Exploring Scientific Facts: Insights into Our Universe
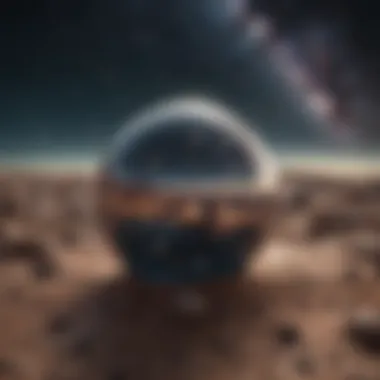
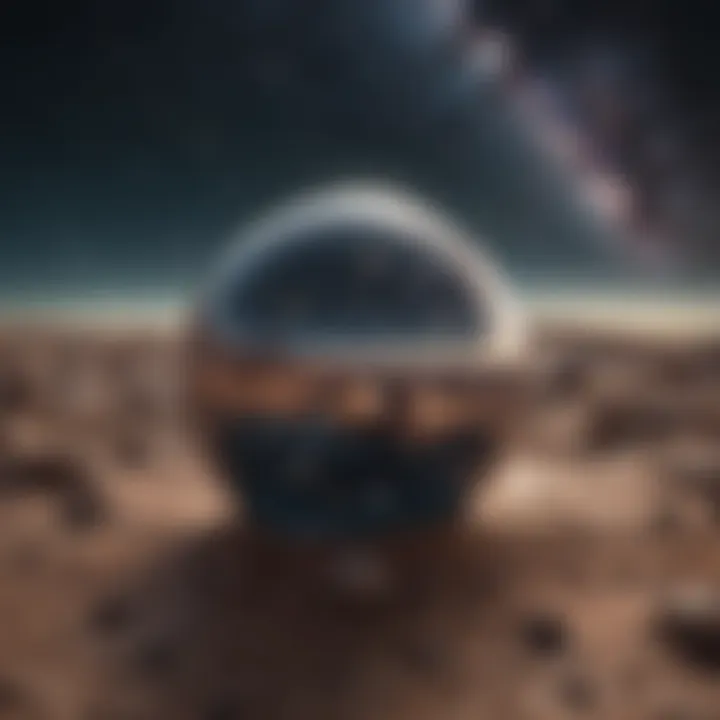
Intro
In our journey to grasp the universe and its workings, it becomes essential to ground ourselves in solid scientific facts. These facts are not just isolated pieces of information; they form the building blocks of understanding. From the tiniest particles to massive galaxies, science offers a lens through which we can perceive phenomena and draw meaningful connections.
Delving into scientific inquiry isn’t merely an academic pursuit—it's a pathway to informed decision-making in everyday life. Take, for instance, climate change and its cascading effects on earth’s ecosystems. Knowledge derived from rigorous scientific research can equip individuals, policymakers, and communities to tackle pressing issues effectively.
This article aims to peel back layers of complexity surrounding scientific facts. We will explore the origins, validation processes, and significance of these facts across various disciplines, such as biology, chemistry, physics, and earth sciences.
By doing so, we hope to foster a mutual appreciation for the sciences, sparking discussions that resonate among students, researchers, educators, and professionals alike. Scientific literacy can empower individuals and society to engage with the world thoughtfully.
Let’s embark on this journey towards a deeper understanding of our universe, starting with a comprehensive research overview.
Foreword to Scientific Facts
Understanding the nuances of scientific facts is fundamental to our grasp of the world around us. This section is not merely a preamble; it sets the stage for a deeper inquiry into the principles that govern the universe and our place within it. Here, we unpack the essence of what scientific facts are, their implications, and their roles across disciplines.
Scientific facts provide a framework through which we interpret not just the natural world, but also the technological advancements and ethical considerations that shape society. They are not static truths, but rather reflections of rigorous investigation and validation processes that evolve as we discover more about our universe. This exploration aims to inspire critical thinking, something absolutely vital for students and professionals alike.
Definition and Scope
When talking about scientific facts, one must first establish clear definitions. At its core, a scientific fact is an observation that has been repeatedly confirmed and accepted as true. Unlike opinions or assumptions, these facts stand on layers of evidence and serve as benchmarks within the scientific community. Terms like "hypothesis," "theory," and "law" often pop up in discussions around scientific facts. However, it is important to distinguish these from mere observations; whereas a fact can be confirmed, theories and hypotheses offer explanations that are subject to ongoing testing and validation.
The scope of scientific facts extends into various domains such as biology, chemistry, physics, and even social sciences. Each discipline has its own set of facts that are essential for building knowledge structures. In fact, scientific collaboration often requires a shared understanding of these fundamental facts, especially as research becomes more interdisciplinary.
Historical Perspectives
To appreciate the present, one must look at the past. The journey of scientific facts is storied, often fraught with controversies and varying interpretations. Historically, what was widely accepted as a fact could later be upended. For instance, the Earth was once deemed the center of the universe until Copernicus challenged this notion with his heliocentric theory. The pendulum of understanding swings as new evidence comes to light and old paradigms are challenged.
Moreover, the methods of validating scientific facts have evolved remarkably. The shift from anecdotal evidence to systematic experimentation marks a significant milestone in the history of science. Encouraging a culture of questioning and rigor, scientists over the centuries have learned that skepticism is a powerful tool in uncovering the truths of the universe.
