Exploring Pharmacodynamics Biomarkers in Drug Therapy
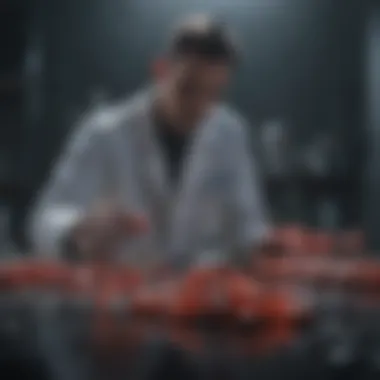
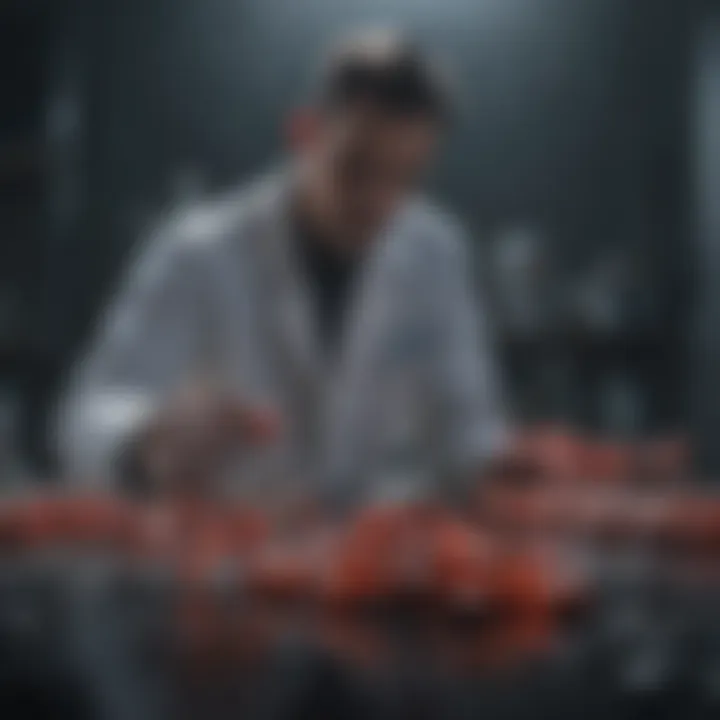
Intro
The world of pharmacodynamics biomarkers is a realm where science meets the intricate dance of drug interactions within the human body. As we navigate through this extensive topic, it's essential to grasp what these biomarkers signify for drug efficacy, safety, and the tailoring of personalized medicine.
Biomarkers represent measurable indicators that reveal the effects of a drug and the physiological state of an organism. Understanding these elements paves the way for improved therapeutic outcomes. It's akin to having a road map; it guides physicians and researchers through the complex pathways of drug mechanisms. The implications canโt be overstated: these indicators not only inform clinical decisions but also foster innovation within pharmaceutical research.
As we embark on this exploration, we will dissect various facets of pharmacodynamics biomarkers, ranging from the methodologies involved in their identification and validation to the cutting-edge technologies that aid in their measurement. By the end of this narrative, readers will appreciate not just the importance of pharmacodynamics biomarkers but also the challenges that lie ahead in their usage.
Through this comprehensive guide, we aim to illuminate the path toward enhancing drug interactions and therapeutic effectiveness. Buckle up, as we delve deep into this subject.
Prolusion to Pharmacodynamics
Pharmacodynamics sits at the heart of how we understand the interplay between drugs and the biological systems they affect. By examining pharmacodynamics, researchers and healthcare professionals can decipher the effects of a drug on the body, the mechanisms of action, and the interplay of dosage and response. This understanding is vital not just for scientists in laboratories, but also for clinicians seeking to deliver optimal care to patients.
Definition and Scope
At its core, pharmacodynamics is defined as the study of the biochemical and physiological effects of drugs on the body. It delves into what a drug does to the body and how it achieves its therapeutic effects. This discipline encompasses everything from the interaction of drugs with their receptors to the diverse physiological responses triggered by these interactions.
The scope of pharmacodynamics extends far beyond a simple overview of drug effects; it includes:
- Quantitative Analysis: Measurement of drug effectiveness and potency.
- Mechanistic Insights: Understanding how drugs elicit physiological changes.
- Clinical Relevance: Application of pharmacodynamic principles in clinical settings to improve therapeutic outcomes.
In a broader context, pharmacodynamics is essential for the optimization of drug therapy, ensuring that each patient's treatment is both effective and tailored to individual needs.
Importance in Drug Development
The role of pharmacodynamics in drug development cannot be overstated. From initial discovery stages through to clinical trials, understanding how drugs interact with their targets informs a multitude of critical decisions. Firstly, pharmacodynamics helps in identifying the most promising candidates during the early phases of drug development, as researchers can prioritize compounds with favorable efficacy profiles.
Moreover, it plays a crucial part in dose selection, guiding what dosages are likely to yield desired effects while minimizing adverse reactions. This understanding is especially pivotal in critical areas like oncology, where the therapeutic window can be narrow, and the margin for error slim.
