaping the future of medicine while also addressing the intricacies that come with it. This understanding provides a solid foundation from which we can appreciate the importance of PK in real-world clinical settings.
Prologue to Pharmacokinetics
Pharmacokinetics (PK) plays a vital role in the development and application of pharmaceutical therapies. Essentially, it studies how drugs move through the body, detailing processes like absorption, distribution, metabolism, and excretion. By understanding these dynamics, researchers can predict the drug's behavior in real-world scenarios, tailoring therapies to maximize efficacy and minimize adverse effects.
Importance of Studying PK The significance of pharmacokinetics lies in its ability to inform critical decisions in drug development and clinical management. PK data not only helps in determining optimal dosing regimens but also sheds light on drug interactions and individual variances in response. Therefore, a solid grasp of pharmacokinetic principles is invaluable for professionals involved in clinical trials, ensuring that patient safety and therapeutic effectiveness remain paramount.
Definition and Importance of PK
Pharmacokinetics refers to the quantitative assessment of the biological fate of a drug after its administration, tracking how it's absorbed into the bloodstream, distributed across various tissues, metabolized by enzymes, and ultimately excreted from the body. This science is crucial because it helps in understanding the duration and intensity of a drug's effect, which directly correlates to its therapeutic potential.
"Understanding pharmacokinetics is not just academic; it's about real-world impact on patient care."
Without robust PK data, clinical trials would run the risk of yielding ineffective or even harmful outcomes. Evaluating factors like half-life, bioavailability, and drug concentration at various time points provides a clear picture of how a drug behaves within different demographic populations. It’s a critical aspect when evaluating the safety and efficacy of new medicines, often guiding trial designs and regulatory approvals.
Historical Context
The study of pharmacokinetics has evolved over decades, rooted deeply in the fields of chemistry, biology, and medicine. Originally, pharmacokinetics was a simple affair; early drug studies focused predominantly on the effects of drugs without much understanding of their kinetics. However, as technology advanced and scientific methodologies improved, the need for a more structured approach became evident.
In the mid-20th century, foundational work laid out the principles of pharmacokinetics, drawing from earlier pharmacological studies. Researchers began establishing mathematical models to predict drug concentrations over time based on animal studies, providing the groundwork for modern PK. Today, thanks to advancements in analytical techniques, it's possible to measure drug levels in various biological matrices with staggering precision. This evolution underscores the increasing complexity and importance of understanding PK when developing new therapies and conducting clinical trials.
Epilogue
In essence, pharmacokinetics is not merely a specialized field; it’s a cornerstone of clinical research that determines the trajectory of drug safety and effectiveness. The historical progression of PK methodologies highlights its growing importance in therapeutic advancements, an evolution that continues to shape clinical trials today.
Components of Pharmacokinetics
Understanding the components of pharmacokinetics (PK) is crucial for grasping how drugs behave in the body. These components lay the groundwork for insightful clinical trial methodologies and enable researchers to predict how a drug will perform in various populations. Delving into each component helps in tailoring drug development strategies and optimizing treatment efficacy. Here, we will explore four key components: absorption, distribution, metabolism, and excretion. Each one plays a vital role in shaping a drug’s journey through the body.
Absorption Mechanisms
Absorption refers to the process by which a drug enters the bloodstream. This initial phase is critical for determining the bioavailability of a drug, which is the fraction that reaches systemic circulation. Various factors influence absorption, including the drug’s formulation, route of administration, and the gastrointestinal environment.
- Route of Administration: Different routes—oral, intravenous, intramuscular, and subcutaneous—significantly affect how quickly and efficiently a drug is absorbed. For instance, intravenous administration offers immediate access to circulation.
- Physicochemical Properties: The solubility and permeability of a drug are closely tied to chemical properties. Lipophilic drugs tend to passively diffuse across cellular membranes, while hydrophilic drugs may require transport proteins.
- Gastrointestinal Factors: pH, presence of food, and gut motility can all influence absorption rates. For example, a fatty meal may enhance the absorption of certain lipophilic medications by increasing bile production.
Distribution Parameters
Once a drug is absorbed, the next stage is distribution—spreading throughout the body via the bloodstream. Several parameters are essential in understanding how drugs distribute and their eventual action.
- Volume of Distribution (Vd): This parameter indicates how extensively a drug disperses into body tissues. A large Vd suggests a drug is widely distributed, possibly accumulating in tissues rather than remaining in circulation.
