Innovations and Applications of Optical MEMS Technology
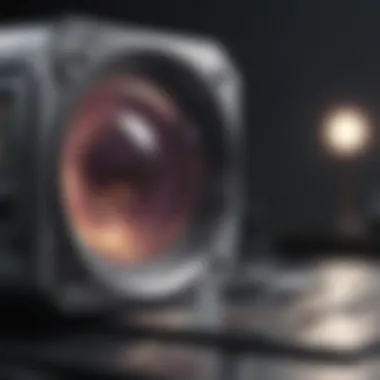
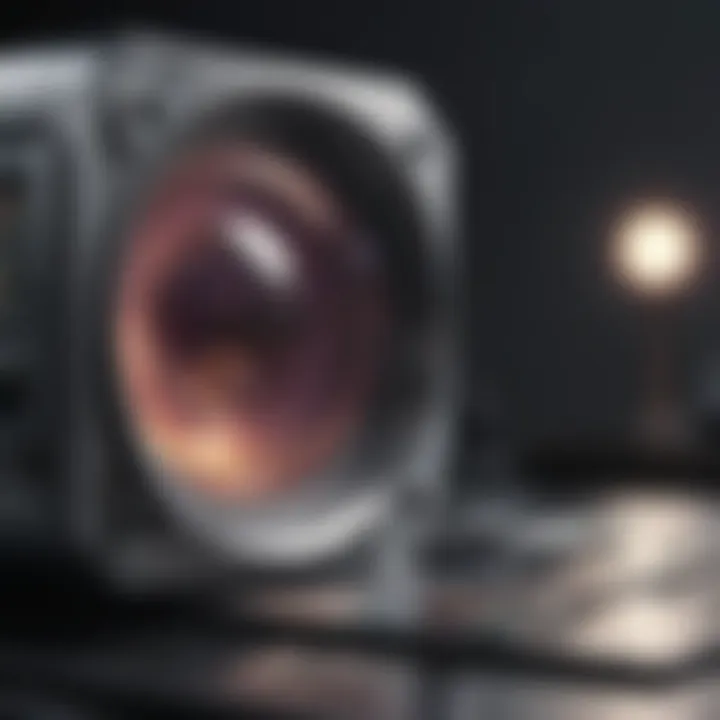
Intro
As technology marches forward, the intersections of optics and microsystems paint a fascinating landscape that captures a myriad of applications, from telecommunications to healthcare. Optical Micro-Electro-Mechanical Systems (MEMS), a prime example of this convergence, has rapidly evolved, breathing new life into various devices and systems that rely on manipulating light at microscopic scales. This article embarks on a detailed exploration into the world of Optical MEMS, illuminating key innovations, fabrications, and the far-reaching implications of these systems across diverse sectors.
In the realm of Optical MEMS, the fundamental principles operate at the thrilling juncture where light meets mechanics. It's about harnessing the tiny, yet powerful, mechanical structures that can control light in incredibly nuanced ways. These devices are not just a fly-by-night trend; they represent significant advancements that have the potential to transform technologies.
The journey into this realm uncovers a multitude of applications, like optical switches which have revolutionized data routing in telecommunications. These innovations not only enhance communication speeds but also offer efficient solutions that impact our everyday lives.
However, the road isn't without its hiccups. Challenges persist in terms of fabrication techniques, reliability, and cost efficiency. Thus, our discussion will also spotlight these hurdles, drawing a picture of the landscape that future researchers might navigate as they strive to propel Optical MEMS into their next chapter.
To provide a solid foundation, we will encapsulate key findings and methodologies used in the current research landscape. By synthesizing knowledge from various studies, the intent is to revolve around a coherent thesis about the significance of Optical MEMS that generates interest and understanding among students and professionals alike.
Preamble to Optical MEMS
Optical Micro-Electro-Mechanical Systems (MEMS) represent a fascinating intersection of optics and micro-technology, shaping countless innovations across various fields. By exploring Optical MEMS, we can appreciate how light manipulation at a microscale is becoming an essential component in communication, healthcare, and consumer electronics. These systems make the once-unthinkable, thinkable. The importance of Optical MEMS lies in their ability to enhance performance and efficiency while shrinking the size of devices, which is fundamental in today's fast-paced technological landscape.
