Exploring Stirring Bioreactors: Principles and Innovations
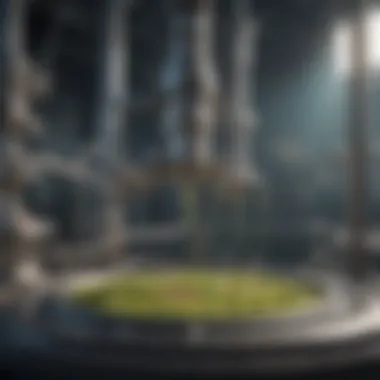
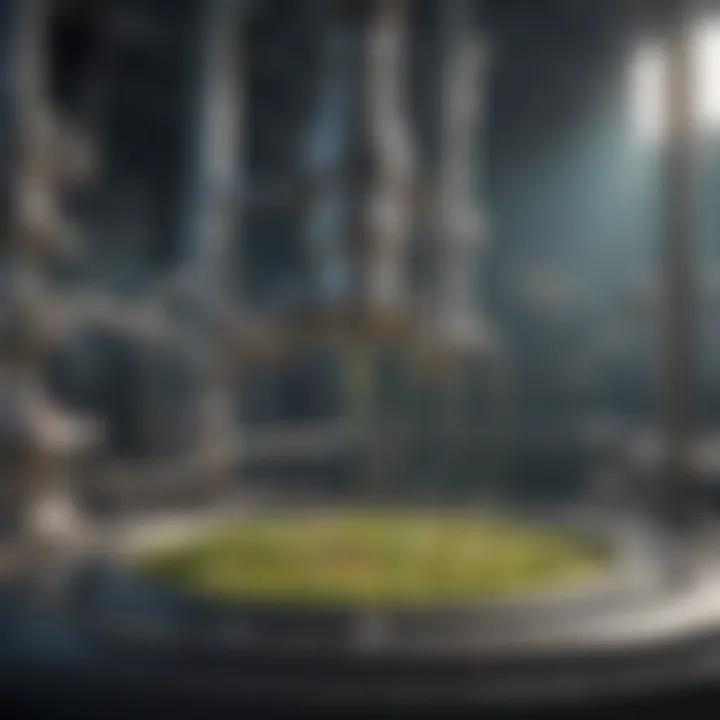
Intro
In the realm of biotechnological processes, stirring bioreactors stand out as crucial components for enhancing various chemical and biological reactions. These systems facilitate the mixing of substrates and cells to optimize growth and production yields. This article aims to provide an in-depth examination of these vital systems, highlighting their operational principles, applications, and advancements.
Stirring bioreactors utilize mechanical agitation to create uniform conditions within the reactor. This is essential for many industries, including pharmaceuticals, environmental biotechnology, and food science. As research progresses, understanding the dynamics of these systems becomes increasingly important for effective application.
By exploring diverse types of stirring systems, their mechanisms, and the influencing factors, this article will forge a comprehensive narrative that serves as a valuable resource for students, researchers, educators, and professionals in the field.
Research Overview
Summary of Key Findings
Recent studies have shown that stirring bioreactors can significantly enhance mass and heat transfer, which directly affects reaction yields. Specific findings include:
- Enhanced mixing results in higher nutrient availability for microorganisms.
- Optimal stirring speed can reduce shear stress, preserving cell viability in sensitive cultures.
- Innovations like impeller design and control strategies have greatly improved efficiency.
Methodologies Employed
The investigation employed a range of methodologies, such as computational fluid dynamics (CFD) simulations and experimental trials using different reactor designs. These approaches enabled the analysis of mixing patterns, turbulence levels, and overall reactor performance.
In-Depth Analysis
Detailed Examination of Results
The results indicated that various reactor configurations yield different efficiencies. For instance, reactors equipped with radial flow impellers generally provide more homogeneous mixing compared to axial flow impellers. However, the choice of design depends on the specific application, such as microbial fermentation or enzyme immobilization.
Comparison with Previous Studies
Comparing these findings with prior studies reveals a trend towards the adoption of more advanced technologies. Many researchers have noted an increase in the applicability of bioengineering principles, particularly in optimizing bioreactor designs. Previous methods often relied on traditional paddle mixers, which lacked the efficacy of modern solutions.
"Understanding the underlying principles of stirring bioreactors is essential for maximizing yields and efficiency."