- Protein Binding: Drugs often bind to proteins like albumin in the bloodstream, which can either enhance or hinder their efficacy. Drugs that are highly protein-bound may be less bioavailable, since only the unbound fraction is active.
- Tissue Permeability: The ability of a drug to penetrate tissues and cells can be influenced by blood flow and specific characteristics such as lipid solubility and size.
Metabolism Pathways
Metabolism, or biotransformation, is the process by which the body chemically modifies a drug. This process typically occurs in the liver, where enzymatic action transforms a substance into compounds that are easier for the body to excrete.
- Phase I Reactions: These reactions usually involve oxidation, reduction, or hydrolysis and serve to introduce or expose functional groups on the drug molecule, often making it less active.
- Phase II Reactions: In this stage, conjugation reactions occur, where the drug or its metabolites are linked to another substance to form a more soluble compound, aiding in excretion.
- Enzyme Variability: Individual differences in enzyme activity due to genetic factors can result in variability in drug metabolism, leading to different therapeutic outcomes in similar populations.
Excretion Processes
Excretion is the final stage of the pharmacokinetic journey, referring to the removal of drugs and their metabolites from the body. The kidneys are the primary organs for drug excretion, but other routes include bile, saliva, sweat, and breast milk.
- Renal Excretion: The kidneys filter the blood, removing waste products. The rate of drug excretion can change based on kidney function, hydration status, and other health conditions.
- Biliary Excretion: Some drugs are secreted into bile and subsequently excreted in the feces. This pathway is particularly relevant for large, hydrophobic compounds.
- Factors Influencing Excretion: Urinary pH, age, and concurrent medications can all affect how quickly and efficiently a drug is excreted from the body.
"A thorough understanding of each component of pharmacokinetics can illuminate how drugs are processed and inform best practices within clinical trials."
In summary, each element of pharmacokinetics—absorption, distribution, metabolism, and excretion—plays a significant role in shaping the effectiveness of drugs in the clinical setting. Recognizing the importance of these components allows for better study designs and ultimately enhances drug safety and efficacy.
Designing a Pharmacokinetics Study
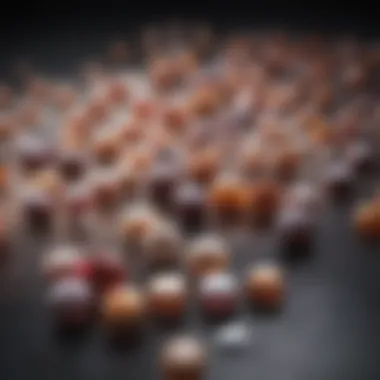
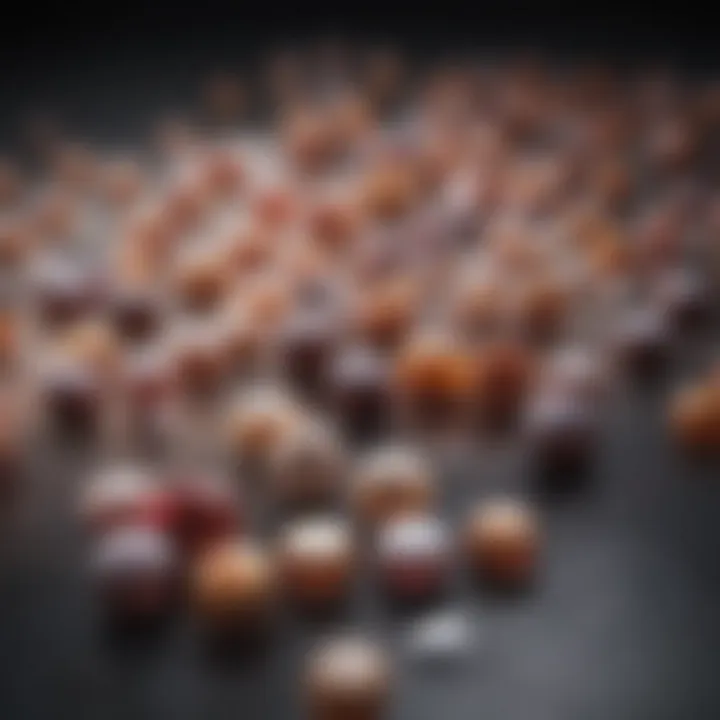
Creating a pharmacokinetics (PK) study is no walk in the park. It calls for a careful balance of precise objectives, well-defined endpoints, and the right mix of participants. These elements are crucial, not just for gathering data, but for ensuring that the findings can reliably inform drug development and patient care.