Definition and Significance
Optical MEMS are devices that integrate optical elements with micro-machined components. This fusion allows for precise control and manipulation of light waves. In essence, they use tiny mirrors and lenses that can be adjusted with remarkable precision to direct and modulate light. The significance of Optical MEMS can be summed up in a few key points:
- Enhancing Communication: Optical MEMS are crucial in telecommunications, where they facilitate high-speed data transfer.
- Miniaturization: The ability to create smaller and more efficient components opens doors for innovative applications, especially in consumer electronics.
- Cost Reduction: These systems can lower production costs, making advanced technologies accessible to a broader audience.
All these factors illustrate why Optical MEMS aren't just a niche area of study but a fundamental part of modern technology that continually pushes the envelope.
Historical Development
The journey of Optical MEMS is quite the tale of innovation and adaptation. The initial concept of combining optical elements and micro-electromechanical systems dates back to the late 20th century. Early experiments laid the groundwork for what would become a booming field today. By the mid-1990s, researchers and manufacturers were actively exploring new applications. For instance, early devices for optical switches began to surface, establishing foundational work that would lead to modern applications.
The rapid advancement of fabrication techniques has allowed researchers to pivot towards more complex functionalities over the years. In the early 2000s, the introduction of micro-mirrors and adaptive optics propelled further development, refining the technology. Significant milestones include:
- The launch of digital micromirror devices by Texas Instruments, revolutionizing projection technologies.
- The development of micro-optical components that serve critical roles in laser and sensor technologies.
As we trace this evolution, it becomes clear that Optical MEMS reflect not only technical progress but also the growing demand for efficiency and innovation in a tech-savvy world.
Fundamentals of Optical MEMS
Optical MEMS play a critical role as the backbone of various modern optical technologies. It's essential to understand the fundamentals to appreciate their impact. This section dives into the core principles and materials involved in Optical MEMS, emphasizing what makes them unique and beneficial in today’s fast-paced technological landscape.
Basic Principles
Optical MEMS combine mechanical and optical components to create systems that can modulate light in sophisticated ways. The basic principle revolves around manipulating light pathways using tiny mechanical systems that can adjust, direct, or filter light. This interaction allows for precise control over optical signals, which is crucial in applications like telecommunications and imaging.
The fundamental mechanisms at play include the actuation of components through various means such as electrostatic forces or thermal expansion. For instance, when an electrical signal is applied, a micro-mirror might tilt, redirecting light that passes over it.
Additionally, the fine control of these mechanisms allows multi-dimensional modulation of light rather than just on or off states, broadening the spectrum of uses in high-speed communications or sensor technologies.
Components and Materials
Understanding the various components of Optical MEMS is crucial for grasping how these systems function. There are three primary components to discuss: micro-mirrors, light modulators, and micro-lenses.
Micro-mirrors
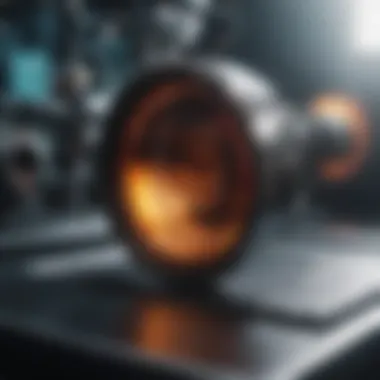
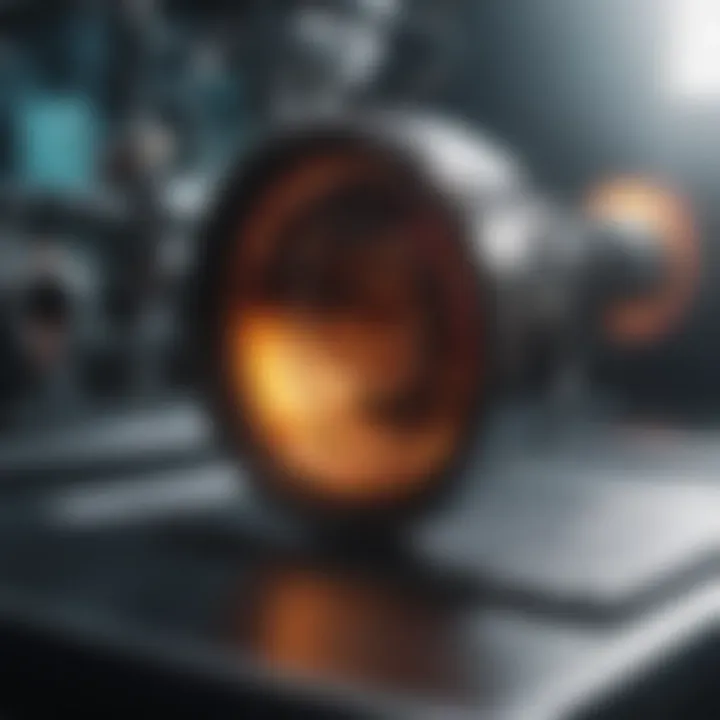
Micro-mirrors serve as the workhorses in many Optical MEMS applications. Their ability to tilt at varying angles makes them ideal for redirecting beams of light.