The Evolution of Scientific Understanding
The narrative of how scientific understanding has evolved is both fascinating and essential in grasping the context of scientific facts today. Each discovery feeds into a collective knowledge base that scientists build upon, much like bricks in a wall. With every new fact, each hypothesis tested, previous ideas are reshaped or entirely discarded.
Consider the development of atomic theory. Initially, the concept of the atom was purely philosophical, with the ancient Greeks positing the existence of indivisible particles. Fast forward to the 19th century, and the work of scientists such as John Dalton and later J.J. Thomson and Ernest Rutherford reshaped this understanding substantially, laying the groundwork for modern chemistry and physics.
Today, with advancements in technology, particularly in fields like quantum mechanics and molecular biology, our comprehension of scientific facts is richer and layers deeper than ever before. The dialogue between historical and current perspectives continues, reminding us that the landscape of scientific understanding is always in flux, urging us to adapt and learn.
The Process of Scientific Discovery
The journey of scientific discovery is akin to navigating a vast ocean, where each wave is a new finding, each current a shifting understanding. This section lays out how scientists peel back the layers of our universe to reveal truths about biology, chemistry, physics, and beyond. At its core, the process hinges on a series of meticulous steps that help validate theories and results. Delving into hypothesis formation, experimentation methods, and data analysis provides a roadmap not just for researchers but for anyone intrigued by how knowledge is built.
Hypothesis Formation
Hypothesis formation is the linchpin of scientific discovery. Consider it the starting line, where a question is birthed, often stemming from curiosity or observed anomalies in nature. A scientist’s role transforms from mere observer to an inquisitor, crafting a statement that is not just guesswork but a pathway to exploration.
For instance, if one were to notice that certain plants thrive better in shaded areas, a possible hypothesis could be: "Plants receive sufficient nutrients and grow taller when exposed to indirect sunlight." This statement opens the door to experimentation, allowing researchers to explore various conditions in a controlled environment.
A good hypothesis needs to be testable and falsifiable, which means it can be proven right or wrong through experimentation. This clarity forms the bedrock of the scientific method and ensures that findings contribute durably to the body of knowledge.
Experimentation Methods
Once the hypothesis is set, experimentation methods come into play. These methods form the blueprint for the scientific process, translating theories into tangible actions. The choice of method can vary widely, depending upon the nature of the hypothesis.
- Controlled Experiments: Often favored for their ability to isolate variables. For example, growing two sets of identical plants, one in sunlight and the other in shade, could offer clear insights related to the earlier hypothesis.
- Field Studies: These are more hands-on and often used in ecological or environmental research, where variables cannot be easily controlled. It’s akin to observing behavior in a natural setting, providing a more organic understanding of interactions.
- Computational Simulations: In fields like physics or chemistry, simulating conditions on a computer can yield predictions before tangible experiments are conducted, saving time and resources.
Experimentation is not merely trial and error; it’s a systematic approach to unraveling complexities, producing both data and insights that deepen understanding.
Data Analysis and Interpretation
Finally, after gathering data comes the crucial phase of analysis and interpretation. This is where the raw numbers are synthesized into meaningful information. Data alone is akin to a puzzle with missing pieces. Assembling this data requires tools, though the choice often hinges on the discipline in question.
Advanced statistical techniques are frequently employed in various fields to discern patterns or validate findings. For instance:
- Regression Analysis: This helps determine relationships between variables, crucial for understanding complex interactions in biology.
- ANOVA (Analysis of Variance): Often used when testing multiple groups or treatments to ascertain differences among means.
Once analyzed, interpretation must be approached with a critical eye. What do the findings imply? Are they aligned with existing knowledge, or do they challenge the status quo? Here, scientific skepticism plays a pivotal role, prompting a thorough review of results before final conclusions are drawn.
"In science, it's not enough to just gather data; what we do with it defines the course of inquiry."
Interpretation also requires clear communication of the results, summarizing the findings concisely to inform and engage peer researchers, educators, and the public. A lucid presentation can bridge the gap between scientific jargon and public understanding, paving the way for informed discussions.
Biological Sciences and Their Facts
The biological sciences form a cornerstone of our understanding of life, from the intricate workings of cells to vast ecosystems that sustain every creature on this planet. This section highlights key components such as cell theory, genetic foundations, and ecological relationships, illustrating how biological facts profoundly influence various facets of our existence.
Cell Theory and Its Implications
Cell theory is a foundational concept in biology that articulates the idea that all living organisms are composed of cells. This revelation wasn’t just a passing tidbit; it revolutionized how we perceive life itself. Each organism, whether a single-celled bacterium or a towering redwood, operates at this microscopic level.
