Exploring Molecular Sieves: Principles and Applications
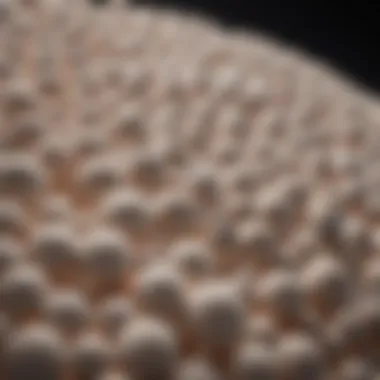
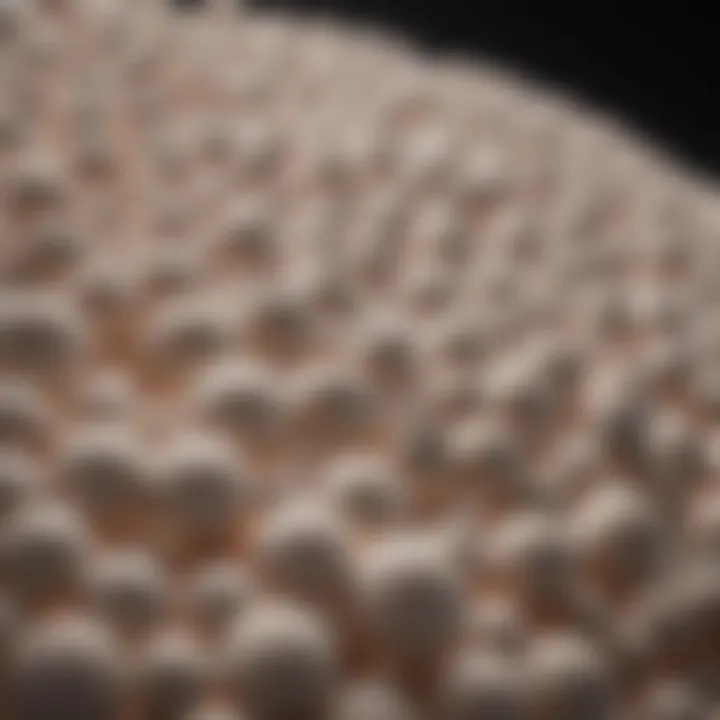
Research Overview
In today's fast-paced world, molecular sieves are quietly revolutionizing multiple sectors, from environmental science to pharmaceuticals. The quest to grasp their fundamental principles and applications forms the backbone of this article. Exploring these intricate materials sheds light not only on their current uses but also on potential future directions and innovations.
Summary of Key Findings
- Molecular sieves are crucial for selective adsorption, enabling the separation of molecules based on size and shape.
- Their varied applications across industries highlight their versatility: from water purification to catalytic processes in chemical production.
- Recent advancements in materials science have led to novel designs that promise enhanced performance and specific functionality, catering to the growing demands of diverse fields.
"Molecular sieves offer a glimpse into the innovative materials driving forward our understanding of selective adsorption capabilities."
Methodologies Employed
The understanding of molecular sieves hinges on comprehensive methodologies that integrate theoretical and experimental approaches.
- Theoretical Studies: These often involve computational simulations to predict adsorption behavior and molecular interactions. Tools such as molecular dynamics and Monte Carlo simulations shed light on how these materials function on a microscopic level.
- Experimental Approaches: In laboratories, researchers characterize the sieves using spectroscopic methods and gas adsorption tests. Techniques such as BET (Brunauer-Emmett-Teller) analysis provide valuable insights into surface area and porosity, fundamental characteristics determining efficacy.
This combination of methods allows researchers to deepen their understanding of molecular sieves, ensuring that the knowledge contributed is both robust and applicable to real-world challenges.
Prelims to Molecular Sieves
Molecular sieves play a pivotal role in various fields, contributing significantly to advancements in technology and science. Understanding this topic is crucial because it encapsulates principles that are foundational to fields like environmental remediation, catalysis, and pharmaceuticals. Molecular sieves are employed to separate molecules based on size and shape, a process that highlights their utility across industries.
Examining the intricacies of molecular sieves provides valuable insight into how they function and the subsequent impacts on processes from refining oil to capturing carbon emissions. As industries grow and adapt to modern challenges, the role of these sieves becomes ever more relevant, spotlighting their importance not just in theory but also in practical applications.
With an awareness of these elements, the reader can appreciate the depth and breadth of molecular sieves in scientific research and innovation.