By synthesizing current data with historical insights, the narrative of stirring bioreactors becomes clearer, revealing the advancements made and the road ahead for future research.
Prelude to Stirring Bioreactors
Stirring bioreactors play a vital role in biotechnological processes. They are essential for cultivating microorganisms, cells, and tissues. These systems enhance the efficiency of biological reactions through proper mixing and aeration. The integration of stirring mechanisms increases the mass transfer rates, allowing for optimal growth conditions. Thus, understanding stirring bioreactors is crucial for professionals in diverse fields such as pharmaceuticals, environmental science, and food technology.
Definition and Purpose
A stirring bioreactor can be defined as a vessel that provides controlled environments for biological processes. These bioreactors use stirring mechanisms to ensure uniform suspension of cells, improve nutrient distribution, and enhance gas exchange. Their purpose extends beyond mere mixing; they are designed to create optimal conditions for growth and productivity. Key benefits of stirring bioreactors include:
- Improved mass transfer: Enhanced transfer of nutrients and gases to organisms.
- Uniformity: Consistent conditions throughout the culture, which is critical in achieving a homogenous product.
- Scalability: Easily adaptable to larger volumes for mass production.
By ensuring efficient mixing, stirring bioreactors significantly contribute to the outcome of various biotechnological applications.
Historical Context
The technology of stirring bioreactors has evolved over many decades. In the early 20th century, simple shaking flasks were commonly used for culturing microorganisms. These flasks provided rudimentary mixing but were limited in controlling environmental factors.
As research progressed, the introduction of bioreactor systems started in the mid-20th century. The invention of mechanically stirred tank reactors marked a turning point. These systems enabled better control over aeration and temperature. This advancement paved the way for industrial applications, particularly in the pharmaceutical sector.
In the 1970s and 1980s, the development of more advanced bioreactors, such as submerged fermenters and air-lift reactors, further enhanced performance. Significant innovations in materials and sensors also contributed to the precision and reliability of stirring bioreactors.
Understanding this historical context illustrates not only the advancements in stirring technologies but also their increasing importance in various industries. This sets the stage for dissecting their current applications and future prospects.
Mechanics of Stirring Bioreactors
Understanding the mechanics of stirring bioreactors is fundamental for optimizing the performance and efficiency of biological processes. The design and operational parameters of stirring systems significantly influence the homogeneity of the culture medium, enabling effective mass transfer and nutrient distribution. In this context, examining different stirring mechanisms and the fluid dynamics involved is essential for achieving desired outcomes in biotechnological applications.
Types of Stirring Mechanisms
Stirring mechanisms can be broadly categorized into various types, each serving unique purposes based on the specific requirements of the bioreactor system:
- Magnetic Stirring: Utilizes a magnetic field to drive a stir bar, providing a clean and efficient mixing process. This method is commonly applied in small-scale bioreactors and has minimal risk of contamination.
- Mechanical Agitation: Involves the use of impellers, fans, or stirrers directly connected to a motor. This type of stirring is prevalent in larger vessels, ensuring adequate mixing and aeration.
- Ultrasonic Stirring: Leverages ultrasonic waves to create cavitation bubbles, which enhance mixing without moving mechanical parts. This innovative approach can be useful in sensitive biological applications where shear forces need to be minimized.
- Pneumatic Mixing: Uses air or gas to create turbulence within the liquid. This method is often favored in applications where fermentation processes require both mixing and aeration.
Each of these mechanisms has its advantages, and the choice will depend largely on the scale of operation, the shear sensitivity of the cells, and the desired mixing intensity.