Furthermore, pharmacodynamics aids in refining treatment regimens by providing insights into:
- Patient Variability: Recognizing how genetic and environmental factors influence drug action.
- Combination Therapy: Understanding how multiple drugs can be combined effectively for synergistic effects.
- Resistance Mechanisms: Identifying how and why certain patients develop resistance to therapies, thereby driving the pursuit of novel interventions.
In sum, a solid grasp of pharmacodynamics not only enriches our comprehension of drug action but also facilitates the rational design and development of safer and more effective therapeutic agents. It underscores the commitment to achieving efficacy in real-world clinical settings, making it an indispensable component in the arsenal against disease.
"Understanding pharmacodynamics is pivotal for crafting treatments that resonate with patient needs, balancing efficacy and safety delicately."
With adequate insights into pharmacodynamics, researchers and healthcare providers can ensure that the ever-evolving landscape of pharmaceuticals is navigated with precision and acumen.
Understanding Biomarkers
When it comes to navigating the labyrinth of pharmacodynamics, biomarkers emerge as focal points that guide our understanding of how drugs affect the body. These indicators serve as bridges between the complexities of biological responses and the clinical effects of medications. By grasping the concept of biomarkers, one can better appreciate their vital role in drug development and therapy, as well as their broader implications in personalized medicine.
Definition of Biomarkers
Biomarkers, in simplest terms, are measurable indicators of biological processes. They can be molecules in blood, bodily fluids, or tissues that reflect the presence of a disease or the effectiveness of a treatment. Think of them as signposts; they help both researchers and clinicians track therapeutic outcomes and make informed decisions. In the realm of pharmacodynamics, biomarkers shine a light on the interactions between drugs and their targets, offering insights into efficacy and safety.
Types of Biomarkers
Understanding biomarkers involves delving into various types, each serving distinct roles within the clinical landscape. These types include diagnostic, prognostic, and predictive biomarkers.
-#### Diagnostic Biomarkers
Diagnostic biomarkers are crucial tools in identifying diseases. Their primary function is to indicate the presence of a condition, often before symptoms even manifest. A notable example would be the use of prostate-specific antigen (PSA) levels to diagnose prostate cancer. This biomarker is advantageous because it can signal the need for further investigation, leading to early intervention. However, its specificity might come into question, as elevated levels may also arise from benign conditions. Therefore, while they are essential for initial disease detection, caution should be exercised regarding over-reliance solely on these indicators.
-#### Prognostic Biomarkers
Prognostic biomarkers are the fortune tellers of the disease world. They provide information on the likely course of a disease or the chances of recovery. For instance, certain genetic markers can predict the aggressiveness of cancers, helping tailor patient management plans. This foresight can indeed steer treatment choices, whether towards aggressive therapies or more conservative approaches. Nevertheless, they also come with complexities, as individual variations can sometimes shift prognostic outcomes.
-#### Predictive Biomarkers
Predictive biomarkers look into the future by indicating how a patient will respond to a specific therapy. A classic case is the HER2/neu marker in breast cancer, which helps determine whether patients will benefit from trastuzumab (Herceptin) treatment. This targeted approach enhances treatment efficacy and minimizes unnecessary side effects. Yet, false positives can occur, leading patients to undergo treatments that may not work.
Role of Biomarkers in Drug Therapy
In drug therapy, biomarkers play a pivotal role by integrating clinical and molecular data. They facilitate a more informed approach to medication, ensuring that treatments are tailored to individual patient needs. This customization, or precision medicine, not only enhances efficacy but also reduces the likelihood of adverse reactions. By understanding how a drug interacts with its target biomarker, clinicians can optimize dosing regimens and improve outcomes.
"The use of biomarkers in drug therapy is not merely a trend; it is a fundamental shift toward more personalized, effective healthcare."
Through ongoing research, new biomarkers will continue to emerge, reshaping our understanding of pharmacodynamics and paving the way for innovations in drug discovery and therapy. As the field advances, the integration of biomarkers into routine practice is expected to enhance patient outcomes and refine therapeutic strategies.