Definition and Overview
A molecular sieve is essentially a material with tiny, uniform pores that allow certain molecules to pass while blocking others, akin to a highly selective filter. Typically made from zeolites or synthetic structures, these sieves can be tailored to select for specific sizes or types of molecules, which enhances their utility in a variety of applications.
At their core, molecular sieves exploit the principle of adsorption, a process where molecules adhere to surfaces in a way that is dependent on the size and polarity of the molecules involved. This specificity makes molecular sieves invaluable for operations such as drying gases, separating isotopes, and refining petrochemicals.
Historical Context
The history of molecular sieves can be traced back to the discovery of natural zeolites, mineral structures known for their ability to absorb and exchange cations. Early uses centered on water purification and ion exchange. However, the field took a substantial leap when researchers began to synthesize these materials in the late 20th century. This synthesis paved the way for an explosion of applications, from catalysis in the industrial sector to their use in advanced filtration systems.
The advancements in material science allowed for greater control over pore sizes and the introduction of new synthetic materials, like silicalite and aluminosilicates. As industries evolved, so too did the methods for producing and utilizing molecular sieves, marking a significant chapter in both academic research and commercial applications.
By discussing the historical evolution alongside the definition, a clearer perspective emerges on how molecular sieves became integral to diverse processes and industries.
Classification of Molecular Sieves
Classifying molecular sieves is a fundamental aspect of understanding their functionality and applications. The differences between natural and synthetic sieves not only inform their specific uses but also highlight the advancements in materials science that drive innovation. This classification plays a vital role in determining the effectiveness of molecular sieves in various sectors, from environmental remediation to pharmaceuticals. By categorizing sieves, we can better appreciate their mechanisms and select the right type for specific challenges.
Natural vs. Synthetic Sieves
Natural molecular sieves are typically found in nature, formed through geological processes. An example would be natural zeolites, which are mined from volcanic ash deposits. These sieves often have limited properties due to the variability in their formation, which can impact their selectivity and efficiency in applications. One of the advantages of natural sieves is their low cost and ecological footprint—they're often readily available and require minimal processing. However, their inconsistency can pose challenges in precise applications.
On the flip side, synthetic molecular sieves are engineered with specific properties tailored for particular applications. They provide a level of control over characteristics like pore size, shape, and chemical composition. For instance, synthetic zeolites can be manufactured to meet exact requirements in gas separation processes. Their design flexibility often makes them preferable for high-stakes industries, where precision is crucial. Moreover, synthetic sieves can achieve higher purity levels and selectivity, which adds to their overall effectiveness. However, it’s important to note that the production of synthetic sieves can involve higher costs and may raise environmental concerns surrounding their synthesis methods.
Types of Synthetic Molecular Sieves
Synthetic molecular sieves can be further divided into various types, each with unique properties and applications. Among these, zeolites, silicalites, and aluminosilicates stand out as prominent categories, each boasting distinct characteristics that cater to different uses.
Zeolites
Zeolites are perhaps the most commonly used synthetic molecular sieves, known for their crystalline structure and well-defined porosity. Their primary feature is a three-dimensional framework of silica and alumina tetrahedra, creating channels and cavities that allow selective adsorption of molecules. This characteristic makes zeolites a popular choice in catalysis and gas separation because they can distinguish between molecules based on size and polarity. The advantages of using zeolites include high thermal stability and regeneration capabilities, which are vital in industrial processes. However, the complexity of their synthesis can lead to inconsistencies in performance, making them a double-edged sword in some applications.
Silicalite
Silicalite is a specific type of zeolite that contains only silicon oxide, presenting a porous structure with uniform pore sizes. The key characteristic of silicalite is its hydrophobic nature, which enables it to selectively adsorb nonpolar molecules, making it particularly beneficial for applications in petrochemical processes. Silicalite's unique feature lies in its high thermal stability and resistance to alkali and acid, although this also limits its ability to interact with polar compounds. This can be seen as a disadvantage in situations where a broad spectrum of adsorption is needed, but it enhances its effectiveness in specific environments, such as in the purification of hydrocarbons.