Here are some key points about cell theory:
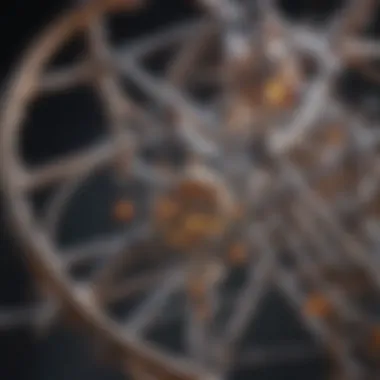
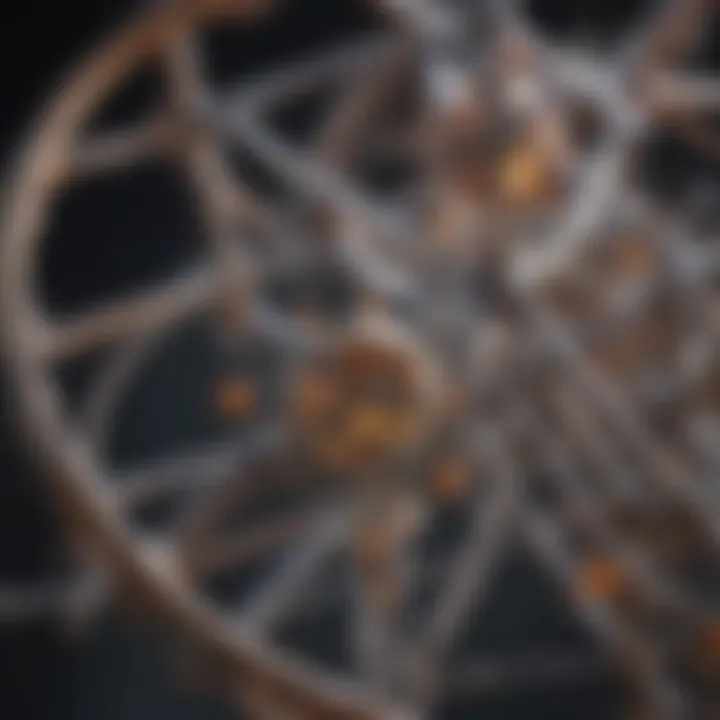
- All living things are made of cells: This principle underscores the unity of life. It highlights how diverse entities share a common structural foundation.
- Cells are the basic units of life: This tells us that all biological processes occur within the confines of a cell, leading to a better understanding of diseases and treatments.
- All cells come from pre-existing cells: This implies that life is self-perpetuating, relying on the replication of cells, which is crucial for growth and healing.
The implications of cell theory extend beyond mere classification; it informs research in fields like medicine and genetics. Understanding cellular processes aids in developing treatments for diseases. When we comprehend how cells interact, we can design therapies to target specific issues, such as cancerous growths or degenerative conditions.
"The cell is the basic unit of structure and function in all living organisms." — A fundamental truth reflecting the essence of biological sciences.
Genetic Foundations
Genetics is an exciting area of biology focused on heredity and variation in organisms. It explains how traits get passed down through generations. With the discovery of DNA, our understanding of biology took a giant leap forward. DNA is the blueprint for life, dictating everything from physical characteristics to predisposition to certain diseases.
Some significant aspects include:
- Understanding hereditary disorders: Genetic research sheds light on conditions like cystic fibrosis or hemophilia, aiding in early diagnosis and potential treatment pathways.
- Genetic engineering: This enables manipulation of genetic material to enhance crop yields or create medical advances through targeted therapies.
- The Human Genome Project: It was a monumental step that mapped all genes in the human DNA, serving as a reference for numerous studies and medical breakthroughs.
This genetic foundation affects not just biology but intersects with ethics as it raises questions about gene editing and the socio-economic implications of access to genetic therapies.
Ecological Relationships
Ecology, another key component of biological sciences, studies how organisms interact with each other and their environments. Understanding these relationships allows us to grasp the intricacies of ecosystems and the life cycles that sustain them.
Key elements of ecological relationships to consider include:
- Food chains and webs: These complex networks illustrate how energy transfers from one organism to another. They show how essential each species is to maintain ecosystem balance.
- Symbiotic relationships: Various forms such as mutualism, parasitism, and commensalism highlight the interdependence of species, demonstrating that survival often hinges on collaboration or competition.
- Conservation efforts: Understanding ecological interactions is critical in efforts to preserve biodiversity and address climate change. Initiatives aimed at conserving endangered species depend heavily on ecological insights.
What differentiates biological sciences from other fields is their focus on the interconnectedness of life forms, and how they adapt in response to their environments.
In sum, biological sciences and their key facts are not mere academic concepts; they hold the power to inform practices that impact health, environment, and even societal structures, making them incredibly relevant in today’s fast-changing world.
Fundamental Concepts in Chemistry
Understanding chemistry is crucial as it opens a window into the very fabric of our universe. In its essence, chemistry provides insights into how matter interacts, which is fundamental when we dissect larger scientific discussions. By examining the basic principles of chemistry, we grasp the mechanisms that govern everything from the simplest life forms to complex technological innovations. This section takes a close look at atomic structure, chemical reactions, and thermodynamics, which are the cornerstones of this science.
Atomic Structure and the Periodic Table
Every substance in the universe is made up of atoms, the tiniest functional units of matter. The structure of an atom is composed of protons, neutrons, and electrons. The arrangement of these particles determines the characteristics and behavior of elements. For instance, atomic number—the count of protons—defines the element itself, which is neatly organized in the periodic table.
The periodic table isn’t just a chart; it’s a storybook of elements, revealing patterns that illustrate how elements behave chemically. Each column, or group, often reacts similarly because they have the same number of electrons in their outer shell. Understanding this framework is not only critical for students and researchers but also helps professionals make sense of chemical properties and predict reactions efficiently.
