Exploring MSN Nanoparticles: A Comprehensive Overview
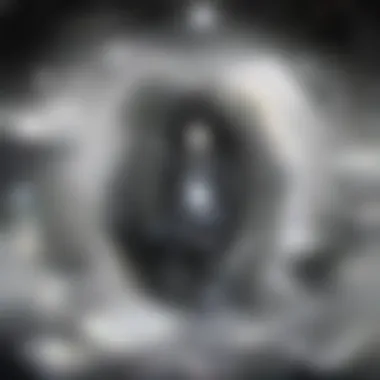
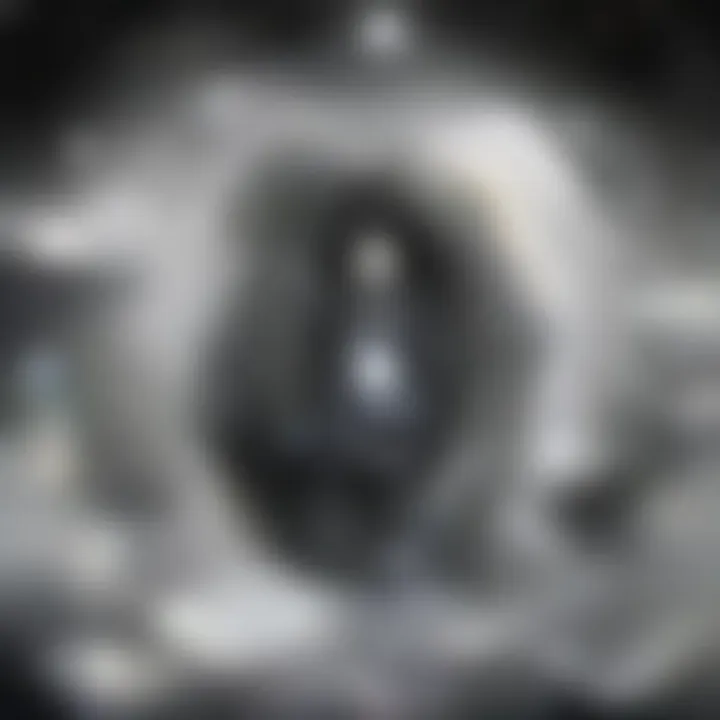
Intro
The exploration of mesoporous silica nanoparticles (MSN) is essential for advancing nanotechnology in various fields. These nanoscale structures possess significant properties, including large surface areas and tunable pore sizes, making them valuable for numerous applications, particularly in biology and materials science. This article covers the intricacies of MSN, highlighting their composition, synthesis methods, and diverse applications. It also addresses the challenges researchers face and provides insights into future directions for MSN technology.
Research Overview
Summary of Key Findings
Mesoporous silica nanoparticles have emerged as a versatile platform in biomedical applications. Key findings regarding their characteristics include:
- High surface area allows for effective drug loading.
- Uniform mesopore structure facilitates controlled release of therapeutic agents.
- Biocompatibility enhances their acceptability in medical contexts.
Their ability to encapsulate various substances opens new avenues in drug delivery systems, imaging techniques, and environmental remediation strategies.
Methodologies Employed
The synthesis of MSN can be achieved through several methods:
- Sol-gel process - A widely used technique for fabricating silica nanostructures, offering control over particle size and surface chemistry.
- Template-assisted synthesis - Involves using surfactants or hard templates, which leads to well-defined pore structures.
- Co-condensation - This method allows the incorporation of functional groups directly during the synthesis, vital for drug targeting.
These methodologies have undergone extensive research to improve efficiency and adaptability.
In-Depth Analysis
Detailed Examination of Results
Recent studies indicate that the improved functionalization of MSN enhances their performance in drug delivery. For instance, using specific targeting ligands can improve accumulation in diseased tissues.
Moreover, characterization techniques, such as N2 adsorption/desorption isotherms, transmission electron microscopy, and dynamic light scattering, are pivotal in elucidating structural properties. Each of these techniques provides distinct advantages in understanding the physical characteristics and behavior of MSN in various environments.