Objectives and Endpoints
Establishing objectives in a PK study is akin to setting a destination before embarking on a journey. They guide every phase, from designing the study to interpreting the results. Objectives could range from understanding how quickly a drug is absorbed to its distribution in various tissues. In some cases, these goals might be broader, like assessing the drug's impact on a particular disease state across different populations.
Endpoints, on the other hand, are the milestones that signal progress toward these objectives. They can be measured outcomes, like peak plasma concentrations (Cmax) or the area under the concentration-time curve (AUC). Making these endpoints clear and measurable is essential. It allows researchers to assess whether their questions can be answered. Effective endpoints not only enhance the study's reliability but also improve regulatory acceptance when it comes to documentation and reporting.
Sample Size Determination
Sample size determination is a critical step in study design. Too few subjects can lead to an inconclusive study, while too many can waste resources and raise ethical concerns. Here, statistical power comes into play. A power analysis helps in estimating the number of subjects needed to reasonably detect expected effects and ensure that variability in drug response is adequately captured.
Researchers often consider population variability, including factors like age, weight, and health status. For instance, when studying a drug intended for the elderly, it may be wise to include a corresponding number of younger volunteers to compare how age influences PK behaviors.
"Determining the proper sample size is not just a numbers game; it’s essential for the validity of the entire research."
Choosing Study Population
Choosing the right study population is another vital cog in the wheel of pharmacokinetics study design. Researchers need to select a cohort that reflects the intended patient demographic for the drug being studied. For example, if the drug is aimed at curing a disease prevalent mostly among older adults, then it would make sense to focus on that age group rather than including a mix of ages blindly.
Additionally, special considerations may be required for subpopulations such as pediatric patients, pregnant women, or those with comorbidities. Compliance, responses, and overall health can differ significantly across different groups. Hence, careful selection not only leads to more applicable results but also impacts drug safety evaluations.
In short, designing a PK study is more than just an exercise in logistics and planning. It’s a foundational phase that shapes the very outcomes of drug development. By keeping a keen eye on objectives, sample sizes, and study populations, researchers lay the groundwork for impactful insights that can drive the future of healthcare.
Analytical Techniques in PK Studies
In the realm of pharmacokinetics, analytical techniques serve as the backbone of any well-designed study. They are indispensable in ensuring the reliability and accuracy of data that ultimately informs drug development. Without robust analytical methods, one might as well be navigating unchartered waters without a compass. The intricacies involved in measuring drug concentrations, their metabolites, and the corresponding pharmacological effects cannot be overstated. Such measurements lay the groundwork for understanding how a drug moves through the body, highlighting the interdependence of absorption, distribution, metabolism, and excretion (ADME).
The selection of analytical techniques encompasses a multitude of considerations. Factors like the nature of the drug, the biological matrix in question, and the desired analytical sensitivity play a crucial role. For instance, a potent drug may highlighly require sensitive bioanalytical methods that can detect miniscule amounts in biological samples, while compounds with a broader therapeutic range could potentially use less sophisticated approaches. Every aspect must be measured against cost-effectiveness, validation requirements, and the intended study design.
Bioanalytical Methods
Bioanalytical methods are a central element of pharmacokinetic studies. They refer to techniques used to measure concentrations of drugs and their metabolites in biological samples such as blood, urine, and tissues. Common techniques include liquid chromatography coupled with tandem mass spectrometry (LC-MS/MS) and enzyme-linked immunosorbent assays (ELISA). These methods stand out due to their sensitivity and specificity. For example, the LC-MS/MS technique can allow for the detection of low nanomolar concentrations, making it particularly useful for drugs with extensive metabolism where metabolites may exist in trace amounts.
Among bioanalytical methods, it's imperative to consider sample preparation, which can significantly influence the quality of the analytical results. If the samples are not prepared correctly, even the most advanced bioanalytical equipment can yield false readings. Common challenges in sample preparation include protein precipitation, solid-phase extraction, and liquid-liquid extraction. Each method comes with its own set of pros and cons, so selecting the one that fits the study's goal is crucial.
Data Collection Tools
Data collection tools in pharmacokinetics studies go beyond mere spreadsheets and notebooks. They are vital for capturing the intricacies of biological responses to drug administration. Both electronic data capture systems and clinical trial management software have become essential components in modern PK studies. Not only do they streamline data entry, but they also improve the reliability and accessibility of large datasets.