"The periodic table is the most important tool for chemists; it summarizes the relationships between elements and their chemical behavior."
Chemical Reactions and Equations
Chemical reactions form the crux of chemistry, representing the transformation from reactants to products through various interactions. Each reaction can be expressed using chemical equations, which communicate what happens at a molecular level. For example, when hydrogen gas combines with oxygen gas, it forms water—H₂ + O₂ → 2H₂O.
This equation doesn’t just illustrate a simple combination; it shows us the conservation of mass principle where atoms are neither created nor destroyed, merely rearranged. This principle informs every scientific discipline and enhances our understanding of environmental challenges. Accurate chemical equations allow scientists to predict results, scale up reactions for industrial applications, and even inform environmental policies.
Thermodynamics Principles
Thermodynamics is a branch of chemistry that deals with heat and temperature and their relation to energy and work. Principles like the laws of thermodynamics guide scientists in understanding energetic changes occurring during reactions. For instance, the first law expresses that energy cannot be created or destroyed, only converted, underscoring everything from engine efficiency to living organism metabolisms.
Moreover, the second law introduces concepts like entropy, illustrating the tendency for systems to move toward disorder. Grasping thermodynamic principles is vital for professionals in engineering and environmental sciences, as they seek to optimize energy use and minimize waste.
Physics: The Fundamental Laws
Physics lays the groundwork for understanding the universe; it's the study that explains how matter and energy interact. Delving into the fundamental laws of physics reveals not just theoretical concepts but also practical applications that govern our everyday existence. Knowledge of these laws assists students, researchers, educators, and professionals in comprehending natural phenomena while inspiring interdisciplinary studies across science and technology fields.
The vital aspects of physics encompass:
- Predictive Power: Physics allows us to predict natural occurrences, from the direction of a car's motion to the trajectory of celestial bodies.
- Technological Applications: Innovations in technology, such as MRI imaging and semiconductors, originate from principles of physics.
- Interconnectedness: Understanding physics encourages exploration beyond the confines of specific disciplines. For instance, in medicine, physics helps develop techniques utilized in diagnostic imaging.
Each of the three sections—Newton's Laws of Motion, the Theory of Relativity, and Quantum Mechanics—further enhances our comprehension of these foundational principles.
Newton's Laws of Motion
Newton's Laws of Motion are cornerstones of classical mechanics, providing essential insight into the movement of objects. Essentially, they encapsulate:
- First Law (Inertia): A body remains at rest or in uniform motion unless acted upon by a net external force. This law underpins many daily experiences; for example, a child on a skateboard will not stop moving until friction, or another force, brings them to rest.
- Second Law (F=ma): The acceleration of an object is depending on its mass and the net force acting on it. This relationship is encapsulated in the equation F=ma, where force equals mass times acceleration. This understanding helps professionals in fields ranging from engineering to aerodynamics.
- Third Law (Action-Reaction): For every action, there is an equal and opposite reaction. This principle explains phenomena such as rocket propulsion, where the expulsion of gas results in forward motion of the rocket.
The implications of Newton's Laws extend well beyond physics textbooks. They form a basis for many technologies, from vehicles to amusement park rides, delivering real-world applications.
The Theory of Relativity
Einstein’s Theory of Relativity fundamentally shifted our understanding of time and space. Comprising two parts—Special and General Relativity—it delineates:
- Special Relativity: Introduced the idea that the laws of physics remain constant across inertial frames and posited that the speed of light is universally constant. This inquiries into how time behaves differently for observers moving at different speeds—a calculation with profound implications for technologies such as GPS, where adjustments must accommodate relativistic effects.
- General Relativity: An extension discussing gravity as a curvature in spacetime caused by mass. Understanding general relativity is crucial for advancements in astrophysics, including black hole physics and cosmology.
Einstein succinctly illustrated this principle:
"Space and time are the frameworks within which the events of the universe occur."
This framework influences various modern physics branches significantly, including cosmology and astrophysics.