Comparison with Previous Studies
Comparative analyses reveal significant advancements over earlier MSN formulations. Older nanoparticle designs often lacked adequate surface functionalities and surface stability. Modern MSN, however, incorporate advances in surface engineering, resulting in significant improvements:
- Enhanced drug release kinetics.
- Increased cellular uptake.
- Superior imaging contrast in medical applications.
This upward trend in effectiveness underlines the need for ongoing research and development in the field.
Prelims to MSN Nanoparticles
The exploration of mesoporous silica nanoparticles (MSN) occupies a prominent role in the evolving landscape of nanotechnology. Their unique structural characteristics and versatile applications make them a focal point in scientific research and industry practices. Understanding these nanoparticles is essential for identifying their potential in diverse fields such as drug delivery, biomedical imaging, and environmental solutions.
Defining Mesoporous Silica Nanoparticles
Mesoporous silica nanoparticles are distinguished by their porous structure. These nanoparticles possess well-defined pore sizes, typically ranging from 2 to 50 nanometers, which enhance their surface area and functionality. The porosity enables effective loading and release of various molecules, making them ideal candidates for targeted delivery systems. The inherent biocompatibility of silica further adds to their appeal in biomedical applications.
The synthesis of mesoporous silica nanoparticles can be achieved through various methods, such as sol-gel processes and template-assisted techniques. The choice of method determines their structural attributes and functional capabilities. Once synthesized, the ability to customize their surface through functionalization opens new avenues for applications, ranging from drug carriers to diagnostic tools.
Historical Context and Development
The history of mesoporous silica nanoparticles traces back to the late 1990s, when researchers began to recognize the potential of silica nanostructures. Initial investigations focused on characterizing their physical properties, laying the groundwork for future innovations. Over the years, advancements in synthesis techniques allowed for the tunability of their size and surface characteristics, further enhancing their applications.
The introduction of the Mobil Composition of matter No. 41 (M41S) family of mesoporous silicas, which includes MCM-41, marked a pivotal moment in the development of MSN. These early materials demonstrated significant promise in catalytic and adsorption processes. Since then, the focus has expanded to more bio-relevant applications, highlighting the need to understand MSN's multifunctional properties.
Research continues to evolve, with interest in integrating MSN into hybrid systems, enhancing their capabilities. As the understanding of these materials deepens, so too does the potential for incorporating them into innovative technologies that address complex problems in health and environmental sectors.
"The capacity to manipulate the nano-scale architecture of mesoporous silica nanoparticles opens new horizons in material science and biomedical research."
Chemical Composition of MSN
Understanding the chemical composition of mesoporous silica nanoparticles (MSN) is essential for comprehending their unique properties and potential applications. The basic building block of MSN is silica, which plays a critical role in defining their structural integrity and functionality. The choice of silica as a foundational material contributes to the high surface area and porous structure characteristic of these nanoparticles. These properties are vital for applications ranging from drug delivery to environmental remediation, making the study of their composition increasingly relevant in scientific research.