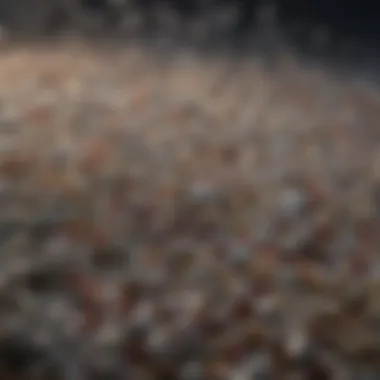
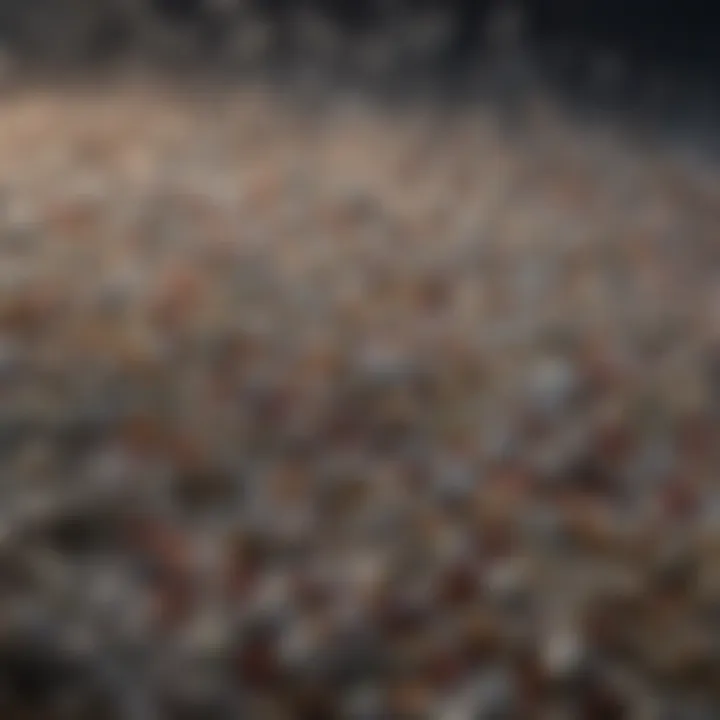
Aluminosilicates
Aluminosilicates are another class of synthetic molecular sieves that incorporate both silicon and aluminum in their structure. The presence of aluminum in aluminosilicates gives them distinct ion-exchange properties, which can be beneficial in various separation processes. A significant advantage of these materials is their ability to manipulate ionic interactions, aiding in applications like wastewater treatment and ion-exchange processes. However, their manufacturing process can be quite complex, and variations in aluminum content can lead to inconsistent performance, posing challenges in precise applications. This mix of benefits and limitations makes aluminosilicates worthy of attention in the realm of molecular sieving.
"The classification of molecular sieves is crucial as it informs their application, effectiveness, and efficiency in addressing modern challenges across various sectors."
Understanding these distinctions helps researchers and industry professionals choose the appropriate molecular sieve for their specific needs, ensuring optimal performance in real-world applications.
Principles of Molecular Sieving
The principles of molecular sieving lie at the heart of understanding how these materials function and why they are integral in a variety of applications. Key to this understanding are the mechanisms that dictate how molecules interact with the sieve structure, enabling selective separation based on size, shape, and chemical properties. This ability to act as an intricate filter is what lends molecular sieves their versatility in fields ranging from environmental remediation to pharmaceuticals.
Adsorption Mechanisms
The notion of adsorption is fundamental when discussing molecular sieves. Essentially, adsorption refers to the process by which molecules adhere to the surface of the sieve material. Understanding the specifics of this interaction is crucial for optimizing molecular sieve performance in real-world applications.
Physical Adsorption
Physical adsorption, often termed physisorption, is characterized by weak van der Waals forces. These interactions allow for reversible adhesion of molecules onto the sieve surface. One of the remarkable aspects of physical adsorption is how quickly it occurs; the molecules latch onto the sieve like a rubber stamp on ink, with no chemical bonds forming.
- Key characteristic: The predominant force here is related to the physical characteristics of molecules and surface areas.
- Why it's a popular choice: Its reversibility is a big win in many industrial processes, allowing for easy regeneration of the material after use. In applications like gas separation, this becomes particularly advantageous as it can boost efficiency without incurring hefty costs.
- Unique feature: Physical adsorption is often less energy-intensive, meaning less expenditure in both energy and resources is required to employ it.
- Advantages:
Easier material recovery.
Lower operational costs. - Disadvantages:
However, its effectiveness is often limited at elevated temperatures, where the kinetic energy of molecules can lead to reduced retention.
Chemical Adsorption
On the flip side, chemical adsorption is a process where molecules form stronger bonds with the surface of the molecular sieve. This interaction often involves covalent bonds and can lead to significant changes in the molecules themselves.
- Specific aspect: This type of adsorption generally results in more stable and longer-lasting interactions, offering consistent performance in certain applications.