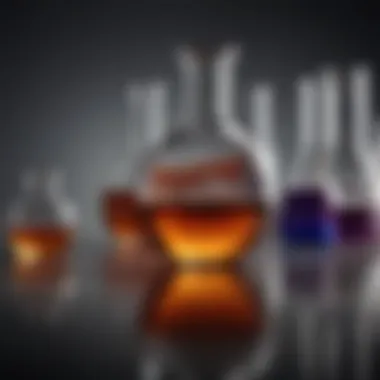
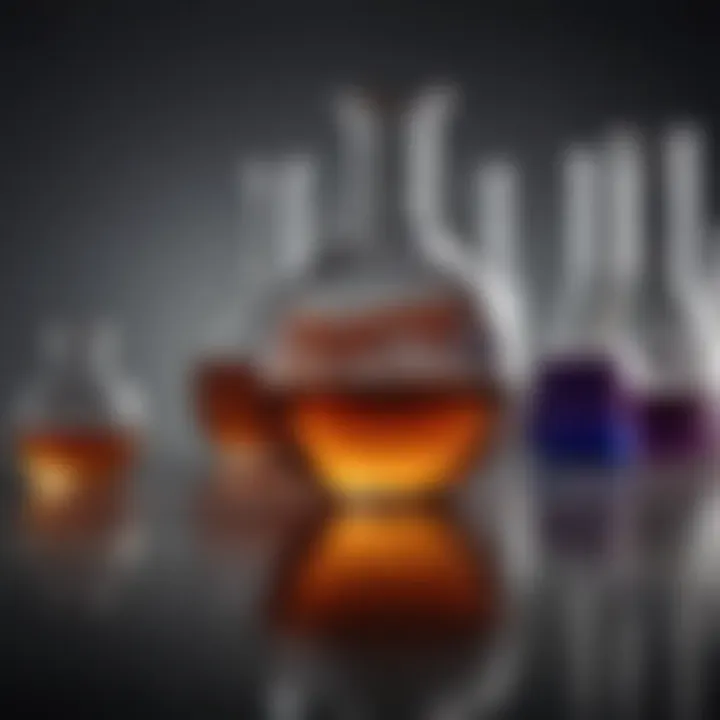
Mechanism of Pharmacodynamics
Understanding the mechanism of pharmacodynamics is pivotal in grasping how drugs exert their effects within the body. This involves a detailed examination of how drugs interact with their targets, and this understanding sets the stage for designing effective therapeutic strategies. Factors such as receptor binding, efficiency, and duration of action are crucial when evaluating a drug's pharmacodynamic profile. By exploring these mechanisms, researchers and practitioners can enhance the precision of drug therapy, thereby improving patient outcomes.
Drug-Receptor Interactions
At the heart of pharmacodynamics lies the concept of drug-receptor interactions. Simply put, drugs work by binding to specific receptors on cells, initiating a cascade of biological effects. The nature of this interaction can vary significantly; some drugs are designed to activate the receptor (agonists), while others block them (antagonists). The affinity of a drug for its receptor plays a key role in determining its efficacy.
- Agonists: These substances mimic the action of natural signaling molecules. For instance, morphine acts as an agonist on mu-opioid receptors, providing pain relief by enhancing pain inhibition signals.
- Antagonists: On the other hand, drugs like naloxone displace agonist binding, blocking the effects of opioids, which is vital in overdose situations.
The selectivity of these interactions is critical. A highly selective drug may target a specific receptor subtype and minimize side effects, while a less selective agent can impact multiple pathways, leading to a broader range of effectsโboth therapeutic and adverse.
"Understanding how a drug interacts with its receptor can illuminate both its potential effectiveness and its risks."
Dose-Response Relationship
The dose-response relationship is another foundational pillar in pharmacodynamics. It describes how the magnitude of the drug effect correlates with its concentration at the site of action. Essentially, it answers a fundamental question: How much drug is needed to achieve the desired effect?
This relationship can be characterized graphically in a dose-response curve, where the x-axis represents the dose and the y-axis shows the response. Here are some key points to keep in mind:
- Threshold Dose: The minimum amount of a drug needed to produce an observable effect.
- Maximal Effect (Emax): The highest effect achievable by a drug regardless of dose.
- Potency: A measure of how much drug is needed to produce a given effect; a more potent drug will produce a response at lower concentrations.
Variability in this relationship can arise due to differences in patient biology, including genetics, age, and existing health conditions. This variability is why understanding pharmacodynamics is essentialโtailoring dosage can optimize therapeutic outcomes, effectively personalizing treatment plans.
Time Course of Drug Action
The time course of drug action delves into when a drug begins to take effect and how long those effects last. This is integral to treatment regimens, as it informs both the timing and frequency of dosing.
Key terms associated with this characteristic include:
- Onset of Action: The time it takes for a drug to start having its effect.
- Duration of Action: How long the drug's effects persist.
- Half-Life: The time required for the concentration of the drug in the bloodstream to reduce by half.
Understanding these time dynamics can ultimately influence clinical decisions. For instance, rapid-onset medications like adenosine are vital in acute cardiology settings, while others with longer activities might be chosen for chronic conditions, ensuring continuous therapeutic coverage.
In summary, the mechanisms of pharmacodynamics encompass a variety of processesโincluding receptor interactions, dose-response relationships, and the temporal aspects of drug actionโeach critical for accurate and effective drug therapies. By systematically unraveling these mechanisms, the healthcare community can align treatments more closely with the individual needs of patients.