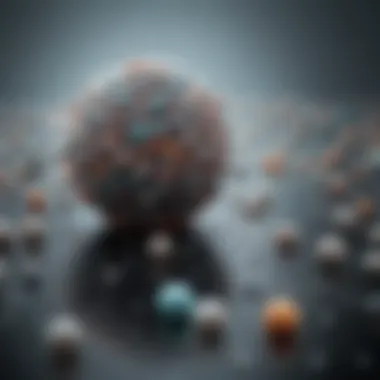
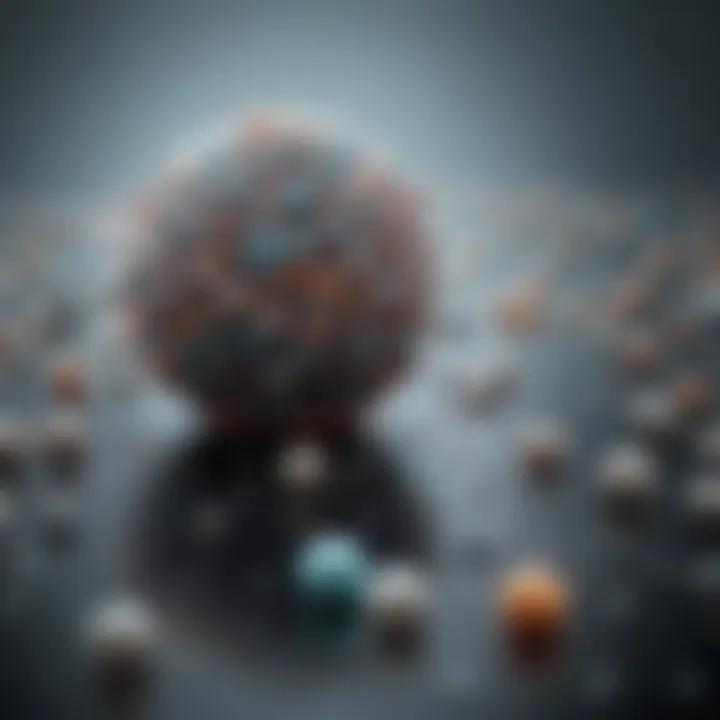
Silica Framework Structure
The silica framework of MSN is characterized by its mesoporous structure, which refers to pore sizes in the range of 2 to 50 nanometers. This specific range allows for the encapsulation and transportation of various substances, enhancing their utility in biomedical applications. Additionally, the porous nature of silica enables high loading capacity for drugs or diagnostic agents, which is a key advantage in formulations aimed at targeted drug delivery.
The framework structure also facilitates significant modifications. By manipulating the pore size or geometry, researchers can optimize the MSN properties for specific applications. Thus, the silica framework is not just a structural feature; it also directly influences the drug release kinetics and bioavailability of therapeutic agents embedded within.
Functionalization Strategies
Functionalization of MSN is central to tailoring their surface properties, enhancing their compatibility with various biological and environmental systems. Two main strategies are often discussed: surface modifications and materials compatibility.
Surface Modifications
Surface modifications involve the addition of various functional groups to the silica framework. This is crucial for improving interaction with biological targets or enhancing stability in environmental settings. For example, attaching amino or carboxyl groups can significantly affect the drug release profile. This makes surface modifications a popular choice in designing MSN for drug delivery applications.
A key characteristic of surface modifications is their ability to enable targeted delivery. For instance, conjugating ligands that can recognize specific cell markers allows for precise targeting. However, these modifications come with challenges, such as potential toxicity or undesired immune responses. It is essential to evaluate the balance between functionality and safety in these advanced designs.
Materials Compatibility
Materials compatibility focuses on the interaction of MSN with other substances, including therapeutic agents and biological systems. This aspect is fundamental in determining how effectively MSN can be utilized in practical applications. The compatibility of MSN with various drugs can affect not only their stability but also their efficacy once administered.
One key advantage of materials compatibility is the potential for synergistic effects. When combined with specific biodegradable polymers or lipids, MSN can enhance drug solubility or bio-distribution. Nevertheless, achieving optimal compatibility requires extensive research to understand how different materials interact with the silica framework to avoid adverse reactions or instability during storage.
"The unique properties of MSN largely depend on their chemical composition, which determines overall performance in diverse applications."
In summary, the chemical composition of MSN significantly influences their utility across a range of fields. The silica framework and various functionalization strategies are pivotal in advancing the performance of these nanoparticles, hence essential for future innovations and applications.
Synthesis Methods of MSN
The synthesis of mesoporous silica nanoparticles (MSN) is critical to their development and application in various fields. Understanding the synthesis methods is essential, as it influences the structural and functional properties of MSN. Each method offers unique benefits but also presents certain challenges. Knowledge of these techniques is vital for researchers and professionals looking to tailor nanoparticles for specific uses.
Sol-Gel Process
The sol-gel process is one of the most commonly used techniques for synthesizing MSN. This method involves the transition of a solution into a solid gel phase, facilitating the formation of a silica network. The basic principles of the sol-gel process include hydrolysis and condensation reactions of silicate precursors like tetraethyl orthosilicate (TEOS).