- Why it's beneficial: The formation of these stronger bonds can enable more selective separation processes, particularly for complex mixtures where a high level of specificity is crucial, such as in catalysis.
- Unique feature: Chemical adsorption can be engineered to target specific reaction pathways which are essential in creating desired outcomes.
- Advantages:
Greater specificity in adsorption.
Better retention at higher temperatures. - Disadvantages:
On the downside, the energy involved in the adsorption process can lead to irreversible changes, thus complicating regeneration processes.
Pore Size and Selectivity
The dimensions of the pores in molecular sieves play a critical role in dictating selectivity. They act like keyholes, allowing only specific molecules to pass through while blocking others.
- Importance of pore size:
The size must be tailored for the type of separation you want to achieve, which can vary widely based on the application. - Selectivity: This term speaks to the chemical affinity of the sieve material for certain molecules over others. The interplay between pore architecture and the physical-chemical properties of the molecules being sieved defines effectiveness in applications ranging from gas separation to environmental cleanup.
In summary, the principles of molecular sieving hinge on the intricate dance between adsorption mechanisms and the architecture of the sieves themselves. Understanding the strengths and limitations of both physical and chemical adsorption, combined with the pivotal role of pore size and selectivity, lays the foundation for advancements in molecular sieve technology.
Production and Synthesis of Molecular Sieves
The production and synthesis of molecular sieves are central themes in understanding their role in various applications. This section delves into the methods that give rise to these materials and highlights their significance in ensuring that molecular sieves meet the required standards for various industrial and research purposes. The precise synthesis techniques influence the properties such as pore structure, selectivity, and chemical stability which dictate how these materials behave in specific applications. It is crucial to grasp how these methods work in order to appreciate the complexities and capabilities of molecular sieves in practice.
Methods of Synthesis
Hydrothermal Methods
Hydrothermal methods involve the use of high-temperature, high-pressure conditions to facilitate the crystallization of molecular sieves from a solution. This technique is pivotal, especially for creating zeolites, which are a subtype of molecular sieves known for their porous structure. One of the remarkable attributes of hydrothermal synthesis is its ability to produce materials with well-defined crystal morphologies and controlled sizes. This precision is significant, as the structural integrity directly affects the sieve’s performance.
What's more, this method is considered green in the sense that it uses water as the primary solvent, making it more environmentally friendly than many organic-based processes. The disadvantages, however, include the considerable time often needed for crystallization and potential issues regarding the scalability of the process. Lessons learned from this method contribute significantly to understanding molecular sieves better in labs and industries alike.
Sol-Gel Processes
On the other hand, sol-gel processes offer a different pathway for creating molecular sieves. This method typically begins with a solution that transforms into a gel-like state before ultimately forming a solid material. The key characteristic of sol-gel synthesis is its versatility; the process can be easily tailored to tweak the chemical composition and thus fine-tune the physical properties of the sieves. It allows for homogeneity at the molecular level, which can lead to more efficient adsorption properties and stability.
A unique feature of this technique is its capacity to incorporate various components within the silica framework, allowing for the manufacture of mixed molecular sieves. However, it may also present challenges like achieving a uniform distribution of pore sizes, which is vital for certain applications.
Characterization Techniques
Characterization is the bridge that links the production processes to the practical applications of molecular sieves. Understanding the structure and properties of these materials demands well-established techniques that can unveil the intricacies of molecular sieves.
X-Ray Diffraction
X-ray diffraction (XRD) is a prominent technique used in the characterization of molecular sieves, particularly for determining their crystallinity and phase purity. The essence of XRD lies in its ability to provide data on how atoms are arranged within a material, crucial for understanding how these sieves will perform in real-world scenarios. By analyzing the diffraction pattern, researchers can deduce important parameters such as lattice dimensions and symmetry.
It's a mainstay technique because it’s relatively quick and non-destructive, thus allowing researchers to obtain results without altering the samples. However, while XRD provides a wealth of information, it may not always be sufficient for analyzing all forms of molecular sieves—especially when it comes to their surface characteristics.