Identifying Pharmacodynamics Biomarkers
Identifying pharmacodynamics biomarkers is pivotal in the contemporary landscape of drug development and therapeutic efficacy. These biomarkers provide vital insights into how drugs interact within the body, thereby influencing their effectiveness and safety profiles. Understanding this aspect ensures that treatments are not only appropriately dosed but tailored to individual responses, maximizing benefits while minimizing adverse effects. With the growing emphasis on personalized medicine, the precise identification of biomarkers becomes even more relevant. By utilizing specific and robust selection criteria, researchers can identify markers that correlate strongly with drug response, ultimately enhancing both clinical outcomes and the drug development journey.
Selection Criteria for Biomarkers
When choosing biomarkers, several critical factors must be considered to ensure their relevance and reliability. Here are some selection criteria:
- Specificity: A good biomarker should distinctly indicate a particular condition or biological process and not overlap with other markers.
- Sensitivity: It should reliably detect the presence of a drug response across various populations.
- Reproducibility: Consistent results across different testing environments and conditions are crucial for clinical reliability.
- Clinical Utility: The biomarker must have a clear application in enhancing therapeutic strategies, which can drive clinical decisions.
Employing these criteria ensures that the biomarkers identified will be instrumental in real-world applications, adding significant value to pharmacodynamics research.
Biomarker Discovery Techniques
Biomarker discovery comprises various techniques that delve into the molecular basis of drug action and individual response. Below, we explore three primary discovery methods: genomic, proteomic, and metabolomic approaches.
Genomic Approaches
Genomic approaches focus on the comprehensive study of an individual's genes and their expression. These methods allow researchers to identify variations that significantly affect drug metabolism and response. One key characteristic of genomic approaches is their ability to provide insights at the DNA level, uncovering genetic predispositions that can predict which patients will benefit from a particular drug. This aspect makes genomic techniques quite popular in personalized medicine.
However, while genomic data can be incredibly insightful, it comes with certain challenges, like the complexity of genomic interactions within biological systems that can complicate data interpretation. Moreover, it may not capture the dynamic changes occurring in response to treatments, thus necessitating complementary methods for a holistic view.
Proteomic Approaches
Proteomic approaches examine the entire set of proteins produced in an organism, providing insights into the functional aspects of cells and tissues critical for understanding drug actions. The key with proteomics lies in its ability to reflect immediate biological responses to drug administration, capturing dynamic changes over time. This temporal aspect allows for better correlation with therapeutic outcomes.
Nevertheless, proteomics can be resource-intensive, demanding sophisticated technologies and analytical tools to manage large data sets that arise from protein analyses. These costs and complexities can be hurdles for widespread adoption in clinical settings but also open doors for innovative analysis techniques that can ease these challenges.
Metabolomic Approaches
Metabolomic approaches analyze metabolic profiles to assess physiological changes in response to drugs. They offer a snapshot of the biochemical activities and can reveal unique metabolic signatures associated with drug efficacy or toxicity. One remarkable aspect of metabolomics is its ability to provide real-time feedback on treatment effects, given that metabolites are often the end result of biochemical pathways affected by drugs.
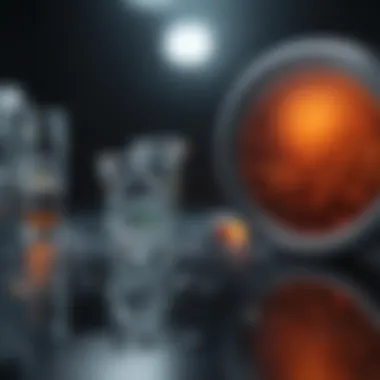
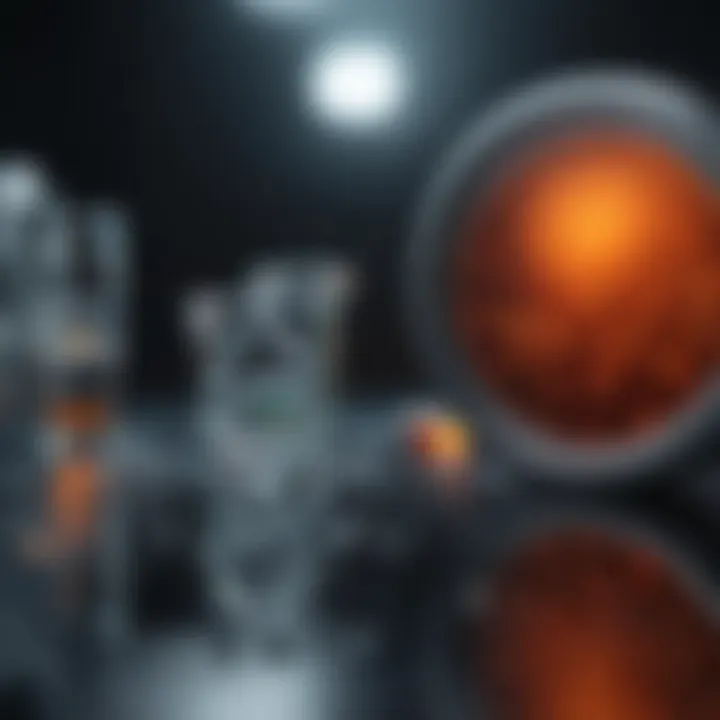
Despite its advantages, such as capturing a wide array of potential biomarkers, metabolomics can run into issues with variability in sample preparation and measurement techniques, limiting reproducibility. These challenges necessitate rigorous standardization protocols to ensure reliability of findings in clinical research.