Quantum Mechanics Overview
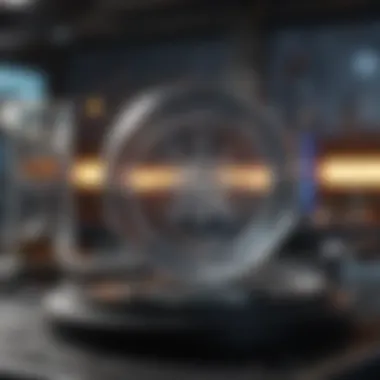
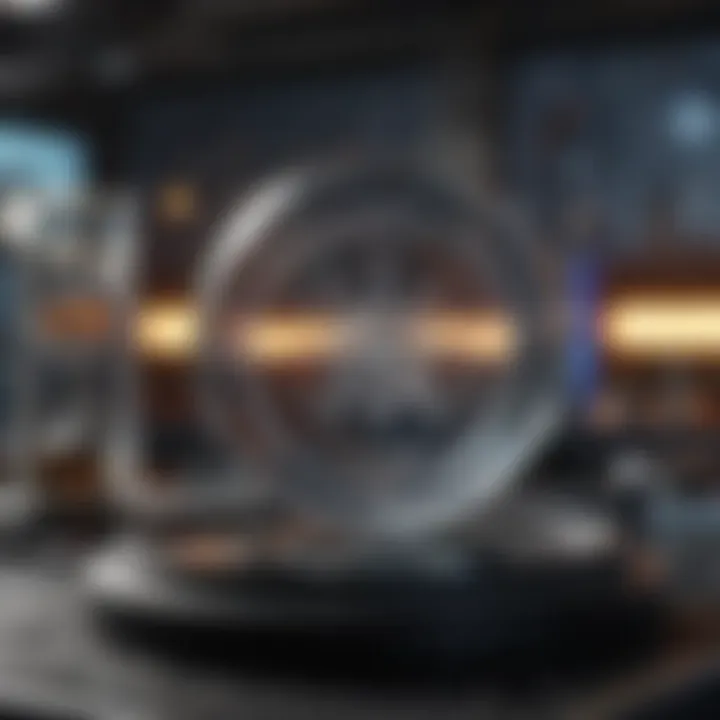
Quantum Mechanics introduces a realm that starkly contrasts classical physics knowledge. It grapples with the behavior of matter and energy at extremely minute scales, influencing many aspects of today’s science and technology. Key elements include:
- Wave-Particle Duality: Particles, such as photons, exhibit properties of both waves and particles, complicating our understanding of matter.
- Uncertainty Principle: Articulated by Heisenberg, this principle states that one cannot simultaneously know both the position and momentum of a particle with absolute certainty. This has profound ramifications for the nature of reality and observation in quantum experiments.
- Superposition and Entanglement: Quantum states can exist in multiple conditions until observed, and entangled particles remain interconnected regardless of distance, leading to philosophical inquiries about locality and reality.
Everyday technologies, including semiconductors and lasers, derive from these quantum principles. By recognizing the fundamental laws of physics, we gain insights that are crucial in navigating our increasingly complex technological landscape.
Earth Sciences and Their Importance
The Earth sciences encompass a broad array of disciplines that probe into the workings of our planet, from its majestic landscapes to its complex atmospheric systems. Understanding Earth sciences is crucial not only for appreciating Earth's natural beauty but also for grasping the interconnectedness of geological, atmospheric, and ecological processes. These sciences equip us with essential knowledge that underpins environmental management, resource allocation, and disaster preparedness. Moreover, they serve as a foundation for informed policy-making in addressing urgent issues like climate change and sustainable living.
Geological Principles
Geological principles form the bedrock of Earth sciences, allowing us to explore the composition, structure, and processes that shape our planet. By studying the Earth's layers, rocks, and minerals, we gain insight into phenomena such as earthquakes, volcanoes, and the formation of mountains. For instance, the theory of plate tectonics explains how the Earth's lithosphere is divided into tectonic plates that move over the semi-fluid asthenosphere.
- Natural Resources: This understanding helps in locating natural resources, including fossil fuels and minerals, vital for our energy needs.
- Risk Assessment: It also aids in assessing natural hazards, enabling communities to prepare for and mitigate the impacts of disasters.
In essence, geological principles offer a lens through which we can see the dynamic history of our planet, providing valuable information crucial for both everyday life and scientific inquiry.
Atmospheric Studies
Atmospheric studies delve into the properties and behaviors of the gases surrounding the Earth. The atmosphere is a delicate balance of elements, and even slight shifts can lead to widespread consequences. Analyzing atmospheric patterns helps us understand weather systems and climate changes.
- Weather Prediction: Meteorologists rely on advanced tools and techniques to forecast weather, impacting agriculture, travel, and emergency management.
- Climate Science: Research in this field also tackles climate change, enabling scientists to track shifts over time and predict future trends. Such insights are critical for shaping policies and actions to curb greenhouse gas emissions.
Understanding atmospheric studies equips society to adapt to challenges posed by climate variability and promotes a more resilent approach to our daily lives.
Ecological Sustainability
As we understand both geological and atmospheric principles, we are compelled to address ecological sustainability. This aspect focuses on the relationships between living organisms and their environments, stressing the need for balance for the well-being of our planet.
- Conservation Efforts: Conservation biology informs methods to protect endangered species and habitats, ensuring biodiversity is preserved for future generations.
- Resource Management: Sustainable practices in farming, forestry, and fisheries aim to minimize depletion of natural resources, acknowledging the importance of ecosystems.
"Sustainability is not just a buzzword; it's a commitment to a future where both humanity and nature thrive."
The insights derived from ecological studies empower communities to adopt practices that protect their environments, thus fostering a sustainable future.
The End
In summary, the Earth sciences are pivotal in understanding the complexity of our planet. From geological principles that reveal Earth's structure to atmospheric studies that inform on climate conditions and ecological sustainability that advocates for a balanced existence, each aspect plays a role in crafting informed citizens and responsible guardians of our environment. It's clear that understanding these principles transcends academic curiosity; it's about taking actionable steps toward a sustainable future.
The Role of Technology in Science
In the modern landscape of scientific inquiry, technology serves as the bedrock supporting numerous advancements and breakthroughs. It’s more than just a tool; it’s a catalyst for innovation, shaping the way researchers gather data, conduct experiments, and interact with their findings. As we dissect this relationship, it’s key to recognize the 'how' and 'why' behind technology’s importance in science:
- Enhanced Accuracy: Technology ensures that data collection methods yield reliable and precise results, reducing human error. In fields like astronomy or molecular biology, where minute details can influence outcomes, the difference can be astronomical.