For instance, electronic laboratory notebooks (ELNs) allow researchers to document procedures, observations, and results in real-time, which ultimately enhances the reproducibility of the study. On the other hand, clinical trial management systems (CTMS) help manage subjects, monitor progress, and ensure compliance with regulatory standards. Having the right tools in place significantly reduces variability in data collection, thus improving the validity of the results.
Statistical Analysis
Statistical analysis in pharmacokinetics plays a pivotal role in interpreting the data collected from bioanalytical methods and data collection tools. It goes beyond wearing a lab coat and crunching numbers; it requires a thoughtful approach to understanding variability and uncertainty within the data. Employing the proper statistical techniques enables researchers to draw meaningful conclusions about drug behavior in diverse populations.
Statistical methods such as non-compartmental analysis (NCA) and compartmental modeling are often utilized to characterize pharmacokinetic parameters such as clearance, volume of distribution, and half-life. While NCA is simpler and easier to implement, compartmental modeling allows for a more detailed understanding of drug dynamics and kinetics. The choice between these methods often hinges on the specific objectives of the study, available data, and the level of analytical complexity that can be managed. The right statistical analysis can untangle the narrative that the data tells, shape hypotheses, and ultimately guide future research.
In summary, the analytical techniques used in pharmacokinetics studies are critical. A thoughtful selection can elevate the quality of research and significantly impact drug development outcomes.
The synergy of bioanalytical methods, data collection tools, and robust statistical analysis paves the way for reliable pharmacokinetics studies. When these components coalesce, they provide invaluable insights into how drugs behave in the body, shaping the trajectory of clinical research and therapeutic innovation.
Regulatory Aspects of PK Studies
The landscape of pharmacokinetics (PK) studies in clinical trials is heavily shaped by regulatory frameworks. Understanding these regulations is vital for ensuring that PK studies are designed, executed, and reported effectively. Regulatory aspects not only validate the findings but also provide a safeguard for patient safety, ensuring that new drugs undergo rigorous testing before reaching the market. For professionals involved in drug development, the significance of these guidelines cannot be overstated, as they establish the benchmark for data credibility and reproducibility.
Notably, these regulations serve multiple purposes. They establish standards for study protocols, which help to mitigate uncertainty regarding the effectiveness and safety of medications. Compliance with these regulatory guidelines is not an option; it is an essential part of the clinical trial process that impacts timelines and development costs. The implications of adhering—or failing to adhere—to these guidelines can be both costly and time-consuming, leading to delays in drug availability and increased scrutiny from health agencies.
"Regulatory frameworks provide the structure within which the scientific data is interpreted, ensuring that its applications are ethical and safe."
Guidelines from Regulatory Agencies
Regulatory agencies such as the U.S. Food and Drug Administration (FDA) and the European Medicines Agency (EMA) offer a robust set of guidelines surrounding PK studies. One primary document, the FDA's Guidance for Industry: Pharmacokinetics in Drug Development, outlines critical considerations such as:
- Study Design: Clear expectations for how to approach the design of PK studies, including phase considerations and the types of data needed.
- Data Collection: specifications for the methods of collecting pharmacokinetic data, highlighting required parameters and analytical approaches.
- Statistical Methods: outlines recommended statistical methods for interpreting the data, which helps ensure consistency and reliability in results.
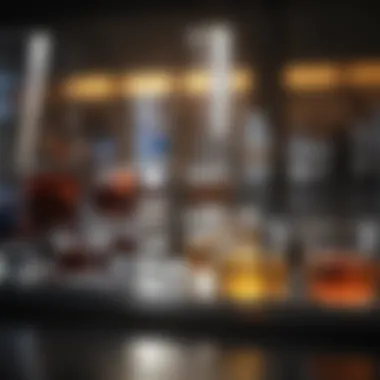
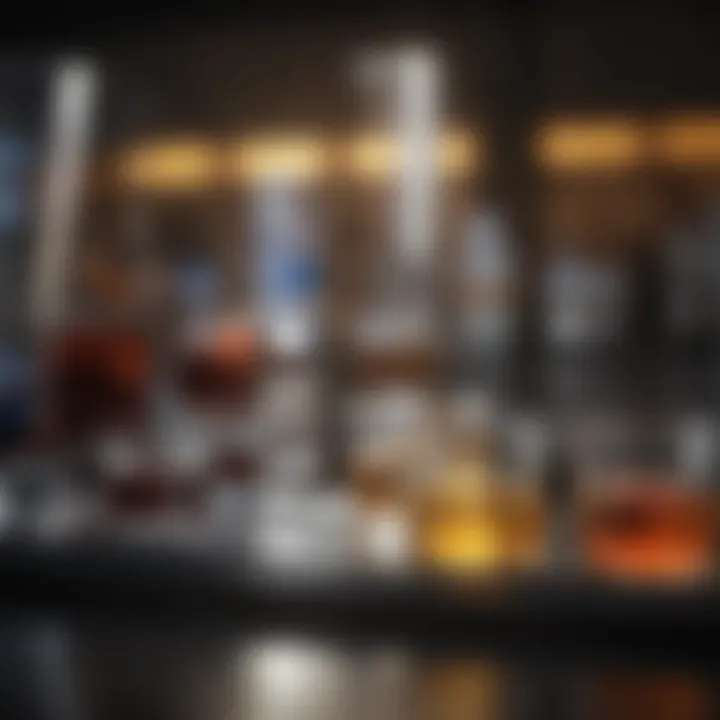
In addition to these specific guidelines, agencies emphasize the need for transparency and thorough documentation during the clinical trial process. This includes the need for a pre-specified statistical analysis plan and proper handling of missing data, all meant to fortify the integrity of the study outcomes.