Fluid Dynamics in Bioreactors
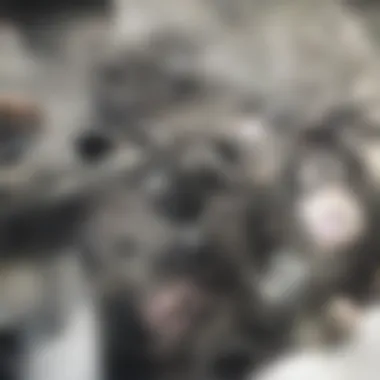
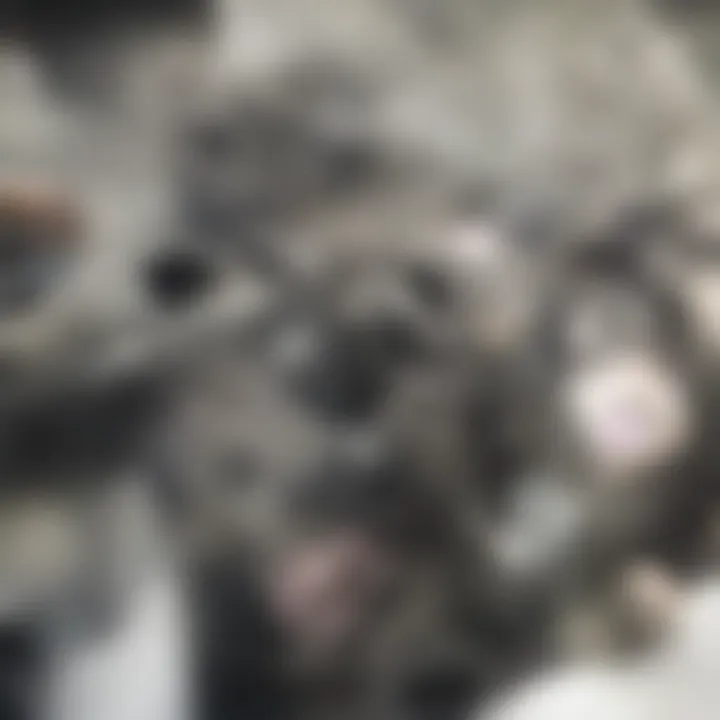
Fluid dynamics play a critical role in determining how effectively nutrients and cells interact within the bioreactor. Key aspects to consider in fluid dynamics include flow patterns, shear rates, and mixing times:
- Flow Patterns: The design of the bioreactor should facilitate efficient flow patterns, such as laminar or turbulent flow, to avoid significant gradients in concentration or temperature. Proper design reduces dead zones where cells and nutrients stagnate, optimizing performance.
- Shear Rates: Different cell types exhibit varying sensitivities to shear stress. It is important to measure and control shear rates in the reactor to protect delicate cells while maintaining effective mixing.
- Mixing Times: Achieving uniformity in the culture medium is essential. Studies have shown that appropriate mixing times lead to improved yield and product quality in fermentation processes.
In summary, thorough consideration of the mechanics of stirring bioreactors enables researchers and practitioners to enhance operational efficiencies. The interrelated factors of stirring mechanisms and fluid dynamics provide a foundation for designing systems that meet the specific needs of various biotechnological applications.
"The performance of bioreactors is highly dependent on the type of stirring mechanism employed and the fluid dynamics within the reactor."
By systematically analyzing these components, one can develop optimized bioreactor configurations that meet both scientific and industrial goals.
Design Considerations
In the realm of stirring bioreactors, design considerations are paramount. These aspects directly affect not only the efficiency of the bioreactor but also its operational stability and effectiveness in various applications. The complexity of biological processes necessitates a thoughtful approach to design. Here, we will explore three primary elements: geometrical configurations, material selection, and scalability factors.
Geometrical Configurations
The geometry of a stirring bioreactor significantly influences the fluid flow and mixing efficiency. Different shapes, such as cylindrical or rectangular vessels, serve distinct purposes. For example, a cylindrical bioreactor provides uniform mixing and is more efficient in terms of space utilization.
Consider the following points when evaluating geometrical configurations:
- Mixing Efficiency: The shape must facilitate optimal mixing of the broth, ensuring proper nutrient distribution and metabolic activity.
- Volume Consideration: Larger vessels require particular attention to avoid dead zones where mixing is minimal.
- Scaling Up: It is essential to design the geometry for scalability to larger formats while maintaining performance.
Each configuration offers advantages and challenges that must be balanced against the intended use of the bioreactor.
Material Selection
Material selection plays a crucial role in ensuring bioreactor longevity and compatibility with biological processes. The materials used in constructing bioreactors must withstand harsh conditions. Key factors to consider include:
- Corrosion Resistance: The interior should resist corrosion from reactive components in the medium.
- Biocompatibility: Ensuring the selected materials do not leach harmful substances into the culture is critical for maintaining cell health.
- Thermal Stability: Materials must withstand temperature variations without compromising structural integrity.