- Key Characteristic: Precision is the standout characteristic of micro-mirrors. They can be designed to position light with incredible accuracy, making them integral in applications like projectors and optical switches.
- Why Beneficial: Their small size and lightweight nature allow for compact designs while maintaining performance. This is particularly valuable in consumer electronics, where space is at a premium.
- Unique Feature: A significant aspect is their scalability; many micro-mirrors can be integrated into a single chip, enabling parallel processing of optical signals.
- Advantages/Disadvantages: While micro-mirrors can provide superior performance, they may also be susceptible to environmental factors such as humidity and temperature, which can affect their accuracy over time.
Light modulators
Light modulators alter the intensity or phase of light waves. They can essentially make light do the tango when controlled properly.
- Key Characteristic: Versatility stands out; they can implement a range of modulation types, from amplitude adjustments to phase shifts, which is essential for applications requiring dynamic signal processing.
- Why Beneficial: Light modulators are vital in telecommunications where varying signal conditions require adaptable systems; their ability to instantaneously adjust light pathways enhances system robustness.
- Unique Feature: Many types of modulators, like liquid crystal or electro-optic modulators, can achieve ultra-fast switching speeds, vital for high-data rate applications.
- Advantages/Disadvantages: However, these devices often necessitate complex drive electronics, potentially increasing the cost and design complexity of a system.
Micro-lenses
Micro-lenses play a pivotal part in enhancing optical system performance by focusing or spreading light effectively.
- Key Characteristic: Precision and functionality define micro-lenses. Their small size allows for high-density assembly, making them perfect for applications in camera systems and optical sensors.
- Why Beneficial: By improving light coupling efficiency, they can drastically increase the performance of systems in image capture and projection. Their ability to integrate into compact spaces marks them as highly beneficial in mobile devices.
- Unique Feature: Their customization potential is noteworthy; micro-lenses can be fabricated to specific geometries tailored for particular applications, enhancing their efficacy.
- Advantages/Disadvantages: On the flip side, micro-lenses can be vulnerable to dirt and scratches, issues that might affect their performance and durability if not carefully managed.
Overall, these components work together in harmony to form the foundation of Optical MEMS, contributing significantly to their applications and functionalities.
Manufacturing Techniques for Optical MEMS
The manufacturing of Optical Micro-Electro-Mechanical Systems (MEMS) entails a vast landscape of techniques that are crucial to the creation of precise and functional devices. This aspect is essential because it directly influences the performance, reliability, and cost-effectiveness of the optical MEMS, which are increasingly integral in various modern applications. By understanding these manufacturing techniques, professionals and students alike can appreciate the nuances that lie behind the operation and deployment of these sophisticated systems.
Lithography Processes
Lithography serves as a cornerstone technique in the MEMS fabrication process. This method is primarily utilized to transfer patterns onto the substrate, which forms the basis for creating micro-structures that define the optical functionalities of MEMS devices. Lithography processes can broadly be categorized into two types: mask-based and maskless lithography.
- Mask-based lithography employs a photomask that contains the circuit patterns. By exposing photoresist on the wafer through these masks, intricate designs can be produced, laying the groundwork for further processing steps.
- Maskless lithography, on the other hand, utilizes focused electron beams to directly write the pattern on the photoresist material. This method allows for flexibility and rapid prototyping but can be slower in producing high-volume products.
Each of these lithography techniques has its unique strengths and weaknesses. In particular, mask-based lithography has dominated the industry due to its efficiency and scalability, which are crucial for mass production.