Validation of Pharmacodynamics Biomarkers
Validation of pharmacodynamics biomarkers is a cornerstone of effective drug development and precision medicine. Biomarkers hold significant promise for improving patient outcomes through personalized therapies, yet the path to utilizing these indicators demands rigorous validation processes. This section delves into the methodologies applied for validating pharmacodynamics biomarkers, highlighting the general processes involved while illuminating their importance in optimizing drug therapies.
Clinical Validation Processes
Clinical validation is a systematic approach to ensure that biomarkers accurately predict clinical outcomes. This involves multiple phases, often starting from preclinical studies right through to Phase IV post-marketing studies.
- Preclinical Phase: At this stage, researchers explore the biological relevance of the biomarker through laboratory experiments, typically using cell lines or animal models. The goal is to establish preliminary evidence that the biomarker can predict drug response.
- Phase I Trials: Here, researchers test the biomarker in a small group of healthy subjects. The key aim at this stage is to understand safety, dosing, and the relationship between the biomarker and pharmacodynamics of the drug.
- Phase II Trials: The validation continues in a target patient population, focusing more on efficacy. The researchers assess whether the biomarker correlates with therapeutic effects.
- Phase III Trials: This is where the biomarker undergoes more formal validation. A larger population is studied, and the relationships between the biomarker and clinical outcomes are carefully analyzed to ensure reliability and accuracy.
- Post-marketing Phase: Even after approval, ongoing studies help to cement the biomarker's utility in a broader real-world population, assessing long-term efficacy and safety.
In all these phases, data collection must be rigorous, and statistical analyses should be meticulous. This ensures that healthcare professionals can confidently use the biomarker for clinical decision-making.
Regulatory Considerations
When discussing the validation of pharmacodynamics biomakers, regulatory considerations play a vital role in the drug approval process. Regulatory bodies like the U.S. Food and Drug Administration (FDA) or the European Medicines Agency (EMA) have set stringent guidelines regarding biomarker validation.
- Guidance Documents: Regulatory agencies provide specific guidance on various types of biomarkers that can influence clinical outcomes. These documents outline the necessary evidence required for the biomarker to be considered valid.
- Risk Assessment: The validation process must address the potential risks that may arise from the use of a biomarker. Itโs critical that the biomarker not only reliably predicts drug efficacy but also mitigates any adverse effects in diverse patient populations.
- Standardization: Consistency in biomarker measurement is crucial. Regulatory bodies require standard protocols for collecting and analyzing biomarker data across different clinical trials to ensure comparability.
- Post-Market Surveillance: After a drug is on the market, the continual collection of data related to the biomarkerโs effectiveness and safety is mandatory. This ongoing surveillance helps refine new therapeutic approaches and can lead to revisions in dosage recommendations or usage guidelines.
Technological Advances in Biomarker Measurement
The landscape of biomarker measurement is ever-evolving, with technological advancements fueling significant progress in pharmacodynamics research. Understanding the advancements in this area is crucial because it shapes how we identify, measure, and apply biomarkers in clinical settings. These technologies not only enhance the accuracy of biomarker detection but also streamline their integration into therapeutic processes, thereby improving patient outcomes and safety.
High-Throughput Screening
High-throughput screening (HTS) has revolutionized the way researchers assess large sets of biological compounds. Its significance cannot be overstated. With the ability to simultaneously test thousands of samples, HTS accelerates the identification of promising biomarker candidates in drug development. The major benefits include:
- Efficiency: HTS reduces the time taken to gather substantial data, making the drug discovery process faster and more efficient.
- Cost-Effectiveness: By minimizing the resources expended in the early stages of research, HTS allows for a more effective budget allocation in pharmaceutical studies.
- Increased Discovery Rates: With the scale at which HTS operates, itโs easier to uncover biomarkers that may have otherwise gone unnoticed.
Moreover, HTS often employs automated systems, making it easier to handle complex biological samples, and ensuring consistency and reliability in data collection. However, a common challenge is the potential for noise in the data, which necessitates robust statistical analysis to ensure that findings are meaningful.
Liquid Biopsy Technologies
Liquid biopsy technologies represent a significant leap forward in biomarker measurement, especially in oncology. Unlike traditional biopsies that require invasive procedures, liquid biopsies rely on the analysis of bodily fluids, primarily blood. This method has several critical advantages:
- Minimally Invasive: Patients experience much less discomfort and risk when undergoing a liquid biopsy.
- Real-Time Monitoring: By allowing for continuous monitoring of biomarkers, clinicians can assess therapeutic efficacy and make necessary adjustments without delay.
- Comprehensive Insights: Liquid biopsies can detect a wider range of biomarkers, providing a more complete picture of the biological activity within a patientโs body.