Ethical Considerations
Ethics in PK studies revolve around safeguarding participants' well-being while providing reliable data for drug efficacy and safety. Issues of informed consent, risk assessment, and the management of vulnerable populations are crucial components.
Firstly, obtaining informed consent is vital. Participants must be fully aware of what the trial involves, the potential risks, and the benefits of taking part. This not only fosters trust between researchers and participants but also aligns with ethical research principles that prioritize participant autonomy.
Moreover, the ethical implications of PK studies extend to the design of the trials itself:
- Risk-Benefit Ratio: Careful consideration must be given to the potential benefits of the drug against possible risks to the participants.
- Inclusion Criteria: Inclusion and exclusion criteria should be ethically sound, especially concerning vulnerable populations, such as children, the elderly, or pregnant women.
Ultimately, fostering an ethical research environment not only complies with regulatory requirements but also enhances the quality of the research. The dual challenge of meeting regulatory expectations while respecting ethical standards becomes an exercise in balance that every researcher must navigate.
Pharmacokinetics in Special Populations
Pharmacokinetics affects drug efficacy and safety across diverse patient groups. In certain populations, like children, pregnant women, and elderly individuals, the body processes drugs differently. Each group presents unique characteristics that influence drug absorption, distribution, metabolism, and excretion. This complexity emphasizes the need for tailored pharmacokinetic studies. Special populations are not merely subsets but require thoughtful consideration to ensure optimal therapeutic outcomes.
Pediatrics
When considering pharmacokinetics in pediatrics, it's critical to understand that children are not just small adults. Physiological differences—such as organ maturation and body composition—lead to distinct pharmacokinetic profiles. For example, infants have higher total body water percentages compared to adults, which can affect drug distribution. Furthermore, enzyme activity related to drug metabolism can vary significantly with age. In particular, phase I and II metabolic pathways may not be fully developed at birth, impacting drug clearance rates.
A practical implication is that dosage recommendations for children often rely on weight-based criteria. However, this approach might not always ensure safety or efficacy because it doesn't account for these nuanced pharmacokinetic differences. An example of this is the use of paracetamol, which must be carefully dosed to avoid toxicity as children grow.
Pregnant and Lactating Women
Pregnancy introduces a cascade of physiological changes that can significantly modify drug pharmacokinetics. Blood volume and plasma expansion increase, altering drug distribution volumes. Moreover, placental transfer of medications adds another layer of complexity, as drugs can affect fetal development. The increased activity of certain drug-metabolizing enzymes can lead to changes in clearance rates of medications.
For instance, the use of antidepressants during pregnancy has raised concerns about potential risks to the fetus while still being necessary for the mother's mental health. Such situations demand a careful balancing act—between treating the mother effectively and safeguarding fetal health.
Lactation also requires attention to drug pharmacokinetics. While many drugs are excreted in breast milk, the concentration and impact vary significantly, emphasizing the importance of choosing appropriate medications during breastfeeding to minimize risks to the infant.
Elderly Patients
In elderly patients, pharmacokinetics is influenced by biological aging processes, which can alter the pharmacodynamics of medications. Key factors include reduced organ function, changes in body composition, and polypharmacy, where older adults often take multiple medications. In particular, renal function naturally declines with age, which raises concerns for drugs that rely heavily on renal clearance. This necessitates careful evaluation of dosing adjustments to avoid adverse reactions.