- Increased Efficiency: Techniques such as automation and high-throughput screening allow larger datasets to be analyzed quicker than ever. This not only saves time but also drives projects that utilize vast amounts of data, such as genomic studies.
- Interdisciplinary Collaboration: Modern technological tools foster cooperation across disciplines. Scientists from varied backgrounds can utilize shared platforms for real-time data exchange, enriching research with diverse perspectives.
Instrumentation and Techniques
Instrumentation has evolved remarkably, changing the landscape of scientific research. Take, for example, the rise of CRISPR-Cas9 technology in genetics. This tool has transformed genetic engineering by allowing more precise edits to DNA. Researchers can now seamlessly tweak genes, leading to advances in medicine and agriculture. Beyond genetics, instrumentation such as mass spectrometers and electron microscopes give scientists the ability to analyze substances at unprecedented levels.
For instance:
- Mass spectrometers: These allow for the determination of molecular weights and structures, crucial in both environmental studies and drug development.
- Electron microscopes: They reveal structures at atomic levels, fortifying the foundation of materials science.
Data Management Systems
As scientific research shifts toward big data, the management of information becomes paramount. Robust data management systems provide a framework that aids in organizing, storing, and retrieving data efficiently. The benefits of these systems include:
- Data Integrity: By maintaining a centralized repository, the risk of data loss or manipulation declines.
- Collaboration: Teams can streamline their efforts, enabling multiple researchers to work on a single dataset without redundancy, enhancing teamwork.
- Accessibility: Proper management ensures that data is accessible to those who need it, whether they’re directly involved in the research or part of the public interested in scientific findings.
Furthermore, technologies such as cloud computing and blockchain are paving the way for enhanced transparency and security. Projects are no longer hindered by storage capabilities or complex sharing mechanisms.
Innovations in Scientific Research
Innovations fueled by technology are reshaping the scientific landscape, fundamentally changing research methodologies and opening up new realms of possibility. Some notable advancements include virtual simulations, artificial intelligence in data analysis, and lab-on-a-chip technologies. These innovations lead to:
- Faster Results: Researchers can simulate hypotheses to see potential outcomes before conducting real-world tests, saving time and resources.
- Deeper Insights: AI-driven algorithms can analyze complex datasets, revealing trends and correlations that manual analysis might miss.
- Enhanced Accessibility: Miniaturized labs can provide essential capabilities in remote areas or low-resource settings.
"In science, technological innovations work hand-in-hand with theories and methodologies, making the quest for knowledge more effective and transformative."
The intertwined nature of technology and science signifies a promising horizon for forthcoming explorations, pushing the boundaries of our understanding. As we embrace these changing tools, it’s important to remain critical, ensuring that they serve to enhance our comprehension of the universe, rather than cloud it.
Communicating Scientific Knowledge
Effective communication of scientific knowledge is crucial in today’s fast-paced world. It also acts as the bridge linking complex scientific ideas with the general public. When scientists articulate their findings clearly, they ensure that their invaluable research reaches a wider audience, which in turn fosters a more scientifically literate society.
One key element of this process is the peer review system. This system is fundamentally designed to uphold the integrity of science by ensuring that research is evaluated by experts before publication. It acts as a filter, guaranteeing that only work meeting rigorous standards makes it into academic journals. However, it is not without its challenges. For instance, the peer review process can sometimes be slow, delaying the availability of groundbreaking information. Additionally, it is not infallible—cases of bias and sometimes even corruption have been reported. Therefore, while vital, ongoing scrutiny of this process is necessary to maintain its effectiveness.
Another indispensable aspect is publications and scientific journals. These publications serve as repositories of knowledge, allowing researchers to share their insights and findings. They encompass a variety of formats, from interdisciplinary journals to specialized ones, catering to specific fields. The challenge lies in making sure that this wealth of information is accessible. Many journals are behind paywalls, complicating access for interested parties. The recent rise of open-access journals attempts to remedy this, but the effectiveness of this model is still being debated.
Moreover, science education and outreach play a critical role in cultivating a knowledgeable populace. Initiatives aimed at demystifying science, such as community workshops or engaging multimedia content, can make a huge difference. Schools and universities foster the next generation of scientists while also sparking interest in non-specialists. The goal here should be to generate enthusiasm and understanding, rather than mere rote memorization of facts.
“In science, communication isn’t just about presenting knowledge; it’s about inspiring curiosity.”