Etching Methods
Once a pattern is defined on the substrate, the next step is to etch away the undesired material, creating the necessary structures. Etching methods can further be divided into wet etching and dry etching, both of which have distinct roles in the manufacturing of optical MEMS.
- Wet etching involves using liquid chemicals to remove layers from the surface of the substrate. This technique is often simpler and cost-effective but offers less precision compared to dry etching.
- Dry etching, particularly reactive ion etching (RIE), allows for greater control and a more refined etch profile, thus enabling the production of intricate geometries that are often required in optical applications.
These etching processes are vital since they define the precision of micro-components that ultimately determine the device’s performance. In high-tech applications where optical clarity and precision are paramount, the choice of etching method can make or break the final product.
Packaging and Integration
After the micro-structures are formed, the final step involves packaging and integrating the MEMS devices. This phase is critical and often involves various layers of complexity. Proper packaging ensures that the optical MEMS are protected from environmental factors like moisture and debris, which could significantly impair their performance.
In terms of integration, there are several considerations that need addressing:
- Optical alignment is essential to ensure proper functionality, especially in systems where multiple MEMS devices work together to perform complex tasks.
- Electrical connectivity must also be established, which often requires advanced materials and techniques to maintain function without compromising the optical pathways.
Moreover, advancements in smart packaging options aim to enhance the functionality of optical MEMS. This innovation is aimed at achieving not just performance goals but also at reducing manufacturing costs and improving user experience.
"The choice of manufacturing technique in Optical MEMS production - be it lithography, etching, or packaging - inevitably shapes the future of optical technologies across industries."
Applications of Optical MEMS
The realm of Optical Micro-Electro-Mechanical Systems (MEMS) is not just a fascinating field of study; it is woven into the very fabric of modern technology. In examining the applications of Optical MEMS, we uncover how these systems drive advancements across multiple sectors. Their versatility in telecommunications, consumer electronics, and healthcare emphasizes their significant role in pushing the boundaries of what is possible in optical technology. As these applications evolve, they are reshaping industries and paving the way for innovative solutions.
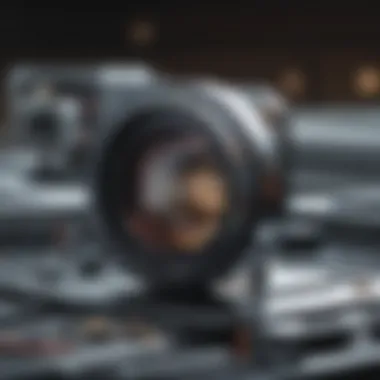
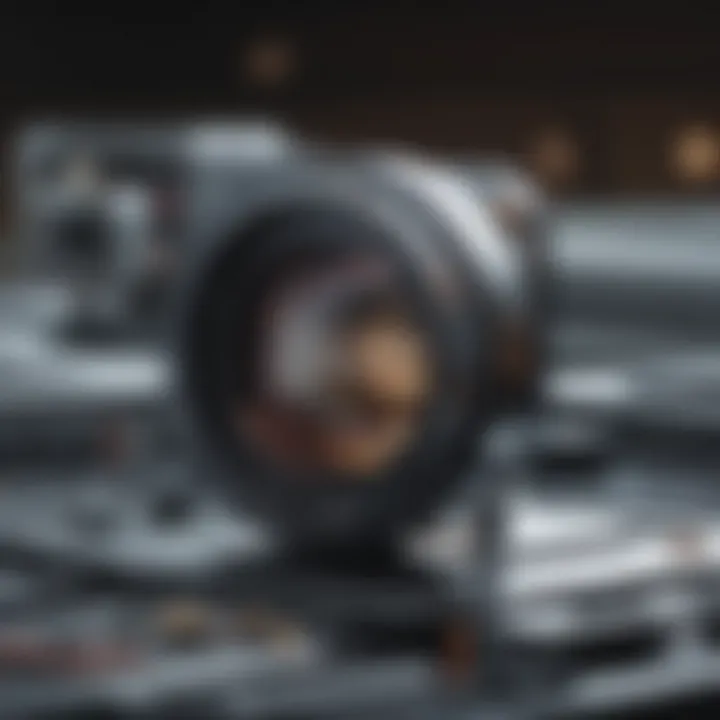
Telecommunications
Optical switches
Optical switches are pivotal components within the telecommunications landscape. They facilitate the routing of light signals between different pathways without converting them into electrical signals. This characteristic is crucial as it preserves bandwidth and reduces latency, key factors in high-speed communication networks. One of the unique features of optical switches is that they enable dynamic data routing, which helps in managing data traffic effectively. While they can streamline operations and enhance signal integrity, the complexity of their implementation and the need for precise alignment can be considered a disadvantage, requiring significant investment in infrastructure.