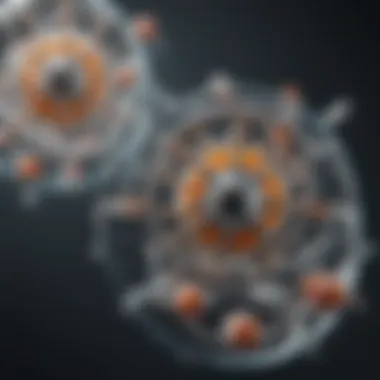
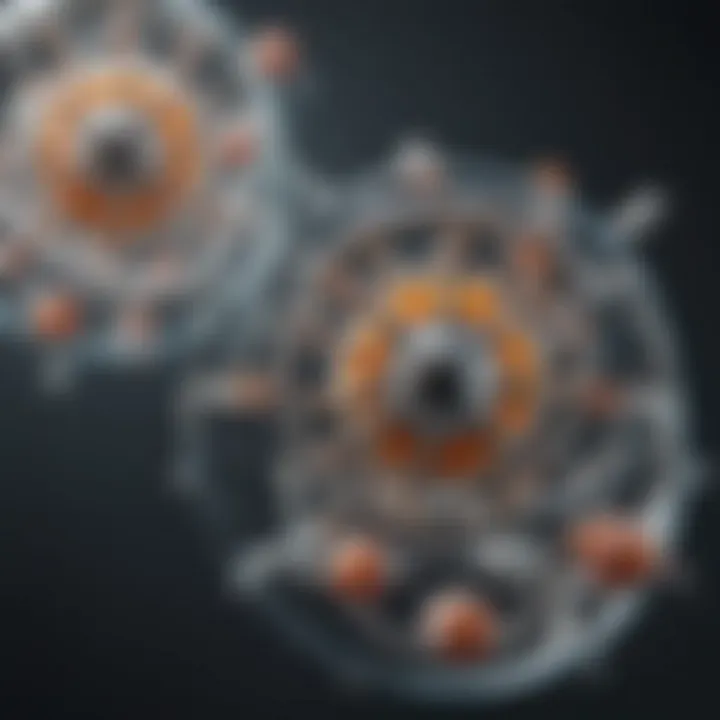
Scanning Electron Microscopy
In contrast, scanning electron microscopy (SEM) stands out by providing direct visual insight into the morphology of molecular sieves. This technique generates high-resolution images, allowing for a detailed examination of the surface structure. At a glance, the topographical and compositional information gleaned from SEM helps to determine how the molecular sieve might interact with different molecules during their filtering processes.
Despite the valuable insights SEM offers, one must keep in mind its limitations in terms of depth of field and the necessity to conduct the analysis in a vacuum. Thus, while it provides critical data about surface features, it should be employed alongside other methods like XRD for a comprehensive understanding.
The careful synthesis and characterization of molecular sieves are essential in optimizing their performance across a multitude of applications, from gas separation to environmental remediation. These processes and techniques not only illuminate the path for future advancements but also ensure that the sieves developed meet the ever-evolving standards of technology.
Applications of Molecular Sieves
The versatility of molecular sieves is truly impressive, finding a home in various fields ranging from industrial processes to environmental science. Understanding the applications of these fascinating materials sheds light on their significance and functional prowess. Each application harnesses the selective adsorption properties of molecular sieves, leading to enhanced efficiency and effectiveness in numerous processes.
In Gas Separation
Molecular sieves play a crucial role in gas separation processes. They can discriminate gases based on size and shape due to their well-defined pore structures. For instance, in the effective separation of nitrogen from oxygen, zeolites are often utilized. This sort of separation is essential in industries where the purity of gases matters, such as in the production of high-quality steel or oxygen for medical applications.
Additionally, molecular sieves enable the capture of contaminants from natural gas streams. They help in removing heavier hydrocarbons, thus preventing pipeline blockages and enhancing overall system efficiency. This filtration capacity not only boosts operational efficiency but also supports environmental sustainability by minimizing emissions.
In Catalysis
Catalysis is another domain where molecular sieves shine brightly. Their porous structures provide ideal support for catalysts, enhancing reaction rates while maintaining selectivity. One striking example is using molecular sieves in the conversion of renewable feedstocks into biofuels. For instance, zeolites can be employed to transform agricultural waste products into valuable energy resources.
Innovative usage includes:
- Acid catalysis: The acidic properties of certain molecular sieves can promote reactions like cracking or isomerization.
- Enzymatic activity: They can support enzymes in reactions needing specific environmental conditions, optimizing efficiency and yields
By integrating molecular sieves into these systems, industries can achieve better yields with reduced environmental impact, highlighting their importance in sustainable practices.
In Environmental Remediation
In the context of environmental remediation, molecular sieves serve as invaluable tools. Their capability to adsorb pollutants from water and air is noteworthy. For instance, when dealing with heavy metal contaminants in wastewater, molecular sieves can efficiently capture and remove these harmful substances, safeguarding both ecosystem and human health.