This technique provides a high level of control over particle size and porosity. For instance, by adjusting the reaction conditions, such as pH and temperature, one can manipulate the final properties of the nanoparticles. Another advantage of the sol-gel process is its scalability, which makes it suitable for large-scale production while maintaining reproducibility. Nonetheless, careful management of variables is essential to achieve the desired outcomes.
Template-Assisted Techniques
Template-assisted techniques enable the creation of MSN with specific pore structures and sizes. In this method, hard or soft templates are utilized to define the shape and arrangement of nanoparticles. Common materials used as templates include polymers and surfactants.
One of the primary benefits of this technique is the ability to produce MSN with highly uniform and tunable pore sizes. For example, by selecting different surfactants or template materials, the final structure can be customized for targeted applications, such as drug delivery or catalysis. However, a notable drawback is the requirement for template removal, which can add complexity to the synthesis process. This must be addressed to ensure the structural integrity of the MSN is preserved.
Co-assembly Approaches
Co-assembly approaches offer another innovative method for synthesizing MSN. This technique involves the simultaneous organization of silica precursors and other materials during the formation of nanoparticles. These materials can include organic compounds or biological molecules, which can be integrated into the silica framework.
The advantage of co-assembly is its potential for functionalization directly during the synthesis phase. This means that specific chemical groups can be introduced into the MSN, enabling tailored interactions for various applications. On the downside, controlling the co-assembly conditions may be more complex, requiring fine-tuning to avoid undesired aggregation or uneven distribution of components.
Overall, the synthesis methods of MSN are pivotal in determining the properties and applications of these nanoparticles. By understanding these techniques, researchers can optimize the nanoparticles for specific needs in areas like drug delivery, imaging, and environmental remediation.
Characterization of MSN Nanoparticles
Characterization of mesoporous silica nanoparticles (MSN) plays a critical role in understanding their physical and chemical properties. This step is essential for optimizing their use across various applications, including drug delivery and environmental remediation. Proper characterization helps researchers assess the quality and performance of these nanoparticles. Several methods exist for characterization, and they provide insights into the surface area, pore size, morphology, and chemical composition of MSN. Each aspect contributes to determining how these nanoparticles will behave and perform in different scenarios.
Surface Area and Pore Size Analysis
Surface area and pore size are pivotal in determining the functionality of MSN. High surface area generally enhances the interaction between the nanoparticles and the target environment, critical for drug delivery systems. Pore size impacts the loading capacity and release profiles of drugs. Techniques such as Brunauer-Emmett-Teller (BET) analysis are typically employed to determine these parameters. Through this analysis, researchers can refine MSN properties, tailoring them for specific applications. A clear understanding of surface and pore characteristics directly influences the effectiveness and efficiency of MSN in various fields.
Morphological Characterization
Morphological characterization provides information about the shape and size of MSN nanoparticles. This understanding is crucial for assessing how these nanoparticles will aggregate or interact in biological and environmental contexts.