Stainless steel and polysulfone are popular choices due to their durability and interactions with biochemical reactions.
Scalability Factors
Scalability is an important consideration when designing stirring bioreactors, especially in the context of moving from laboratory-scale to industrial-scale production. Effective scalability involves numerous considerations, including:
- Process Replicability: The operational parameters must be easy to replicate across different scales to ensure consistent outcomes.
- Resource Management: Efficient scaling must also manage inputsβlabor, energy, and materialsβto minimize costs while maximizing yield.
- Performance Evaluation: Regular assessment of both small-scale and large-scale systems assists in understanding potential performance issues.
Scalability connects all the previous design considerations and creates a foundation for successful biotechnology developments.
Operational Conditions
Operational conditions in stirring bioreactors are critical for achieving optimal performance and product yield. These conditions, encompassing temperature control, pH levels, and nutrient delivery systems, create an environment conducive to the growth of microorganisms or cells used in bioprocessing. Understanding and controlling these variables is essential for ensuring consistent results in various applications, from pharmaceuticals to environmental biotechnology.
Temperature and pH Control
Temperature plays a vital role in microbial metabolism and overall cell viability. Most organisms have specific optimal temperature ranges. Deviations can lead to reduced growth rates, viability, and product formation. Maintaining an appropriate temperature often requires sophisticated heating and cooling systems. Automated systems are typically employed to regulate temperature precisely, using heaters along with cooling jackets or fluid circulation systems.
pH control is equally important. Each microorganism thrives within a certain pH range. Eukaryotic cells, for instance, often require a neutral to slightly basic environment, while some bacteria may favor acidic conditions. Adequate monitoring and adjustment of pH can prevent harmful metabolic byproducts' accumulation, which might adversely affect cell growth and productivity.
Accurate temperature and pH control directly correlate with product quality and process efficiency.
Both temperature and pH must be monitored continuously. Sensors and control loops are important tools that allow for real-time adjustments. Implementing these control systems ensures optimal operational guidelines are followed.
Nutrient Delivery Systems
Nutrient delivery systems are crucial for sustaining cell growth and metabolism in bioreactors. These systems must provide the right quantities of essential nutrients, including sugars, amino acids, vitamins, and minerals. The manner and timing of nutrient addition can significantly impact the overall growth of microorganisms and the production of desired metabolites.
Common strategies for nutrient delivery include continuous feeding methods and batch strategies. In continuous feeding, nutrients are added constantly or at regular intervals to maintain optimal concentrations. This method supports prolonged growth phases and can enhance productivity. On the other hand, batch strategies involve adding all nutrients at the beginning of the cycle. While simpler, this approach can lead to nutrient depletion or accumulation of toxic byproducts if not carefully managed.
It is also essential to consider the solubility and availability of nutrients. Some nutrients might precipitate out, rendering them ineffective. Using well-formulated nutrient solutions and incorporating monitoring systems for nutrient concentration can mitigate these challenges.
In summary, operational conditions, especially temperature and pH control along with nutrient delivery systems, are paramount for maximizing the efficiency of stirring bioreactors. Proper management of these factors not only enhances cell growth but also ensures high-quality product yields.
Applications of Stirring Bioreactors
Stirring bioreactors play a pivotal role in various industries by facilitating controlled biological reactions. Their applications span multiple fields, including pharmaceuticals, environmental biotechnology, and food science. Understanding these applications is essential, as they highlight the versatility and effectiveness of stirring bioreactors in contemporary biotechnology practices. Each application carries specific benefits and considerations, which enhance their allure in research and industry.