Signal processing
Signal processing within the realm of Optical MEMS has seen noteworthy advancements. These systems process optical signals for encoding, filtering, and amplification, thereby enhancing the quality of transmitted information. The key characteristic of optical signal processing is its capacity to handle vast amounts of data with minimal latency, making it a favorable choice for high-speed communications. Moreover, the ability to integrate signal processing functionalities directly onto optical chips provides compactness and efficiency. Nonetheless, the rapid evolution of this technology may bring challenges in terms of compatibility with existing systems and standardization across platforms.
Consumer Electronics
Camera systems
The integration of Optical MEMS in camera systems has initiated a revolutionary shift in how images are captured. Notably, the micro-mirrors used in these systems allow for autofocus and image stabilization, considerably improving image quality. The main advantage of these optical components is their ability to deliver high precision and speed in focusing, making them increasingly popular in both smartphones and professional cameras. However, their sensitivity to environmental conditions can be a limitation, potentially affecting performance in varying temperatures or humidity levels.
Augmented reality devices
In the domain of augmented reality, Optical MEMS contribute by enabling immersive experiences through advanced optics. The micro-lenses and modulators found in these devices allow for precise manipulation of light, which enhances image overlay on real-world views. The key benefit here is that they create seamless interactions in AR applications, enriching user engagement. On the flip side, the complexity of development and the need for powerful processing capabilities may pose challenges in achieving widespread adoption.
Healthcare and Biomedical Devices
Optical sensing
Optical sensing technologies are gaining traction in healthcare applications, providing non-invasive methods for monitoring and diagnosing various conditions. The beauty of optical sensing lies in its capacity to detect changes in light properties, which can indicate biological phenomena. This characteristic of real-time data collection aids health professionals in making timely intervention decisions, thus proving immensely beneficial. However, the cost of sophisticated optical sensors and the need for specialized training to interpret data can be hurdles that clinics and hospitals face.
Imaging technologies
Imaging technologies in the healthcare sector benefit enormously from Optical MEMS techniques. For instance, advanced imaging systems that utilize optical micro-mirrors can achieve unparalleled resolution and speed in medical diagnostics. One key feature is their ability to deliver high-quality images with minimal invasiveness, which is crucial for procedures like endoscopy. Although they offer significant advantages, the complexity of these systems and the need for high-quality materials can result in higher development costs, making broad implementation a bit challenging.
Challenges in Optical MEMS Technology
Navigating the landscape of Optical MEMS involves more than just innovation; it also encompasses a variety of challenges that can impede progress and adoption. Those involved in the field, whether engineers, researchers, or industry stakeholders, must be aware of these issues to effectively innovate and implement solutions. Addressing these challenges not only enhances the performance and durability of Optical MEMS but also broadens their applicability across various sectors. Let's dig into some of the key challenges that come with Optical MEMS technology.
Thermal Stability
Thermal stability is crucial for the efficient functioning of Optical MEMS devices. As these systems are often integrated into environments with fluctuating temperatures, the materials must maintain their performance even when subjected to heat or cold. Temperature changes can lead to deformation, affecting precision in optical devices. The consequences of these shifts can be significant—ranging from degradation of device performance to complete failure in critical applications like telecommunications.
To improve thermal stability, researchers are exploring various advanced materials. For example, silicon carbide (SiC) has emerged as a promising option due to its high thermal conductivity and reliability at varying temperatures. Similarly, composite materials that combine different properties may also be leveraged to enhance operational temperature ranges.
In application, any solution must be assessed thoroughly; otherwise, some benefits might be overshadowed by increased costs or complexity in manufacturing processes.
Mechanical Reliability
When it comes to mechanical reliability, Optical MEMS devices face intense scrutiny because their functionality is deeply intertwined with physical movements. Any mechanical failure can have dire effects, especially in applications involving precision optics. The small scale at which Optical MEMS operate means that even minute defects can lead to serious performance issues.