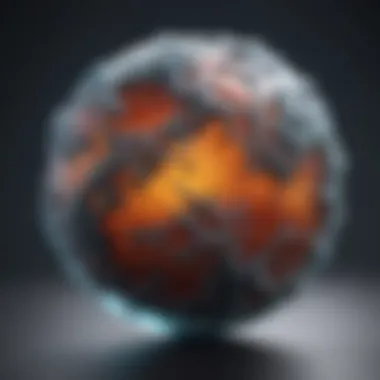
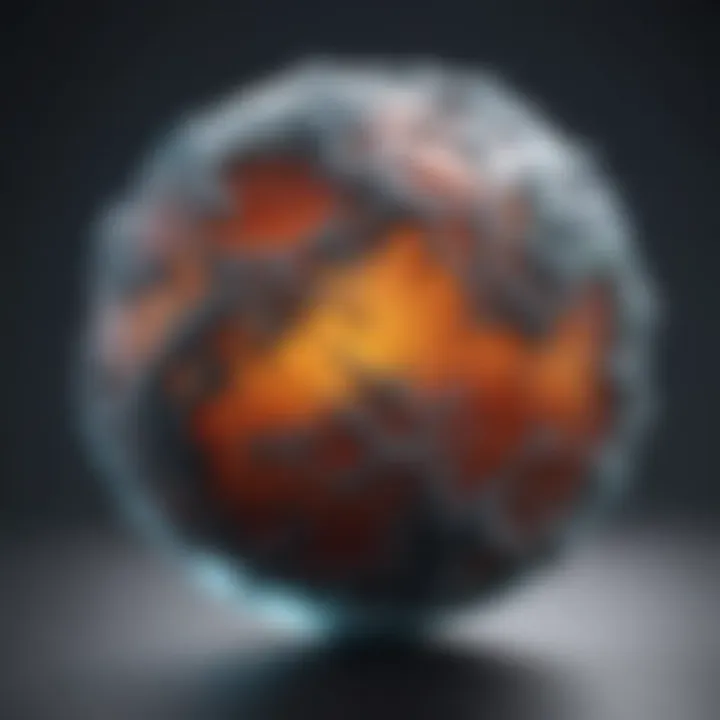
Electron Microscopy Techniques
Electron microscopy techniques, such as Transmission Electron Microscopy (TEM) and Scanning Electron Microscopy (SEM), offer detailed images of MSN at the nanoscale. TEM provides high-resolution images that reveal both morphology and size distribution, presenting a clear view of structural integrity. SEM, on the other hand, offers surface topography details which are crucial for understanding how MSN can interact with biological systems. This method is a popular choice when precision in visualizing the nanoparticle's structure is needed.
However, these electron microscopy techniques do require that samples be prepared under very specific conditions, which can introduce artifacts potentially affecting the observations.
Dynamic Light Scattering
Dynamic Light Scattering (DLS) measures the size distribution of nanoparticles in solution based on how they scatter light. This technique is critical for estimating the hydrodynamic size of MSN, which represents how the particles behave in a liquid medium. The primary advantage of DLS is its ability to provide rapid results and manage a wide range of particle sizes in suspension. This efficiency makes DLS particularly beneficial for routine characterization tasks. One disadvantage, however, is that DLS can be less informative for non-spherical particles, which may misrepresent actual sizes based on their scattering behavior.
Chemical Composition Analysis
Chemical composition analysis is critical for understanding the functional groups present in MSN, which directly influences their reactivity and interaction with various compounds. Knowing the chemical makeup helps tailor MSN for specific applications.
X-ray Diffraction (XRD)
X-ray Diffraction (XRD) is a powerful technique for determining the crystallinity and phase composition of MSN. The unique aspect of XRD is its ability to identify structural properties at the atomic level. This method stands out because it provides insights into the arrangement of atoms, assisting researchers in ensuring that the synthesized MSN meet specific criteria for intended applications. While XRD is a strong choice due to its robustness, it requires careful sample preparation to avoid contamination which might skew results.
Fourier Transform Infrared Spectroscopy (FTIR)
Fourier Transform Infrared Spectroscopy (FTIR) allows for the detection of chemical bonds and functional groups in MSN. This technique is significant as it reveals the surface chemistry of the nanoparticles. It is widely used because it can provide rapid results with high sensitivity and specificity, which is vital for tuning the functionalization of MSN. One limitation is that FTIR typically requires a sufficient sample quantity, which can be a concern when working with nanoparticle quantities.
The accurate characterization of MSN nanoparticles not only informs their current applications but also lays the groundwork for future innovations.
Applications of MSN Nanoparticles
The applications of mesoporous silica nanoparticles (MSN) are diverse and impactful, stretching across various fields such as medicine, environmental science, and materials engineering. Each application's significance lies in its ability to leverage the unique properties of MSNโsuch as high surface area, tunable pore size, and surface functionalization. These properties not only enhance the effectiveness of MSN in specific tasks but also create avenues for innovation in existing practices.