Pharmaceutical Production
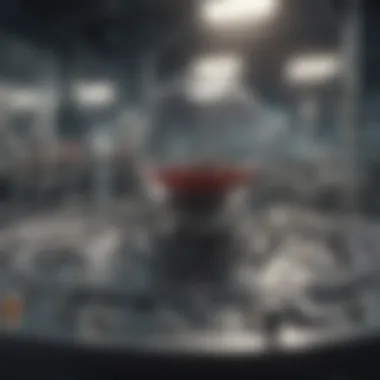
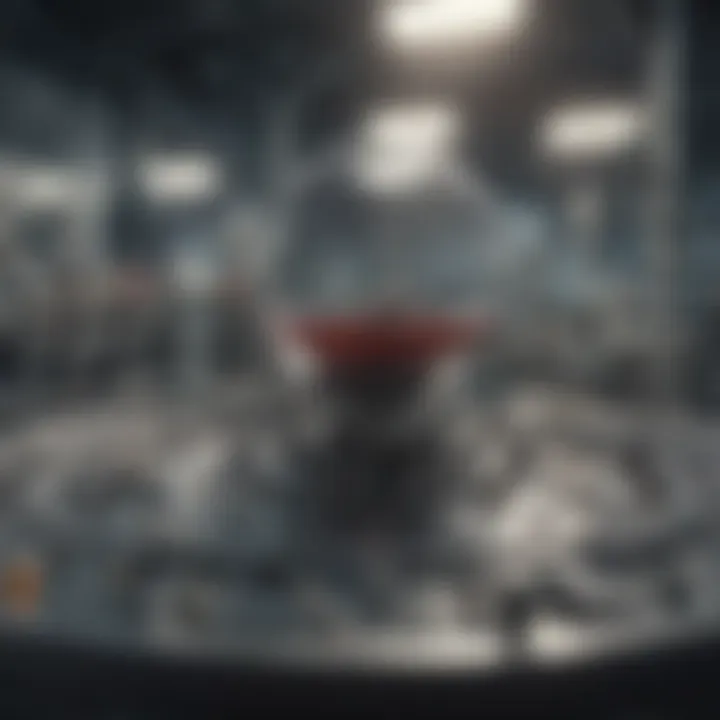
In the pharmaceutical industry, stirring bioreactors are indispensable for the production of biologics and pharmaceuticals. These bioreactors enable the cultivation of microorganisms, cells, or tissues under controlled conditions, optimizing the output of desired compounds. For example, monoclonal antibodies, needed for therapies, are often produced in bioreactors. The stirring action ensures proper mixing and oxygen delivery, which are critical for cell growth and productivity. Ensuring consistent conditions in pharmaceutical production can greatly reduce batch variability and increase overall yield.
Considerations in pharmaceutical production include:
- Batch size: The scale of production often dictates the choice of bioreactor. Larger volumes may require more intricate systems.
- Sterility: Maintaining a sterile environment is essential to prevent contamination, making material selection for bioreactors vital.
- Yield and quality: Optimization strategies must be employed to improve both yield and quality of the final products.
Environmental Biotechnology
Stirring bioreactors are crucial in environmental biotechnology, where they help in the treatment of wastewater and the bioremediation of contaminated environments. These systems enhance the degradation of pollutants by creating optimal conditions for microbial activity. For instance, in the treatment of industrial effluents, stirring bioreactors ensure that microorganisms maintain contact with the pollutants longer, increasing degradation rates. This is particularly relevant in situations where quick remediation is necessary to protect ecosystems.
Key factors that enhance their effectiveness include:
- Nutrient management: Regular monitoring and adjusting nutrient levels can significantly impact microbial growth and pollutant degradation.
- Oxygen transfer: Efficient stirring promotes oxygen transfer, which is vital for aerobic microbial processes.
- Temperature control: Stable temperatures support consistent microbial performance, directly impacting the efficiency of bioremediation processes.
Food Science Innovations
In the realm of food science, stirring bioreactors contribute profoundly to the production of fermented foods and feedstock. Fermentation processes, such as those used in brewing beer and producing yogurt, benefit from controlled stirring, which promotes uniform mixing and enzyme activity. The ability to maintain control over fermentation parameters leads to reproducible quality and flavor profiles in products.
Important considerations in food science applications include:
- Fermentation time: Precision in timing can affect the flavor and texture of food products.
- Microbial cultures: Selecting the appropriate strains is vital for the desired results in fermentation.
- Regulation and safety: Food production must meet strict safety standards, necessitating careful monitoring of all bioreactor operations.
Consistent and optimized applications of stirring bioreactors in pharmaceuticals, environmental biotechnology, and food science underscore their fundamental role in modern biotechnology.
Through a comprehensive examination of these applications, it becomes clear that stirring bioreactors are an integral component in achieving efficiency and innovation in various sectors. Their significance cannot be overstated as industries continue to evolve and adopt stricter demands for productivity and sustainability.