Stress and fatigue are two major factors affecting mechanical reliability. Over time, and after numerous cycles of actuation, components may experience wear and tear. This is where the design strategy becomes vital—utilizing finite element analysis can help in assessing potential points of failure and improving architectures for resilience.
Another direction for enhancing reliability focuses on lubrication systems. Incorporating advanced lubricants and coatings can reduce friction and wear, extending the operational life of MEMS devices. Thus, balancing performance with reliability in mechanical design plays a significant role in advancing Optical MEMS technology.
Cost and Scalability
Cost and scalability present pivotal challenges in Optical MEMS technology. The manufacturing processes often involve expensive raw materials and intricate fabrication techniques, which can drive up costs significantly. Hence, there is a pressing need for cost-effective production methodologies that don’t compromise quality.
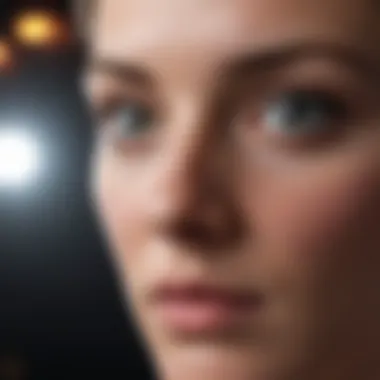
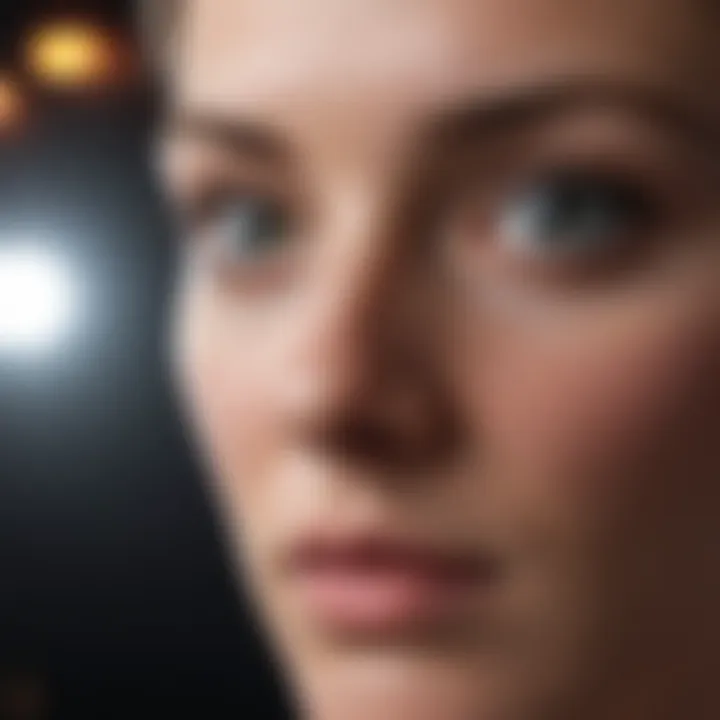
One approach being explored is the transition to large-scale production techniques such as batch processing, akin to semiconductor manufacturing. By streamlining production processes, it may be feasible to reduce unit costs considerably. Alongside this, research into new materials may also lead to less expensive options without sacrificing performance.
Furthermore, the scalability of Optical MEMS technology is closely tied to the complexity of integration within existing systems. As functionality increases, ensuring that these devices can be integrated into existing manufacturing processes becomes essential. Scalability isn't just about production; it's also about making sure that the resulting devices perform reliably in the field and meet the demands of end-users.
Tackling these challenges head-on can open doors for Optical MEMS technologies, enabling their wider usage and enhancing overall user experience.
Future Trends in Optical MEMS
Optical MEMS technology is moving at breakneck speed, and understanding the future trends is crucial for both researchers and industry experts alike. With applications ranging from telecommunications to portable electronic devices, keeping an eye on what’s coming next can help shape innovations and guide effective investments. One specific trend is the integration of advanced technologies that boost performance while reducing costs. The implications of these advancements are far-reaching, impacting not just the products we use but also the way we interact with technology.