Drug Delivery Systems
Targeted Delivery Mechanisms
Targeted delivery mechanisms are fundamental in enhancing the effectiveness of drug therapies. They focus on directing therapeutic agents precisely to diseased tissues. This specificity minimizes off-target effects, ultimately improving therapy outcomes. The significant characteristic of these mechanisms is their precision. MSN can be engineered to release drugs in response to specific stimuli, such as pH changes or enzyme presence in target sites. This controllability is a reason why targeted delivery is prevalent in current research on drug delivery systems. The unique feature of these strategies is their ability to improve bioavailability. However, challenges remain, including potential immune responses and the need for thorough testing.
Controlled Release Profiles
Controlled release profiles are essential for optimizing dosage regimens in drug therapy. By allowing active substances to be released over extended periods, they reduce the frequency of administration and enhance patient compliance. This application is popular because it aligns with the goals of modern pharmacotherapy, which seeks to minimize peaks and troughs in drug concentration. A defining feature is their ability to sustain therapeutic levels for longer durations. While advantageous, there are potential downsides, including the risk of overloading the system if not correctly calibrated.
Biomedical Imaging
Fluorescence Imaging
Fluorescence imaging is a powerful tool in biomedical applications, allowing researchers to visualize biological processes in real-time. A key characteristic of fluorescence imaging is its sensitivity, enabling the detection of low-abundance molecules. This imaging method is compelling for its non-invasive nature, making it favorable in clinical settings. The unique feature of integrating MSN with fluorescent dyes enhances image resolution and specificity for targeted cells or tissues. However, there are limitations such as photobleaching and depth penetration concerns that need consideration.
Magnetic Resonance Imaging (MRI)
Magnetic Resonance Imaging (MRI) offers high-resolution images of soft tissues, which is paramount for accurate diagnosis. The essential attribute is its unparalleled soft-tissue contrast, making it widely preferred in clinical imaging. The use of MSN in MRI as contrast agents reflects a beneficial direction for enhancing signal intensity. Their unique capability lies in functionalization that improves relaxation times. Nonetheless, there are considerations regarding biocompatibility and regulatory challenges that necessitate thorough evaluation before widespread use.
Environmental Applications
Water Treatment
The application of MSN in water treatment is gaining traction due to their high adsorption capacity for contaminants. This application is increasingly important as water quality deteriorates globally. A key characteristic is the porous nature of MSN, which allows for effective removal of both organic and inorganic pollutants. The unique feature of using MSN in water treatment is their ability to regenerate after sorption, making them a sustainable choice. Challenges include scalability and potential leaching of materials into treated water.
Adsorption of Pollutants
MSN's capability for the adsorption of pollutants is vital in combating environmental pollution. This involves the capacity to adsorb various harmful substances, including heavy metals and dyes. Their high surface area is a significant advantage for rapid adsorption. This feature enables efficient cleanup processes for different kinds of pollutants. However, this application often faces limits in terms of selectivity, as certain pollutants may not interact optimally with MSN, requiring further research to enhance efficiency.
Overall, the applications of MSN nanoparticles present a multitude of benefits but also come with inherent challenges that require continued exploration and innovation.