Efficiency and Performance Metrics
Efficiency and performance metrics are crucial in understanding the overall effectiveness of stirring bioreactors. These metrics not only reflect the operational capabilities of the bioreactor systems, but also guide improvements and innovations in biotechnological applications. By assessing these metrics, researchers and industry professionals can identify bottlenecks, optimize processes, and enhance product yields. Furthermore, focusing on efficiency promotes sustainability, which is increasingly essential in modern biotechnology.
Key elements of efficiency metrics include:
- Energy Consumption: Monitoring energy use informs how effectively a bioreactor operates. Lower energy consumption while maintaining high output is a goal for many operations.
- Mixing Time: The time taken to achieve homogeneous conditions within the reactor affects overall performance. Shorter mixing times can lead to faster processing and higher productivity.
- Cell Growth Rates: Tracking how efficiently organisms grow in the bioreactor indicates the effectiveness of nutrient delivery and environmental conditions.
These factors contribute significantly to achieving desired performance outcomes.
Yield Optimization Strategies
Yield optimization strategies focus on maximizing the output of biotechnological processes while reducing waste and resource usage. To achieve this, several techniques can be applied:
- Adjustment of Stirring Speed: The speed at which the agitator operates can influence mixing and mass transfer. Finding the optimal speed is critical for enhancing yield.
- Nutrient Optimization: Tailoring nutrient concentrations can lead to better cell growth and productivity. Careful monitoring of nutrient levels is essential.
- Temperature Control: Maintaining ideal temperature conditions boosts metabolic rates, leading to increased yields. Precise control mechanisms are essential for this.
Implementing these strategies requires a detailed analysis of the specific bioprocess and organism involved. Continuous monitoring of performance metrics aids in refining these strategies over time.
Monitoring and Quality Control
Monitoring and quality control are integral to maintaining the efficiency of stirring bioreactors. Effective monitoring allows for real-time assessment of various parameters such as pH, temperature, and dissolved oxygen levels.
- Real-Time Data Collection: Utilizing sensors and automated systems enhances the ability to track performance metrics. This data is key to making prompt decisions regarding process adjustments.
- Quality Standards: Adhering to established quality control standards ensures that products meet regulatory requirements and specifications. Regular calibration of equipment is necessary to uphold these standards.
"Consistent monitoring is the backbone of any successful bioprocess. It enables rapid response to deviations and aids in maintaining product quality."
Adopting rigorous monitoring and quality control practices not only improves reliability but also enhances the reputation of the production processes within the industry.
Recent Technological Advancements
In recent years, the field of stirring bioreactors has witnessed significant technological advancements that enhance both efficiency and performance. These developments not only improve operational control but also contribute to meeting the ever-growing demands of various industries. Understanding these advancements is crucial for researchers and professionals aiming to optimize bioreactor systems.
Automation and Control Systems
Automation in stirring bioreactors involves the integration of sophisticated control systems that manage various operational parameters. This is essential because precise control over variables such as temperature, pH, and agitation speed is critical for maximizing productivity and ensuring consistent product quality.
Benefits of automated systems include:
- Increased Precision: Automated systems minimize human error, ensuring that operations remain within specified parameters.
- Real-Time Monitoring: These systems allow for continuous observation of the bioreactor environment, enabling timely adjustments to maintain optimal conditions.
- Data Logging: Automation provides a detailed record of each batch's performance, which is invaluable for troubleshooting and process improvement.
Operational flexibility is another advantage. Automated bioreactors can easily switch between different modes of operation, allowing for adaptability in various production processes. This capability is particularly important in research settings where trial and error are common.
To implement a successful automation strategy, it is crucial to select control systems that align with the specific needs of the bioprocess. Factors such as compatibility with existing equipment, user-friendliness, and support services must be carefully evaluated.