Emerging Technologies
Nano-scale MEMS
One of the most exciting aspects in the future of optical MEMS is the development of nano-scale MEMS. This technology dives deep into the realm of miniaturization, pushing the boundaries of what’s possible in optical functionalities. The main characteristic of nano-scale MEMS is its ability to manipulate light at incredibly small dimensions. This leads to devices that are not just smaller but also potentially more efficient and powerful. Being able to produce optical components at the nanoscale allows for numerous applications, from enhancing sensors in smartphones to developing advanced optical data transmission systems.
Nano-scale MEMS can substantially improve wavelength resolution, making them a favored choice in optical filtering. Nevertheless, they come with their own set of challenges, including difficulties in manufacturing consistency and unforeseen effects arising from quantum scale phenomena.
Smart optics
Smart optics present another crucial leap forward in optical MEMS technology. This field emphasizes adaptability and intelligent processing in optical systems, thereby enhancing performance. One key feature of smart optics is their ability to dynamically adjust focal lengths and other optical properties in real-time. This adaptability makes smart optics an excellent choice for improving augmented reality devices and intelligent imaging systems.
The unique aspect of smart optics lies in their integration with AI, which allows them to learn and optimize performance based on usage patterns. On the flip side, implementing these systems can be complex and costly, raising questions about their scalability in consumer-grade products.
Research Directions
Integration with AI
The fusion of optical MEMS with artificial intelligence (AI) is not just a whim; it’s a trend with lingering implications for the industry. By automating tasks like image processing and data analysis, AI can significantly enhance the capabilities of optical systems. What's more, the integration allows for predictive maintenance, which reduces downtime for devices.
A notable characteristic of this trend is its potential for creating self-adjusting systems that optimize performance based on environmental conditions or user needs. However, the complexity and cost of integrating AI can pose challenges for smaller companies, making this not an all-encompassing solution but rather one that will likely evolve over time.
Advanced materials
Advanced materials are taking the spotlight in shaping the future of Optical MEMS. With innovations like flexible materials and composites, optical devices can be made lighter, more durable, and more efficient. The special feature of these advanced materials is their ability to withstand extreme conditions, thereby extending the life of optical systems in harsh environments.
Incorporating advanced materials not only enhances device performance but does so while meeting growing sustainability standards. However, the availability of these materials and the expertise required for their use can be limiting factors for broad adoption, requiring careful consideration in future developments.
As we look ahead, it becomes increasingly clear that adaptability and performance optimization will be the cornerstone of future innovations in optical MEMS.
Culmination
In wrapping up our discussion on Optical MEMS, it’s clear that this field represents a captivating blend of innovation and application across various industries. The significance of Optical Micro-Electro-Mechanical Systems cannot be overstated; they serve as pivotal components in enhancing optical devices, facilitating high-speed telecommunications, and enabling sophisticated healthcare diagnostics. Through this article, we have explored not just the technology itself, but also its historical journey, the challenges it faces, and the promising future that lies ahead.
Summary of Findings
To summarize our findings:
- Innovation and Technology: Optical MEMS technology is at the forefront of modern optical communication, enabling faster data transmission and smoother signal processing.
- Diverse Applications: From telecommunications to consumer electronics and healthcare, the applications of optical MEMS are broad and varied, touching many facets of daily life.
- Challenges and Considerations: Despite the potential, challenges such as thermal stability, mechanical reliability, and scaling production pose significant hurdles that must be addressed.
- Future Directions: The integration with advanced technologies like AI and the development of new materials signal the ongoing evolution of optical MEMS.
This comprehensive analysis illustrates the current landscape of Optical MEMS technology and its indispensable role in future innovations.
Implications for the Future
Looking ahead, the implications of advancements in Optical MEMS are vast. As technology evolves, we can anticipate:
- Increased Efficiency: Enhanced optical MEMS will likely lead to greater efficiency in data communications, reducing latency and improving user experience in telecommunications.
- Integration with AI: The synergy of AI with MEMS technology could result in smarter optical devices that can adapt and learn from user inputs, enabling more personalized experiences.
- Cost Reduction: Ongoing innovations may lead to decreased manufacturing costs, making Optical MEMS technology accessible to a wider range of applications and consumers.
- Sustainability: With more focus on sustainable materials and practices, the future of Optical MEMS may align with environmentally friendly initiatives, supporting global sustainability goals.
"As Optical MEMS technology continues to advance, the possibilities are limited only by our imagination and commitment to innovation."