"Molecular sieves are like nature's sponge, soaking up trouble and leaving clean water behind."
Moreover, in air filtration applications, these materials can help remove volatile organic compounds, contributing significantly to cleaner indoor environments. Their role in pollution control emphasizes the need for advanced materials in fighting today’s environmental challenges.
In Pharmaceuticals
The pharmaceutical industry has greatly benefitted from the applications of molecular sieves. They facilitate the purification of active pharmaceutical ingredients (APIs) by selectively adsorbing unwanted byproducts during synthesis. This leads to high-purity compounds, which are critical for the efficacy and safety of medications.
Furthermore, molecular sieves can also improve drug stability. They can assist in formulating certain products where controlled release of the active ingredient is essential. For example, when developing extended-release formulations, these sieves can regulate the diffusion of the drug into the body, ensuring sustained therapeutic effects.
In summary, from air and water purification to enhancing chemical processes and improving drug formulations, molecular sieves are integral in driving forward innovation, efficiency, and sustainability across various sectors.
Recent Advances in Molecular Sieve Technology
In the realm of molecular sieves, innovations continue to emerge, reshaping not only the materials themselves but also their applications across various industries. The exploration of recent advances in molecular sieve technology is pivotal for understanding how these materials are evolving to meet the demands of modern science and industrial applications. As we delve into this topic, it is essential to recognize the specific elements that are driving these advancements, the benefits they offer, and the considerations that accompany their integration into practical usage.
Nanostructured Molecular Sieves
Nanostructured molecular sieves represent a remarkable leap forward in the design and application of molecular sieves. These materials, which have structures at the nanometer scale, exhibit enhanced properties compared to their larger counterparts. One prominent feature of nanostructured sieves is their increased surface area, which significantly improves their adsorptive capacity. This quality becomes evident in applications requiring precise separation processes, such as in gas purifications where even the smallest impurities can influence performance.
The benefits of adopting nanostructured molecular sieves are manifold:
- Improved Efficiency: With a larger surface area and more active sites, these sieves can dramatically enhance reaction rates and selectivities in catalytic processes.
- Versatility: Nanostructured sieves can be tailored for specific applications, allowing for a wide range of functions from gas storage to environmental cleanup.
- Enhanced Stability: Their smaller sizes often lead to improved stability under extreme conditions, making them ideal for more demanding industrial applications.
However, working with nanostructured materials isn't without its challenges. The synthesis of these sieves requires precise control conditions, and there is an increased need for advanced characterization techniques to ensure quality and consistency. Moreover, cost considerations can also be a hurdle in scaling up these materials for commercial use, requiring a balance between innovation and practicality.
Smart Molecular Sieves
Turning our attention to another exciting frontier, smart molecular sieves are beginning to play an increasingly important role in various fields. Unlike traditional sieves, smart variants are designed with responsive functionalities. They can change their pore size, surface properties, or adsorption behavior in response to external stimuli such as temperature, pH, or chemical composition. This adaptability opens the door to a wide array of innovative applications.
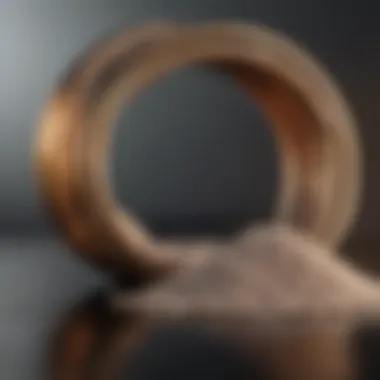
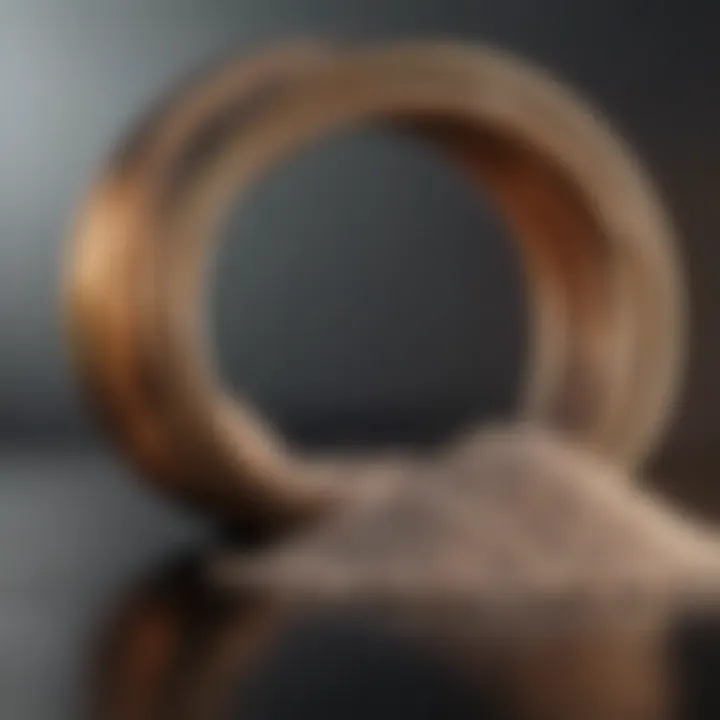
Key characteristics of smart molecular sieves include:
- Responsive Mechanisms: The ability to alter their structural characteristics on demand increases their effectiveness in real-time applications. For example, smart molecular sieves can be engineered to trap specific pollutants when environmental conditions dictate.