Challenges and Limitations
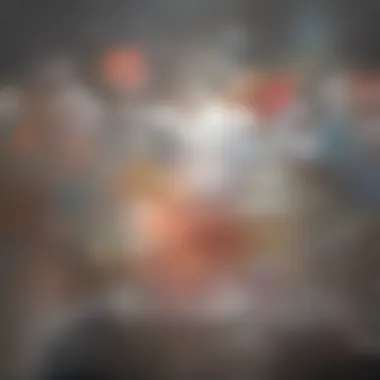
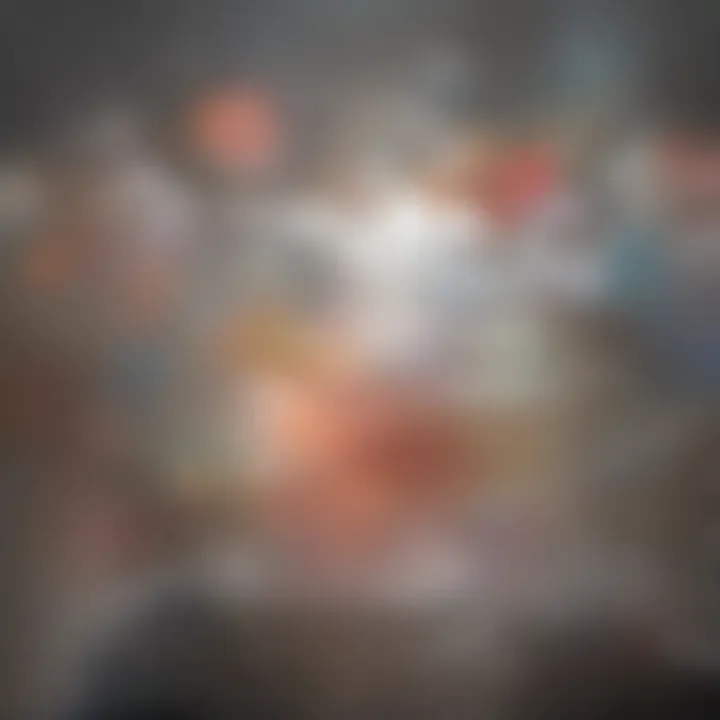
Understanding the challenges and limitations surrounding mesoporous silica nanoparticles (MSN) is vital for advancing their applications in various fields, particularly in biomedical and environmental sectors. These issues can influence research focus, funding availability, and ultimately the successful integration of MSNs into real-world applications.
Toxicity Concerns
Toxicity is one of the most significant concerns when it comes to the utilization of MSN in medical applications. While MSN offers many benefits, such as enhanced drug delivery mechanisms, they can also pose risks to human health and the environment. Studies indicate varying degrees of cytotoxicity related to the size, shape, and functionalization of the nanoparticles.
Researchers have noted that smaller nanoparticles may penetrate cells more easily, leading to potential toxicity. Some research suggests that silica nanoparticles can induce oxidative stress or lead to inflammation. These effects can be detrimental and necessitate an evaluation of biocompatibility before clinical application. Thus, ongoing studies aim to establish a balance between functionality and safety.
Regulatory Hurdles
Regulatory approval processes for nanoparticles like MSN are complex and vary across different regions. Agencies such as the FDA in the United States require comprehensive testing to assess safety and effectiveness, which can be time-consuming and costly. The absence of a standardized framework for evaluating nanomaterials complicates these processes further. Each application, whether it is for drug delivery or environmental remediation, can face unique challenges due to varying regulatory requirements.
Moreover, there is often uncertainty regarding the long-term effects of these materials in biological systems. As a result, regulatory bodies demand extensive data, leading to potential delays in the introduction of innovative technologies to the market. Understanding regulatory landscapes is crucial for researchers and manufacturers aiming to bring MSN applications to fruition.
Scalability of Production
Another challenge lies in the scalability of MSN production. Although existing laboratory synthesis methods demonstrate success at a small scale, translating these processes to large-scale manufacturing poses difficulties. Factors such as consistency in particle size, shape, and functionalization become critical when moving beyond research settings.
To address issues of reproducibility and cost-effectiveness, researchers are exploring various synthesis techniques, adapting those methods that work well in a lab setting to industrial capacities. The pressure of production scalability may also inspire innovations in synthesis methodologies to ensure that MSN can meet market demands efficiently.
Effective collaboration between scientists and industry stakeholders is essential for navigating these challenges, paving the way for more widespread use of MSN in various fields.
Future Directions in MSN Research
The future of mesoporous silica nanoparticles (MSN) research is multifaceted, with a scope that extends beyond traditional applications. The evolving landscape of technology and science demands continuous innovation in the functionality and utility of MSNs. Understanding the future directions in MSN research can provide insights into its potential and limitations. This section outlines the emerging areas of interest that researchers and industries are focusing on, elucidating the expected benefits and considerations.
Emerging Trends in Functionalization
Functionalization of MSN is a major focus as it allows customization of properties for specific applications. Researchers are pursuing new surface modification techniques to enhance target specificity and reduce potential toxicity. Innovative functional groups can be tailored for drug delivery systems, improving biosustainability and interaction with biological environments. This trend not only expands the applicability of MSNs in medical fields but also promotes their functionality in diagnostics and therapeutics. Improved functionalization strategies can facilitate the selective targeting of cancer cells, enhancing the precision of treatment regimens.