Despite these benefits, liquid biopsies face hurdles, such as issues related to sensitivity and specificity in detecting low-abundance biomarkers. Ongoing research aims to refine these processes further to enhance their utility in clinical settings.
Bioinformatics and Data Analysis
As the volume of data generated by biomarker measurement techniques continues to grow, bioinformatics has become indispensable. This field combines biology, computer science, and information technology to analyze complex datasets derived from biomarker studies. Key contributions of bioinformatics include:
- Data Integration: Combining datasets from various sources (genomic, proteomic, etc.) helps create a more holistic understanding of biomarker behavior.
- Predictive Modeling: Machine learning algorithms can predict how different biomarkers may interact with drugs, guiding therapeutic decisions.
- Visual Representation: Advanced visualization tools facilitate the interpretation of complex data, making it easier to draw actionable insights.
In summary, the integration of bioinformatics into the realm of biomarker measurement is not just a trend; itโs a necessity as we move into an era where big data plays a paramount role in healthcare.
"Advances in technology demand that we rethink old paradigms and create new ones where information drives the decision, not just instinct."
By embracing and optimizing these technological advances, researchers and practitioners can ensure that pharmacodynamics biomarkers not only contribute to scientific knowledge but also translate into meaningful improvements in patient care.
Pharmacodynamics in Personalized Medicine
Personalized medicine is rapidly transforming the landscape of healthcare, allowing medical professionals to tailor treatments based on individual patient profiles. Within this context, pharmacodynamics biomarkers have emerged as pivotal players in delivering effective and safe therapies. They offer valuable insights into how a patientโs unique characteristics, including genetics, environment, and lifestyle, affect their response to drugs. This section explores the significance of pharmacodynamics in personalized medicine, focusing on how these biomarkers can refine treatment strategies and improve patient outcomes.
Tailoring Treatments to Patients
When it comes to treating diseases, one size definitely does not fit all. By using pharmacodynamics biomarkers, healthcare providers can better understand the nuances of how different patients metabolize drugs. Here are some critical components of this tailoring process:
- Genetic Variability: Individual genetic makeup can significantly influence drug metabolism. For instance, some patients may possess specific enzyme variants that either speed up or slow down drug processing. This variability could dramatically alter drug efficacy and safety. By understanding these genetic factors through biomarker testing, treatment can be customized to enhance therapeutic effects while minimizing adverse reactions.
- Disease Specificity: Different disease manifestations in patients may benefit from distinct drug actions. Pharmacodynamics biomarkers can identify which therapeutic pathways are most effective for a patient's particular disease profile. This helps in selecting the right drug at the right dosage.
- Patient Engagement: Personalized medicine fosters better communication between healthcare providers and patients. When aspecial treatments based on their biological profiles are discussed, patients are more likely to be engaged in the treatment process, leading toward better adherence and outcomes.
In practical terms, this means that a patient diagnosed with cancer might receive a completely different treatment regimen than one with the same diagnosis but a different genetic makeup. For example, patients with certain mutations in the epidermal growth factor receptor (EGFR) may respond well to drugs like Erlotinib, while others without these mutations may not see the same benefit. Such targeted approaches represent a paradigm shift in pharmacotherapy, driven primarily by the insights gained from pharmacodynamics biomarkers.
Challenges in Implementation
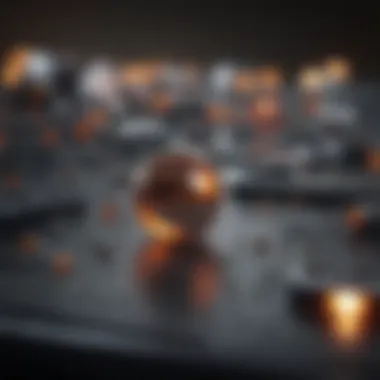
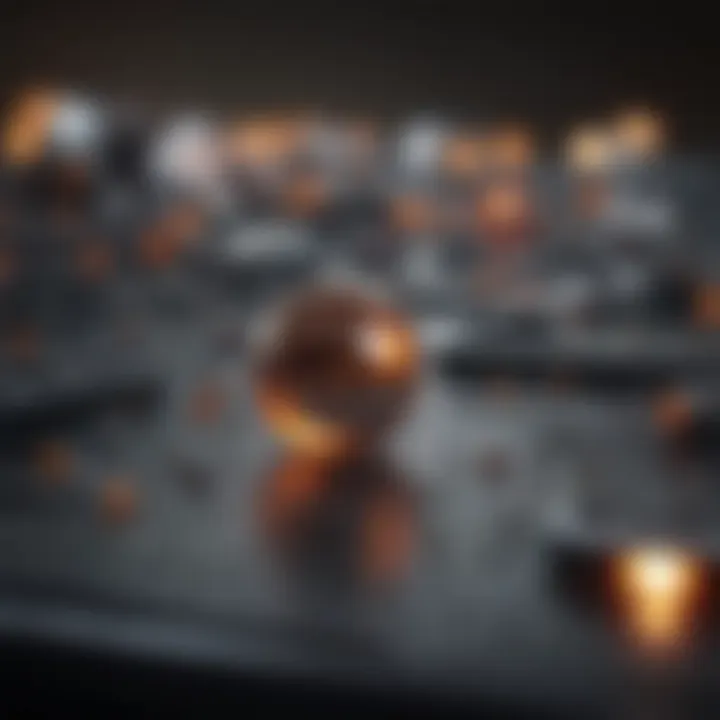
Despite the promise of personalized medicine, the integration of pharmacodynamics biomarkers into clinical practice brings along a set of challenges that need careful consideration. Key obstacles include:
- Cost of Testing: The financial burden of biomarker testing can be substantial, particularly in low-resource settings. Laboratory costs, alongside the healthcare system's capacity to front these expenses, can pose barriers to wider adoption.