Additionally, changes in hepatic metabolism can affect drug half-lives and potentially lead to accumulation of certain medications. For example, medications like warfarin require vigilant monitoring due to variability in response among older adults. This high variability underscores the importance of personalized treatment plans in achieving safe and effective outcomes in this population.
The pharmacokinetic profiles of special populations underscore the importance of more nuanced, population-specific research to optimize therapeutic regimens.
"Understanding pharmacokinetics in special populations is not just an academic exercise; it’s critical to enhancing the efficacy and safety of prescribed medications in these vulnerable groups."
In summary, special populations present specific challenges in pharmacokinetics that demand targeted research and practical solutions. Tailoring drug development and therapeutic strategies based on these group differences not only enhances patient care but also contributes to broader public health objectives.
Translational Pharmacokinetics
Translational pharmacokinetics plays a pivotal role in bridging the gap between preclinical research and clinical application. Its significance lies in its ability to translate findings from animal studies to human models, ensuring that the theoretical concepts developed in the lab can be effectively applied in real-world medical practice. In the realm of drug development, this translation is not merely a checkmark on a list but a comprehensive endeavor that encapsulates understanding the complex interplay of drug absorption, distribution, metabolism, and excretion in humans.
Moreover, translational pharmacokinetics helps in predicting human responses to novel drug candidates, as well as understanding variations among different populations. This aspect is inherently linked to the ultimate aim of pharmacokinetics—to maximize efficacy while minimizing adverse effects in therapeutic interventions. By utilizing data drawn from animal models thoughtfully, researchers can define which parameters are relevant for their human studies, thus improving the predictability of outcomes.
From Animal Models to Humans
The transition from animal models to human subjects in pharmacokinetics is not a simple one; it is often fraught with challenges. Animal models, whether they are rodents like mice and rats or larger species such as pigs and dogs, provide a foundational base for understanding drug behavior. However, several factors differ substantively between these models and human physiology. For instance, metabolic pathways may significantly vary, leading to different drug clearance rates or adverse reactions.
It’s crucial to conduct thorough investigations into the pharmacokinetic parameters observed in animals. This includes assessing factors such as:
- Dosage selection: Determining appropriate dosages that reflect human use.
- Route of administration: Understanding how different routes may impact drug bioavailability.
- Physiological differences: Recognizing how species variations in organ function can influence drug metabolism.
While there is no one-size-fits-all approach, the knowledge gathered from carefully controlled animal studies can guide the design of early-phase human trials. This aspect not only enhances the understanding of potential risks and benefits but also aligns the trial designs more closely with eventual therapeutic applications.
Identifying Biomarkers
Identifying biomarkers is an integral part of translational pharmacokinetics, serving as a bridge that can link pharmacokinetic predictions to observable clinical outcomes. Biomarkers can be specific biological indicators that reveal information about how a drug behaves in a body or how it interacts with various biological systems. They can encompass everything from genetic expressions to metabolite levels and are essential for tailoring therapies to individual needs.
The identification process often utilizes cutting-edge technologies such as:
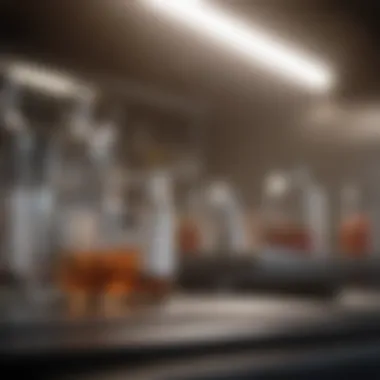
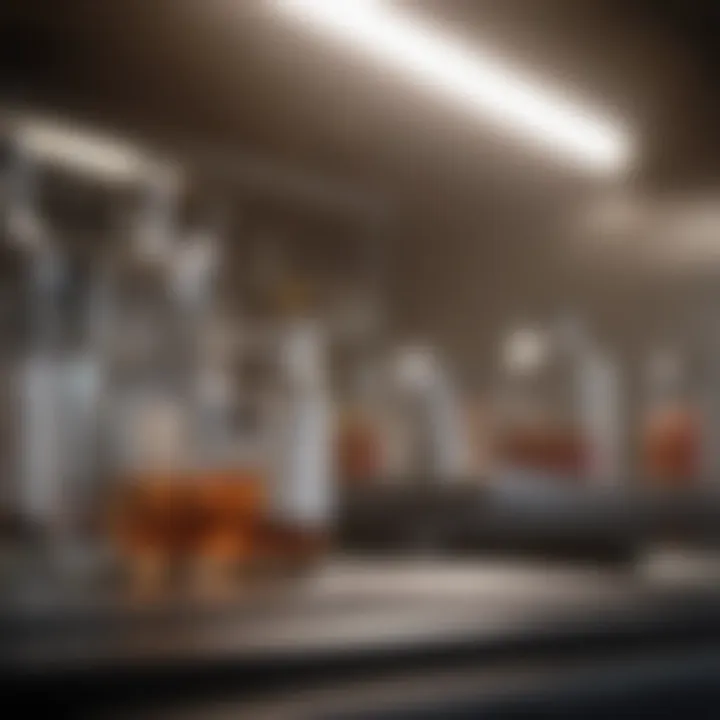
- Genomic profiling: Provides insight into variations in drug metabolism across different genetic backgrounds.