- Enhanced Recovery and Recycling: These materials can potentially recover valuable resources, such as precious metals from wastewater, and adjust their properties to optimize the extraction process dynamically.
- Long-term Viability: The operational flexibility afforded by smart molecular sieves leads to improved longevity and efficiency, making them a worthy investment for industries focused on sustainability.
Despite their promising advantages, challenges persist. The complexity of designing systems that can seamlessly integrate smart sieves into current technologies requires ongoing research. There’s also a need for clear regulatory frameworks to ensure safety and efficiency in their application.
"The advent of smart molecular sieves reflects a significant shift towards more sustainable and efficient materials capable of responding to the dynamic needs of industries."
Challenges and Limitations
Molecular sieves play a significant role across various fields, yet they are not without their challenges and limitations. It's crucial to grasp these aspects when delving into their applications and innovations. Understanding these hurdles not only informs researchers and industry professionals about the current limitations but also highlights areas ripe for advancements. Among these are the stability issues that affect the performance of these materials, and the constant scrutiny over cost-effectiveness. Addressing these challenges is key to unlocking the full potential of molecular sieves and ensuring they remain a cornerstone in modern technology.
Stability Issues
One notable challenge for many molecular sieves is their stability under varying conditions. In certain settings, the structures of molecular sieves can experience degradation. Thermal stability is one major concern; extreme heat may lead to a collapse of the pore structure, severely affecting their sieving capabilities. For instance, zeolites are typically stable at high temperatures, but prolonged exposure to excessive heat can sometimes weaken their crystalline framework.
Another factor that comes into play is chemical stability. Some molecular sieves can be sensitive to specific reagents. For instance, exposure to strong acids or bases can result in structural changes that reduce their effectiveness. A particular material, like silicalite, may withstand acidic environments to some extent, yet over time, the cumulative impacts of exposure can lead to diminished performance.
"Stability is not merely a property; it’s the bedrock that influences the lifespan and efficacy of molecular sieves in practical applications."
Additionally, absorption of moisture can affect stability. Hydrated molecular sieves may display altered selectivity or reduced adsorption capacities, complicating their use in precise applications. Therefore, engineers and researchers continuously explore methods to enhance the stability of these materials, leading to the development of more resilient molecular sieves tailored for specific conditions.
Cost-Effectiveness
Cost-effectiveness is another pressing topic in the discourse on molecular sieves. The synthesis of some advanced molecular sieves, particularly synthetic varieties, often involves intricate processes and raw materials that can drive up production costs. While the benefits in performance and specificity can be substantial, budget constraints in various industries can limit the wider adoption of these cutting-edge molecular sieves.
Labor-intensive synthesis methods can inflate the price, and achieving the right balance between performance and economy is a recurring challenge. For example, although zeolites offer excellent adsorption characteristics, they may not always be the most budget-friendly choice for certain large-scale applications. This aspect prompts industries to seek alternatives that provide a more cost-effective solution without significantly compromising efficacy.
Moreover, maintaining existing molecular sieves without incurring additional costs presents a dilemma. Regular maintenance might be required to optimize performance, leading to ongoing expenses not accounted for during the initial acquisition.
In summary, both stability issues and cost-effectiveness present substantial challenges to the widespread application of molecular sieves. As researchers continue to innovate and refine synthesis methods, future directions in molecular sieve technology will likely aim to enhance stability while reducing overall production costs, ensuring these invaluable materials remain integral to myriad industries.