- Regulatory Hurdles: Navigating the regulatory landscape can slow down the implementation of pharmacodynamics biomarkers. Often, there is a lag between scientific advancements and regulatory approval processes. Regulatory bodies require robust evidence demonstrating that the biomarker can reliably predict clinical outcomes.
- Clinical Practice Variation: Different healthcare providers may have varying levels of familiarity and comfort with interpreting pharmacodynamics data. This inconsistency can lead to disparities in treatment approaches, affecting overall patient care.
- Ethical Considerations: As genomics and biomarkers become more foundational, ethical dilemmas arise surrounding data privacy, potential discrimination, and informed consent. Patients must be fully educated about the implications of undergoing biomarker testing before they can make informed decisions.
"Personalized medicine, powered by pharmacodynamics biomarkers, holds the potential to transform patient care, but its path is fraught with challenges that must be navigated carefully."
Addressing these challenges requires collaborative efforts among researchers, clinicians, patients, and policymakers, ensuring that the promise of pharmacodynamics in personalized medicine is not just a lofty ideal but a practical reality.
Clinical Applications of Pharmacodynamics Biomarkers
Pharmacodynamics biomarkers are pivotal in the clinical landscape, especially in their application across various fields of medicine. Understanding these biomarkers helps clinicians and researchers tailor treatments specifically to the needs of patients, ultimately enhancing therapeutic outcomes. The significance of this section lies in its ability to elucidate how biomarkers can transform practices in oncology, cardiology, and neurologyโareas where precision in drug therapy can lead to markedly different patient experiences and outcomes.
Oncology
In oncology, the role of pharmacodynamics biomarkers is increasingly vital as it directly correlates with how effectively we can treat cancer. Biomarkers such as the estrogen receptor (ER) in breast cancer, or the programmed cell death protein 1 (PD-1) in various tumors, are fundamental in determining the appropriate course of treatment. For instance, the presence of these biomarkers can indicate whether a patient will benefit from hormone therapy or immunotherapy, respectively.
Moreover, pharmacodynamics biomarkers help predict tumor response and resistance to chemotherapy, thus facilitating personalized treatment plans. This not only enhances patient safety by avoiding ineffective therapies but also optimizes resource utilization in healthcare.
"The identification of specific biomarkers can mean the difference between life and death in oncology; understanding their nuances is essential for clinicians."
Cardiology
In cardiology, pharmacodynamics biomarkers play a crucial role in disease management and therapeutic monitoring. For example, levels of troponin in the bloodstream serve as markers for myocardial injury, guiding the clinical decision-making process in acute coronary syndromes. Additionally, the presence of specific biomarkers such as B-type natriuretic peptide (BNP) can aid in diagnosing heart failure and assessing its severity. This tailored approach leads to better-targeted therapies and timely interventions, which can significantly improve patient outcomes.
Risk assessment is another realm where pharmacodynamics biomarkers shine. By analyzing genetic and proteomic markers, clinicians can evaluate a patient's risk for cardiovascular diseases, allowing for proactive management strategies tailored to individual risk profiles.
Neurology
In neurology, pharmacodynamics biomarkers are increasingly recognized for their potential to revolutionize the way we approach treatment for various neurological disorders. For instance, in Alzheimerโs disease, biomarkers such as amyloid-beta and tau proteins measured through cerebrospinal fluid play a crucial role in early diagnosis and monitoring disease progression. These insights not only help in understanding the individualโs condition but also contribute to the broader research efforts aimed at developing new treatments.
Additionally, biomarkers can guide treatments for conditions like epilepsy or multiple sclerosis, providing insights into treatment responses. Personalized dosing regimens and medication selections based on pharmacodynamic analysis can considerably reduce adverse effects and enhance the quality of life for patients.
In summary, the clinical applications of pharmacodynamics biomarkers across oncology, cardiology, and neurology underline their transformative potential in medical practice. With ongoing advances in biomarker discovery, the horizon for personalized medicine looks promising, albeit it comes with challenges regarding implementation and resource allocation.