- Metabolomics: Analyzes metabolites produced as a result of drug action, providing a snapshot of pharmacokinetic responses.
- Proteomics: Examines changes in protein expression profiles in response to treatment, offering additional layers of understanding.
With these practices, biomarkers can aid in predicting efficacy, optimizing dosing regimens, and identifying subsets of patients who may respond better to certain treatments. In this context, the ability to forecast how specific populations might metabolize drugs before they hit the market can enhance drug safety and efficacy, thereby streamlining the development process.
"The right biomarker can turn an educated guess into a well-informed decision, enhancing success rates in clinical development."
Challenges in Pharmacokinetics Studies
The field of pharmacokinetics is crucial for shaping how drugs interact within human physiology. However, various challenges arise during this process that can significantly affect study outcomes. Recognizing and addressing these challenges is vital for the reliability of PK studies and the eventual success of drug development. One major aspect is ensuring that these studies represent real-world scenarios. This isn’t about just running a few tests; it requires a deep understanding of human biology and the various factors that can skew results.
In this section, we delve into two primary hurdles faced in pharmacokinetics studies, highlighting their implications and how they can influence the findings.
Variability in Responses
Individual responses to medications can differ widely, and this variability is often cited as a significant challenge in pharmacokinetics studies. Factors like age, weight, genetic makeup, and even lifestyle choices play a role in how a drug is absorbed, distributed, metabolized, and ultimately excreted.
- Genetic Factors: Variation in genetic makeup can lead to different expressions of metabolic enzymes. For instance, certain individuals might metabolize a drug more quickly or slowly than others, leading to variations in efficacy and toxicity.
- Physiological Differences: The physiological state, including organ function like liver and kidney health, can also produce varying responses. A healthy adult might process a medication differently than someone with liver disease.
- External Factors: Medications can interact with food, other drugs, or even herbal supplements, creating further variability. For example, the presence of grapefruit juice can inhibit certain enzymes, significantly affecting drug metabolism.
This variability complicates sample size determination and the selection of study endpoints. It also makes effective dosing regimens a moving target, challenging researchers to find a one-size-fits-all approach.
"Understanding variability in PK studies is not just about recognizing differences but about embracing a more personalized approach to medicine."
Influence of Drug Interactions
Drug interactions pose another cloud in the pharmacokinetics landscape. These interactions can lead to a reduction or enhancement of a drug's effect, making it tricky to predict clinical outcomes. There are several types of drug interactions to be aware of:
- Pharmacokinetic Interactions: These occur when one drug affects the absorption, distribution, metabolism, or excretion of another. For instance, a drug that induces liver enzymes may increase the metabolism of concurrently administered drugs, potentially rendering them ineffective.
- Pharmacodynamic Interactions: These involve the combined effects of drugs on the same physiological pathway. For example, taking two blood thinners together might increase risks of bleeding.
- Timing of Administration: The way drugs are taken can also influence interactions. Timing can affect absorption rates, leading to altered pharmacokinetic properties.
Research must carefully monitor these interactions. They can cause previously established dosing guidelines to become obsolete, complicating the approval process and patient safety considerations. Hence, awareness and planning are essential as researchers design studies to account for these interactions.
In summary, challenges like variability in responses and the influence of drug interactions present significant hurdles in pharmacokinetics studies. By understanding these difficulties, researchers can better strategize study designs, enhancing the reliability of PK data and its application in clinical settings.
Future Directions in Pharmacokinetics Research
The world of pharmacokinetics is evolving at a breakneck pace, prompting researchers to seek innovative methodologies that can enhance the understanding of drug behavior in the body. It's not merely about measuring how a drug is absorbed, distributed, metabolized, and excreted anymore. Researchers are now turning to more sophisticated approaches to refine drug development strategies and optimize therapeutic outcomes.