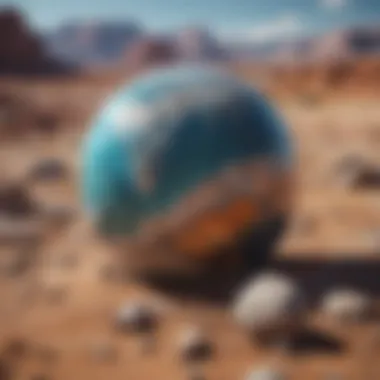
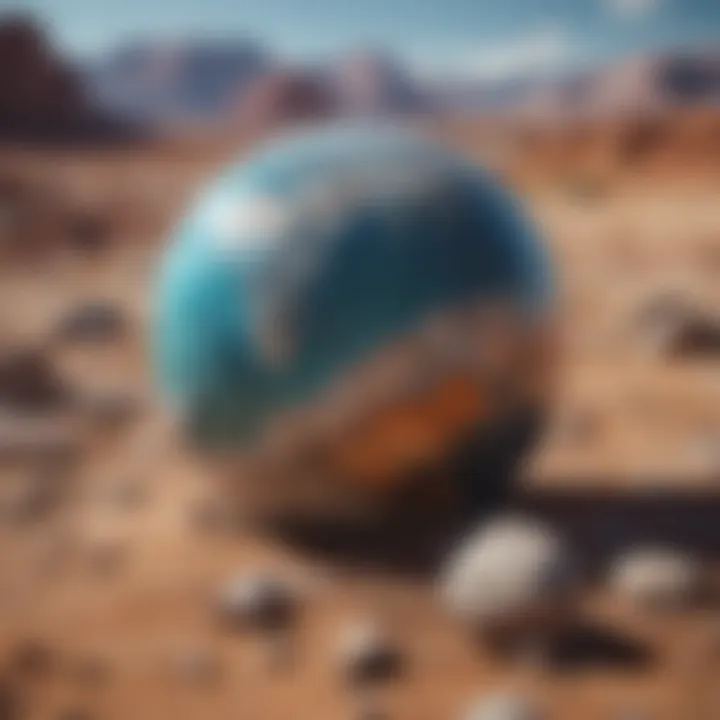
To summarize, the channels through which scientific knowledge is communicated—namely, peer review processes, publications, and educational outreach—are vital for the health of the scientific community. Together, they ensure that knowledge circulates effectively and reaches those who can benefit from it, thereby laying the foundation for an informed public capable of engaging critically with scientific issues.
Challenges in Scientific Research
Understanding the challenges in scientific research is crucial for grasping how knowledge is constructed and validated over time. Despite the seeming clarity that scientific facts provide, several hurdles can convolute the path from inquiry to conclusion. Researchers often find themselves navigating complex webs of reproducibility, ethics, and information access, each of these factors influencing not just scientific output but also public trust in science as a whole.
Reproducibility Crisis
The reproducibility crisis in scientific research refers to the increasing realization that many studies cannot be replicated successfully. This is no small potatoes. When findings can’t be consistently reproduced, it undermines the entire foundation of the scientific method, which hinges on repetition and verification. Various factors contribute to this crisis:
- Poor study design: Some researchers may overlook essential methodological rigors, resulting in flawed experiments.
- Publication bias: Journals tend to favor positive results, often sidelining studies that produce negative or inconclusive findings. This skews the overall perception of scientific validity.
- Statistical issues: Misapplication of statistical methods can lead to false positives or misinterpretations of data.
As researchers and institutions grapple with these challenges, efforts are being made to improve transparency within the research community. For example, pre-registration of studies aims to set a defined hypothesis and methods before data collection. This move could potentially mitigate the issue of cherry-picking data to fit desired outcomes.
"Reproducibility isn't merely a checkbox in the research process; it's the linchpin of scientific credibility."
Ethics in Science
Ethics in scientific research weave through every thread of the scientific fabric. While striving to elucidate and innovate, researchers must be constantly aware of the moral implications their work entails. Ethical considerations can include:
- Human and animal welfare: Any study involving living subjects must adhere to strict ethical guidelines to ensure their safety and dignity.
- Data integrity: Researchers must avoid fabrication or falsification of data, as slipping down this slippery slope can incur damage not only to individuals but to the credibility of science as a whole.
- Conflict of interest: Transparency about funding sources and potential biases is necessary to sustain public trust in scientific findings.
Ethics is not just about avoiding wrongdoing; it also involves actively promoting veracity and social responsibility in scientific endeavors. As the landscape evolves, discussions around ethics become ever more paramount, especially in areas like genetic engineering or AI research where the stakes are high.
Access to Information
Access to information remains a contentious issue in the realm of scientific research. Knowledge is power, as they say, yet barriers to access can stifle innovation and limit the reproducibility of research. Key elements at play include:
- Paywalls for academic journals: Many scholars and students lack the resources to access pivotal research papers, narrowing their horizons and understanding. This situation fosters a divide between well-funded institutions and those with lesser means.
- Open access initiatives: These aim to democratize knowledge by allowing public access to peer-reviewed research. While progress has been made, challenges such as quality control and sustainability remain pertinent to the conversation.
- Fragmented data sources: Multiple databases housing scientific information can complicate efforts to gather comprehensive data, making literature reviews a Herculean task.
Bridging these gaps in information access could unleash a torrent of innovation and collaboration. While some advancements have been made, ongoing dialogue around these issues is essential to ensure equal opportunity in scientific research.
In essence, the landscape of scientific research comes with its fair share of bumps and hurdles. By addressing the challenges of reproducibility, ethics, and access to information head-on, the scientific community can work towards not only enhancing its credibility but also fostering an environment that values transparency and collaboration.