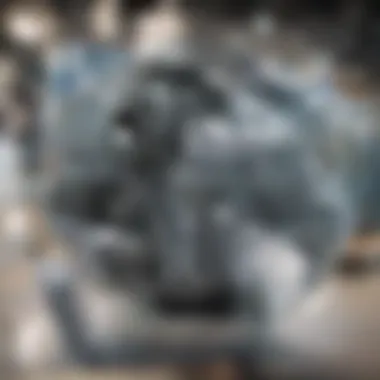
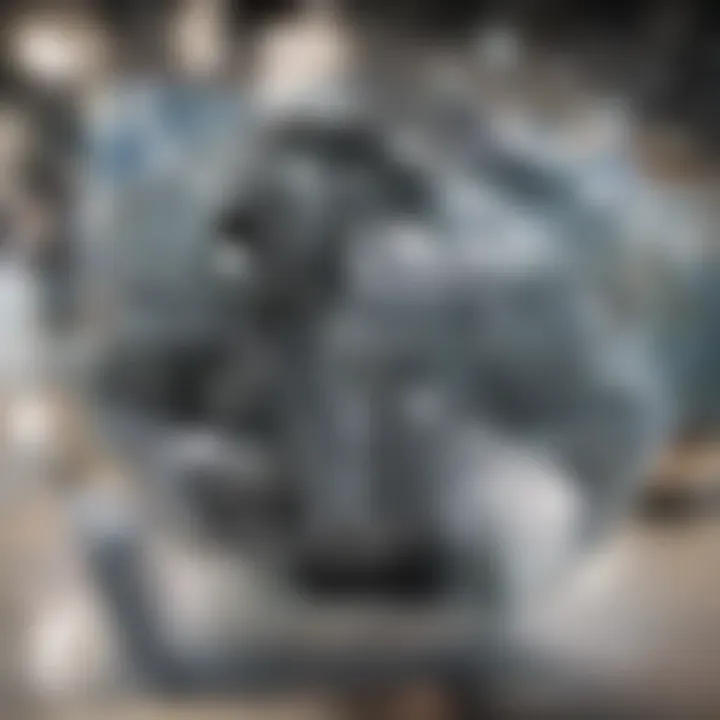
Integration of Artificial Intelligence
The integration of artificial intelligence (AI) into bioreactor technology represents a pioneering frontier. AI systems enhance data analysis and decision-making processes by utilizing machine learning algorithms to predict outcomes based on historical data.
Key aspects of AI integration include:
- Predictive Analytics: AI can forecast ideal operational settings, helping in dynamic adjustments that enhance yield.
- Process Optimization: By analyzing complex datasets, AI can suggest modifications to equipment and procedures that improve efficiency.
- Quality Assurance: AI can identify deviations from expected performance, assisting in maintaining product quality through real-time alerts.
Nonetheless, AI integration comes with considerations such as the need for extensive data sets to train models effectively. Institutions must also invest in infrastructure to support these advanced technologies.
βIntelligent bioreactor systems demonstrate a remarkable ability to learn from their environments, paving the way for more sustainable and productive biotechnology processes.β
As bioreactor technology continues to evolve, the combination of automation and AI will likely play a pivotal role in shaping the future of biotechnology. Continued investment in these areas is essential to harness their full potential and drive innovation across multiple sectors.
Challenges and Limitations
The examination of stirring bioreactors reveals significant challenges and limitations that can affect their efficiency and effectiveness. Understanding these issues is crucial for both research and practical applications. Addressing them can lead to improved designs and operational practices in various fields, including pharmaceuticals, environmental biotechnology, and food science.
Scale-Up Issues
One of the primary challenges encountered with stirring bioreactors is scaling up processes from laboratory to industrial levels. Scale-up involves taking a process that works on a small scale and adapting it to larger volumes without compromising product quality or operational efficiency.
Several factors contribute to scale-up issues. The fluid dynamics, mixing efficiency, and shear forces all change as the reactor size increases. For instance, the mixing time in a large bioreactor may not be equivalent to that in a smaller one. Furthermore, the heat transfer characteristics may vary widely between scales, leading to undesired temperature gradients that can affect microbial activity.
Often, even minor changes in geometrical configurations can significantly impact performance. Engineers must carefully consider these differences while designing reactors to ensure consistent results at larger scales. Collaborations between researchers and industry professionals are essential to share insights and address these complexities effectively.
Energy Consumption Concerns
Energy consumption is another important limitation in the operation of stirring bioreactors. The stirring mechanism typically accounts for a significant portion of the energy needed during the entire fermentation process. This can greatly impact operational costs and the overall sustainability of bioprocesses.
Optimizing energy consumption is critical because it affects the economic viability of bioproducts. Higher energy costs can make certain processes less competitive in the market. Industry stakeholders are encouraged to look for more energy-efficient stirring methods, which can include the use of advanced materials and technologies.