Innovative modeling approaches play a substantial role in mapping the complex PK landscape. These tools not only simulate drug kinetics but also allow for the integration of real-world data, which enriches the predictive capabilities of PK models. Simulating different scenarios can provide insights that raw data alone cannot offer, helping researchers to grasp the nuanced interactions between drugs and biological systems. The benefits this holds for personalized medicine are considerable.
As for genomic data integration, it’s an area that simply can’t be ignored. The fusion of pharmacogenomics with pharmacokinetics opens the door to understanding how genetic variability influences drug responses in various populations. This is particularly relevant in developing tailored therapies that meet the specific needs of individuals, reducing the trial-and-error aspect that can often plague drug administration.
Innovative Modeling Approaches
Modeling in pharmacokinetics is not just about creating equations; it’s a blend of science and art. Advanced approaches like physiologically-based pharmacokinetic (PBPK) modeling have garnered attention for their ability to simulate drug behavior across different physiological scenarios. Such models take into account individual differences, such as age, sex, weight, and even the presence of comorbidities.
Moreover, with the rise of machine learning and artificial intelligence, predictive modeling is becoming increasingly sophisticated. These techniques can analyze vast datasets and reveal patterns that traditional statistical methods might miss. Notably, they facilitate the development of adaptive clinical trials, where adjustments can be made in real-time based on incoming data, thereby optimizing the drug development process.
Integration with Genomic Data
Incorporating genomic data into pharmacokinetics research isn't a distant dream—it's a present reality that enriches our understanding of patient variability. Through genotyping, researchers can identify specific genetic markers that affect drug metabolism, allowing for more accurate dosing and reduced adverse effects. This approach stands to revolutionize the way we look at drug efficacy and safety.
Healthcare providers can leverage this information to manage therapies better, increasing the likelihood of patient adherence and treatment success. Moreover, genomic insights can guide researchers in selecting the right populations for clinical trials, ensuring more homogeneous groups that can lead to more robust conclusions.
For patients, this means receiving medications that are not only more effective but also more personalized, significantly improving therapeutic outcomes.
In summary, the future of pharmacokinetics is not just about traditional metrics. It's about harnessing advanced modeling techniques and genomic data to understand better how drugs behave in diverse patient populations. As we move forward, these methodological advancements will undoubtedly play a pivotal role in drug development, ensuring that efficacy, safety, and patient-centricity remain at the forefront of pharmaceutical research.
The End
The discussion surrounding pharmacokinetics (PK) in clinical trials cannot be overstated. This final section aims to encapsulate the vital themes explored within the article, emphasizing how pivotal PK studies are in the realm of drug development.
Summary of Key Insights
Through our journey, we’ve highlighted several essential points:
- PK plays a foundational role in defining drug dosage regimens. Understanding how a drug is absorbed, distributed, metabolized, and excreted helps establish effective strategies for the administration of therapies.
- Variability in responses among populations can significantly alter the anticipated outcomes of clinical trials. Factors such as age, gender, and genetic makeup must be accounted for to ensure the robustness of data.
- Regulatory guidelines serve as a backbone for ensuring safety and efficacy within PK research. Navigating these regulations is crucial for compliance and successful drug approval.
- Emerging technologies and innovative modeling approaches are reshaping the future landscape of pharmacokinetics. This includes more precise identification of biomarkers and better integration with genomic data, ultimately providing a deeper understanding of how drugs interact within the body.
Implications for Drug Development
The implications drawn from understanding pharmacokinetics are manifold. By prioritizing PK studies, developers can make informed decisions regarding drug formulations, leading to safer and more effective treatments. This includes:
- Personalized medicine: By recognizing the variability across populations, researchers have the opportunity to tailor drug therapies to individual needs, thus enhancing therapeutic outcomes.
- Reduction in adverse effects: Comprehensive PK analysis aids in identifying potential drug interactions, minimizing risks associated with polypharmacy.
- Regulatory compliance: A robust understanding of PK informs the design of clinical trials that meet regulatory standards, ultimately streamlining the drug approval process.
- Resource optimization: Focusing on PK early in the development process can prevent costly failures later, allowing for smarter allocation of financial and human resources.
As we look to the future, the integration of pharmacokinetic principles within clinical trials will continue to evolve. The insights gained from PK studies are integral not just for meeting regulatory expectations, but also for embracing the complexities of human physiology to drive breakthroughs in therapeutic development.