Integration with Other Nanomaterials
The integration of MSN with other nanomaterials represents a promising avenue for future research. Combining MSNs with materials like graphene, carbon nanotubes, or metal nanoparticles fosters synergies that can enhance their performance in areas like catalysis, energy storage, and sensing. For instance, hybrid nanomaterials may improve the efficacy of drug delivery by leveraging the mechanical advantages of each component. This integration can also lead to unique optical or electronic properties, significantly expanding the potential applications of MSNs.
Exploration of New Applications
As research advances, the exploration of new applications for MSNs remains a critical area. Beyond drug delivery and imaging, potential applications in environmental remediation, biosensing, and agriculture are gaining traction. In environmental science, MSNs can be utilized for the efficient removal of pollutants from water sources due to their high surface area and customizable porosity. Additionally, in the agricultural sector, they may serve as carriers for fertilizers, improving nutrient release rates, and promoting sustainable agricultural practices.
"The continual evolution of MSN technology is crucial for meeting future challenges in various fields, including biomedicine and environmental science."
In summary, future directions in MSN research encompass an array of emerging functionalization trends, synergies with other nanomaterials, and the discovery of new applications. These developments hold significant promise in addressing current challenges while expanding the scope and impact of mesoporous silica nanoparticles. The interconnectivity of these advancements illustrates the potential of MSNs to transform various industries, underlining the importance of continued research and innovation.
Epilogue
The conclusion of this article serves as a crucial component in wrapping up the multifaceted discussion surrounding mesoporous silica nanoparticles (MSN). By synthesizing key insights into their composition, applications, and the challenges that confront their deployment, this section emphasizes their growing significance in scientific inquiry and practical applications.
One important element to consider is the versatility of MSN as a platform for various technologies. Their unique properties enable them to be tailored for specific functions in drug delivery, biomedical imaging, and environmental remediation. This adaptability not only underscores their relevance in current research but also sparks interest in exploring new avenues of application.
Furthermore, the benefits of incorporating MSN in medical and environmental contexts are substantial. The capacity for targeted drug delivery can enhance therapeutic efficacy while minimizing side effects, which is crucial in fields like oncology. Likewise, their role in environmental applications signifies a proactive approach to pollution management, thus benefiting public health.
However, it is essential to acknowledge ongoing considerations, such as toxicity and scalability of production. These challenges require meticulous attention and innovative solutions to truly harness the potential of MSN. As we could see, while the promise of MSN is vast, it is balanced by the necessity for careful evaluation of risks and regulatory compliance.
"Understanding the full spectrum of MSN capabilities is key to unlocking their potential benefits across various fields, emphasizing both innovation and responsibility."
In closing, the importance of this conclusion rests not just in summarizing the existing information but in pointing toward a future where MSN can achieve their fullest potential in various applications while ensuring safety and effectiveness.
Summary of Key Points
- Mesoporous silica nanoparticles possess remarkable versatility in composition and function.
- Their applications span medical fields, particularly in drug delivery and imaging, as well as environmental solutions.
- Current challenges include toxicity, regulatory issues, and scalable production.
- Future innovations depend on careful assessment and exploration of new application domains.
Implications for Future Research
The implications for future research on MSN nanoparticles are profound. As technology progresses, further exploration into functionalization and integration with other nanomaterials will be pivotal. This may lead to advanced applications in medical therapeutics and diagnostics, as well as enhanced environmental cleaning technologies.
Moreover, understanding the environmental impact and long-term effects of MSN is critical to ensuring their safe use in real-world applications. Investigating how these nanoparticles interact with biological systems will provide necessary insights to mitigate potential toxicity risks and inform regulatory frameworks.
As the landscape of nanotechnology continues to evolve, it is vital for researchers to remain vigilant about both the opportunities and challenges that MSN entail. Collaboration across disciplines will further enrich this field, paving the way for innovative breakthroughs that impact society positively.