Future Directions and Challenges
The exploration of pharmacodynamics biomarkers is a frontier continually evolving within the pharmaceutical landscape. Their significance cannot be understated; these biomarkers not only contribute to the comprehension of drug interactions but also help tailor therapeutic approaches for individual patients. As we look to the future, several elements need attention to navigate the challenges ahead effectively.
- Integration of New Technologies: Advances in technologies, such as CRISPR gene editing and artificial intelligence, promise to refine biomarker discovery and validation processes. For instance, employing AI can analyze clinical data faster, revealing patterns that may suggest new biomarkers that weren't previously considered or identified by traditional methodologies.
- Collaboration Across Disciplines: The intersection of biology, chemistry, and data science presents an incredible opportunity. By fostering collaboration among researchers, we can create robust frameworks for biomarker research. This interdisciplinary approach can enhance our understanding of complex biological systems.
- Regulatory Challenges: Perhaps one of the most formidable hurdles lies with regulatory frameworks. The protocols are sometimes slow to adapt to rapid advancements in biomarker technologies. A re-evaluation of regulations may be necessary to allow for quicker approval of new discoveries, thus benefiting patient care earlier and more efficiently.
Emerging Research Areas
As we venture into the realm of pharmacodynamics biomarkers, several avenues for innovative research appear promising:
- Microbiome Influences: There's growing evidence indicating that the gut microbiome can affect how drugs are metabolized. Understanding these interactions may help in identifying new biomarkers predictive of drug response.
- Genomic and Epigenomic Biomarkers: Delving into how genetic variations influence drug reactions continues to be vital. Recently, research into epigenomic alterations has gained traction, potentially revealing biomarkers that can predict a patientโs drug response more accurately.
- Digital Biomarkers: With the rise of mobile health technologies, digital biomarkers acquired from user activity and engagement can provide rich, real-time datasets that could reliably indicate drug efficacy over time.
"The future landscape of pharmacodynamics biomarkers will be shaped by both technological ambitions and the ethical frameworks we establish."
Ethical Considerations
With any advancement in the field of pharmacodynamics biomarkers, ethical considerations come to the fore. These dilemmas must guide research and implementation to uphold the integrity of scientific progress:
- Patient Privacy: Collecting data for biomarker research must respect patient confidentiality. Transparency in how data is used, shared, and stored is paramount to maintain trust between patients and researchers.
- Equitable Access: As discoveries advance, the risk of creating disparities in healthcare increases. Ensuring that new treatments stemming from biomarker research are accessible to all demographics, regardless of socioeconomic status, must be a priority.
- Informed Consent: Participants in biomarker studies should fully understand the implications of their involvement. This includes potential risks and the use of their biological data, calling for a thorough consent process that respects their autonomy.
In summary, the exploration of pharmacodynamics biomarkers is laden with potential. However, it is imperative to tread carefully, addressing challenges while remaining cognizant of ethical responsibilities. Only then can we genuinely harness the power of biomarkers to revolutionize medicine.
Culmination
In this final section, we explore the significance of the topic and the weight it carries within the broader context of pharmacodynamics biomarkers. The insights presented throughout the article underline that understanding pharmacodynamics biomarkers is not just a scientific endeavor but a vital part of transforming medical therapies. From influencing drug interactions to shaping personalized medicine, biomarkers serve as crucial indicators in evaluating efficacy and safety.
Summarizing Key Points
To effectively conclude, it is essential to summarize the critical components discussed in this exploration:
- Role in Drug Development: Pharmacodynamics biomarkers contribute substantially to the drug development pipeline by assisting in the identification of effective therapeutic interventions.
- Mechanism Understanding: Insights into drug-receptor interactions and dose-response relationships provided context for how biomarkers can reveal the dynamics of drug action in real time.
- Validation and Technological Advances: The rigorous validation processes and the advent of technologies like liquid biopsies and bioinformatics streamline the efficiency of biomarker measurement.
- Clinical Implications: Their application across various fields, notably oncology, cardiology, and neurology, emphasizes their versatility and critical importance in clinical settings.
- Ethical Considerations: Discussions regarding the ethical ramifications of biomarker use highlight the need for conscientious approaches in research and application.
Overall, pharmacodynamics biomarkers bridge the gap between bench and bedside, anchoring treatments in real-world biology and individualized patient care.
Call to Action for Future Research
Looking forward, itโs evident that while progress has been made, there is still a long road ahead. Future research should prioritize:
- Innovative Methodologies: Development of new biomarker discovery techniques that are not only effective but also capable of capturing the complexities of biological variability in human subjects.
- Integrative Approaches: Encouraging collaboration between disciplines, such as genomics, proteomics, and clinical pharmacology, to enhance understanding and identification of multidimensional biomarkers.
- Ethical Frameworks: Establishing robust ethical guidelines that govern research, ensuring that the benefits of biomarker technologies do not come at the cost of patient autonomy or safety.
- Broadening Scope: Expanding the focus of research to include underrepresented populations in clinical studies, ensuring that findings are universally applicable and beneficial.