Additionally, energy considerations must be balanced against process outcomes. It is vital to achieve an effective mixing environment while minimizing energy usage. Efforts such as employing variable speed drives or integrating automated systems can help address these concerns.
"Efficiency in energy consumption directly impacts the bottom line of biotechnological processes, making it a priority for ongoing research and innovation."
In summary, the challenges of scale-up and energy consumption present essential obstacles in the deployment of stirring bioreactors. Overcoming these limitations will not only enhance operational efficiency but also promote sustainability in biotechnological applications.
Future Directions in Bioreactor Technology
Exploring future directions in bioreactor technology is essential for understanding how these systems can evolve to meet changing demands. Bioreactors must adapt to the urgent need for sustainability and efficiency in various industries. This segment considers significant innovations on the horizon, focusing on two main aspects: sustainability approaches and emerging research fields.
Sustainability Approaches
Sustainability in bioreactor technology is increasingly vital as industries strive for greener practices. Traditional bioreactor systems often rely on energy-intensive processes and can lead to waste generation. Therefore, developing more sustainable approaches is critical.
- Resource Optimization: Using raw materials more efficiently reduces waste. Implementing closed-loop systems can minimize resource depletion.
- Renewable Energy Sources: Integrating renewable energy solutions, such as solar or wind power, can dramatically decrease the carbon footprint of bioreactors.
- Biomass Utilization: Utilizing waste biomass as feedstock promotes a circular economy. This can increase the resource base without relying solely on virgin materials.
Through these methods, bioreactors can contribute significantly to more sustainable production processes, ensuring ecological balance while meeting industrial needs.
Emerging Research Fields
Research on bioreactor technology continues to grow, leading to exciting fields of study that promise innovation. Important topics gaining traction include:
- Synthetic Biology: Developing genetically modified organisms to improve yield and reduce by-products. Techniques in synthetic biology can lead to tailored strains that perform better in bioreactor environments.
- Advanced Materials: Research into new materials for bioreactor construction that enhance durability while being environmentally friendly is crucial. These materials can potentially improve heat transfer and reduce contamination risks.
- Digital Twins: This emerging field involves creating virtual models of bioreactors for real-time simulation and monitoring. Digital twins can optimize performance by predicting outcomes based on varying operational conditions.
These areas offer pathways for enhanced efficiency and functionality in bioreactor systems. As they develop, they will change how industries operate and improve the sustainability of biotechnological processes.
Culmination
In this article, we have delved into the intricate world of stirring bioreactors, highlighting various aspects that make them vital to numerous industries. The pivotal role of stirring mechanisms cannot be overstated. They influence not only the quality of the end products but also the operational efficiency of the bioreactor systems. Understanding the dynamics at play and the mechanisms involved allows researchers and engineers to optimize existing processes or design new systems more effectively.
Summary of Key Insights
The exploration of stirring bioreactors uncovered several critical insights:
- Definition and Mechanisms: Stirring bioreactors are designed to maintain homogeneous conditions in bioprocesses, ensuring consistent product quality. Different stirring mechanisms are available, such as mechanical agitation and airlift systems, each suitable for specific applications.
- Design Considerations: The geometrical configuration and material selection play a crucial role in determining the bioreactor's effectiveness. Equally important are the scalability factors that affect how a process can transition from laboratory to industrial scale.
- Operational Conditions: Effective control over temperature, pH, and nutrient delivery is essential for optimizing fermentation processes and maximizing yields.
- Applications and Advancements: The integration of automation and artificial intelligence in bioprocessing is shaping the future of bioreactor technology, making production more efficient and reliable.
Implications for Research and Industry
The findings presented in this article have several implications:
- Advancements in Bioprocessing Technologies: The insights gained can guide future research directions. As biotechnological processes become more sophisticated, understanding the science behind stirring bioreactors is essential for developing cutting-edge solutions.
- Industry Adoption Strategies: Industries need to embrace these findings to enhance productivity in sectors like pharmaceuticals, food production, and environmental management. Investment in better-designed bioreactors could lead to substantial improvements in operational efficiency and product quality.
- Policy and Training: With the technological evolution, there is a pressing need for updated training programs for professionals in the field. Educating the workforce about new bioreactor concepts and their applications can significantly impact research outcomes and industry practices.
"The future of stirring bioreactor technology hinges on a combination of practical knowledge and innovative thinking."