Future Directions in Scientific Inquiry
The landscape of scientific research is constantly evolving, much like the universe it seeks to understand. Mapping future directions in scientific inquiry sheds light on emerging trends and methodologies that will define the way we approach science in the coming years. By recognizing the interplay between disciplines, the urgent need to address climate change, and the rapid advancements in technology, we can appreciate how they shape our understanding of the world. This section underscores the significance of adapting our methods and perspectives to foster innovation while maintaining scientific rigor.
Interdisciplinary Research Approaches
There's a saying that no man is an island, and the same can be said for scientific research. In recent years, there's been a noticeable shift towards interdisciplinary approaches. Think about it—complex problems don’t fit neatly into one box. For instance, how can we tackle issues like health pandemics or ecological crises effectively without drawing on multiple scientific fields? The blend of biology, chemistry, physics, and social sciences allows for a more holistic understanding.
Researchers are increasingly forming partnerships across disciplines, creating a melting pot of ideas and insights. This method not only maximizes knowledge but also fosters creativity. By working together, experts can combine techniques and theories, leading to significant breakthroughs. Take CRISPR technology; its origins stem from microbiology but gain insights from genetics, engineering, and even ethics. Consequently, interdisciplinary teamwork is becoming imperative in creating solutions that are impactful and sustainable.
Climate Change Research
Climate change is possibly the most pressing issue of our time. Acknowledge it or not, its implications permeate discussions in various scientific realms. The future direction of climate change research must be a priority, as we face dire consequences if action is delayed. Researchers are shifting focus to not just understand the mechanisms, but also to predict outcomes and develop mitigation strategies.
This pursuit is like navigating through foggy waters; the stakes are incredibly high, and clarity is essential. Scientists utilize sophisticated models and simulations, engaging fields like meteorology, oceanography, and environmental science. The goal here is not just to build a body of knowledge, but to translate findings into actionable strategies. In simpler terms, it’s about turning academic theories into practical solutions that can be adopted globally.
"Without the awareness of climate change, our research could easily become a futile effort in the face of impending crisis."
The collaboration between politics, sociology, and science is vital in this endeavor, ensuring that policies resonate with the scientific evidence available.
Technological Advancements Impacting Science
In a world that's powered by technology, the relationship between scientific inquiry and technological advancement cannot be overstated. From artificial intelligence to advanced imaging techniques, technology is the wind beneath the wings of modern science.
For example, machine learning is revolutionizing how researchers process data. Instead of sifting through mountains of numbers, algorithms can indicate patterns and trends faster than a human ever could. Moreover, tools like CRISPR and next-generation sequencing have opened new doors in genetics and molecular biology, accelerating discovery at a staggering pace.
However, with great power comes great responsibility. Ethical considerations must accompany these advancements. The dialogue around data privacy, access, and even ecological impact of technology should be ongoing. Researchers and technologists must work hand-in-hand to ensure that innovations align with the values and needs of society.
Culmination: The Significance of Scientific Facts
As we wrap up our exploration of scientific facts, it's vital to reflect on their significance in shaping our understanding of the universe. Scientific facts serve as the backbone of rational thought, informing not just the scientific community but society as a whole. They pave the way for innovation, drive policy decisions, and impact everyday lives. To have a firm grasp of these facts is to wield the power of informed decision-making, whether it concerns health, environment, or technology.
When we acknowledge the weight of scientific facts, we recognize that they go beyond isolated data points; they build a complex web of interrelationships which enhance our comprehension of the world around us. Such an understanding is crucial, particularly in an age rife with misinformation and sweeping claims that often lack a solid foundation in research.
"Science must not be allowed to become a matter of opinion." - Mark Twain
Critical Thinking and Scientific Literacy
Critical thinking is an essential skill, one that empowers individuals to dissect information effectively. It involves analyzing facts, assessing their validity, weighing evidence, and drawing reasoned conclusions. This skill is more important than ever in today’s fast-paced information age, where claims can spread like wildfire, often leading to confusion or misguided beliefs.
Scientific literacy complements critical thinking; together, they give individuals the tools they need to engage with scientific information constructively. A scientifically literate person can communicate effectively about scientific topics, recognize credible sources, and understand the implications of scientific developments.
Being scientifically literate contributes to various societal needs, including:
- Health Literacy: Understanding medical research and recommendations can guide personal health choices.
- Environmental Awareness: A sound grasp of ecology fosters sustainable practices.
- Technological Adaptability: Awareness of advancements and their societal impacts helps people embrace technological changes.
The Responsibility of Scientists and Educators
Scientists and educators hold a unique position in fostering a culture of scientific facts and critical thinking. As guardians of knowledge, they must act responsibly, ensuring that research is conducted ethically and findings are communicated accurately.
The responsibility of scientists lies not merely in conducting experiments but also in translating complex concepts into understandable ideas for the layperson. They need to engage with the public to demystify science, thus building trust and understanding.
Educators, on the other hand, play a key role in laying down the foundation of scientific literacy. By teaching students how to think critically and apply scientific methods, they prepare the next generation to navigate a world increasingly driven by technology and science. Additionally, they should encourage curiosity and skepticism, guiding students to ask questions and seek evidence before forming beliefs.
Together, scientists and educators can shape a more informed society where scientific facts are seen not just as static data but as dynamic pieces of a larger puzzle—critical to our understanding of the complexities of life and the universe.