Future Directions in Molecular Sieve Research
As we step into a new era of molecular technology, the future of molecular sieve research stands at an intersection of scientific innovation and pressing global needs. The importance of exploring future directions in this field stems not only from the potential improvements in efficiency but also from the urgent environmental challenges we face today. Researchers and industry leaders are now prioritizing the design and development of molecular sieves that can cater to both functionality and sustainability. Understanding these future trends is critical for leveraging the full potential of molecular sieve technologies within various sectors.
Innovative Material Design
The drive for innovative material design in molecular sieves is a cornerstone of future research. One key focus is on modulating the pore structures of these materials to enhance their adsorption capabilities. For instance, scientists are experimenting with hybrid materials that combine organic and inorganic components, leading to sieves that exhibit enhanced selectivity for certain molecules. By engineering these materials at the molecular level, researchers can create materials that not only target specific pollutants in environmental applications but also improve catalytic processes in industrial settings.
Another important aspect is the development of functionally diverse materials. This could mean creating molecular sieves that are not only effective adsorbents but also possess additional functionalities, such as photocatalysis or electrochemical activity. Such innovations could greatly expand the applications of molecular sieves in emerging fields like renewable energy and carbon capture.
"The future of molecular sieves lies in the ability to design materials that adapt and respond to their environments, not just filter them."
The exploration of biodegradable materials is also gaining traction. By using bio-based feedstocks for sieve production, researchers can contribute to a circular economy, minimizing waste and reducing reliance on nonrenewable resources.
Greener Synthesis Processes
Greener synthesis processes are an essential component of future directions in molecular sieve research. Traditional methods of synthesizing molecular sieves often involve the use of harsh chemicals and high-energy inputs, leading to environmental concerns and increased costs. The shift toward more sustainable methodologies is not just an afterthought; it’s becoming a necessity.
One promising avenue is the implementation of solvent-free synthesis techniques. These methods significantly reduce chemical waste and energy consumption. For instance, researchers are exploring mechanochemical synthesis, where milling methods alter molecular structures under mechanical forces without the need for solvents. This approach not only lessens the environmental footprint but can also lead to unique material properties.
Moreover, the adoption of green chemistry principles in the synthesis of molecular sieves can enhance their appeal in industry. Utilizing safer reagents and designing processes that minimize toxic byproducts are some measures that researchers are considering. Innovations such as bio-inspired synthesis—where processes mimic nature's efficiency—could pave the way for developing molecular sieves that are both effective and eco-friendly.
Closure
In closing, the exploration of molecular sieves is not merely an academic endeavor but a vital journey into their multifaceted roles in industry and environmental solutions. These materials are essential for achieving efficient separation processes, catalyzing reactions, and remediating pollutants. Their significance stretches well beyond laboratory confines and crosses into domains such as pharmaceuticals, where they help bring forth life-saving drugs through their selective properties.
Summary of Key Points
- Definition and Function: Molecular sieves are materials with pores that selectively allow certain molecules to pass while blocking others. This selective permeability is critical in various applications, from gas separation to environmental cleanup.
- Diverse Applications: We've seen how molecular sieves serve pivotal roles in many industries, including catalysis, pharmaceuticals, and environmental remediation. Each application taps into their unique structural and chemical properties to solve real-world problems.
- Technological Advances: Recent strides in molecular sieve technology, such as the development of nanostructured and smart molecular sieves, point to a promising future where tailor-made materials can meet even more specific challenges with precision.
- Challenges to Address: Despite their advantages, molecular sieves face challenges that hinder their broader adoption, including stability and cost-effectiveness. Acknowledging these hurdles is crucial for future research.
Implications for Future Research
The future of molecular sieve research is exciting and ripe with opportunities. As industries grapple with issues like pollution and resource scarcity, the demand for effective separation processes only intensifies. Future research directions may include:
- Innovative Material Design: Developing new sieve materials with enhanced properties such as increased stability and selectivity could pave the way for advances in atmospheric and waste control technologies.
- Greener Synthesis Processes: The push towards sustainability cannot be understated. Researchers are likely to focus on more environmentally friendly synthesis methods, reducing waste and energy consumption in the manufacturing of molecular sieves. This aligns with global trends toward greener technologies.
Research in this field promises not only to refine existing applications but also to create new avenues for molecular sieves in fields such as nanotechnology and biomedicine. As advancements continue, our understanding of molecular sieves will deepen, potentially unlocking new solutions to some of the most pressing issues of our time.