Exploring Light Chambers: Principles and Applications
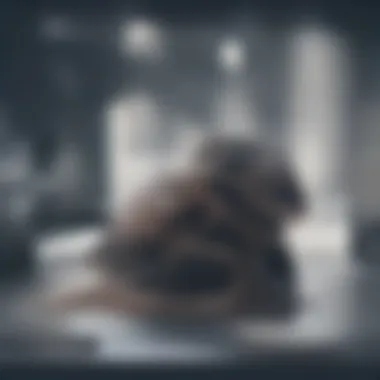
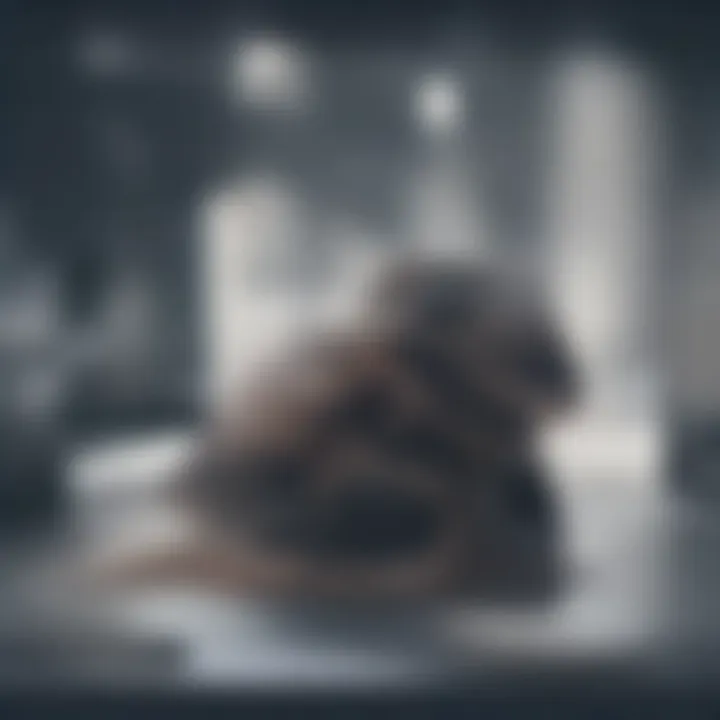
Intro
Light chambers represent a fascinating intersection of physics and technology. These systems harness the properties of light to achieve specific objectives across various scientific fields. Their intricate designs and diverse functionalities mark them as essential tools in todayβs research landscape. Understanding light chambers goes beyond mere curiosity; it is fundamental to grasping advancements across environmental science, biomedical research, and materials science.
The principles underlying light chambers are rooted in photonics, which studies the behavior of light. This article aims to unpack these principles, providing insights into how light can be manipulated and utilized. Furthermore, it will delve into the design strategies employed in constructing light chambers and their significant applications.
By elucidating these aspects, this article will cater to students, educators, and professionals eager for detailed knowledge and original insights into this important field.
Prelude to Light Chambers
Light chambers represent a significant intersection of physics and practical applications. Understanding their structure and functionality is crucial for advancements in numerous scientific fields. Light chambers harness various properties of light to achieve specific outcomes, ranging from environmental measurement to biomedical imaging. Their relevance has grown in recent years, as the demand for accurate data and efficient technology increases.
Definition and Overview
A light chamber can be defined as a controlled environment where light is manipulated to observe or measure specific phenomena. It typically consists of optical components that facilitate the interaction of photons with matter. This interaction can yield valuable insights in various disciplines, including environmental science, mechanics, and medical research.
The core purpose of a light chamber is to enable precise experiments by isolating light phenomena from external variables. These chambers can vary in complexity, from simple setups for educational purposes to sophisticated systems utilized by professionals. Light chambers ultimately allow for an experimental approach that illuminates the subtleties of how light interacts with different materials.
Historical Context
The study of light has deep historical roots, tracing back to ancient civilizations with theories on optics and vision. However, the development of light chambers as we know them began in the 19th century. Early optical instruments such as prisms and spectrometers laid groundwork for more intricate designs.
In the 20th century, advancements in technology propelled the field of optics. The invention of lasers and photonics increased possibilities for light chambers. Researchers began to develop specific applications of light chambers to monitor environmental changes and conduct biomedical research.
As technology continues to evolve, so do light chambers. They now serve multifunctional roles across various sectors, driving innovation and expanding our understanding of the physical world.
"The use of light as a probe for understanding the universe is not merely a scientific pursuit; it is an essential tool for comprehending and addressing environmental and health challenges."
Scientific Principles Behind Light Chambers
The scientific principles behind light chambers are crucial in understanding their design, functionality, and diverse applications. Light chambers operate based on fundamental properties of light and their interaction with matter. Recognizing these principles enables better design for specific applications and facilitates innovations in various fields, such as environmental monitoring and biomedical research.
Properties of Light
Wave-Particle Duality
Wave-particle duality is a fundamental concept of quantum mechanics, asserting that light exhibits both wave-like and particle-like properties. This duality is significant because it helps explain how light can be manipulated in light chambers. One key characteristic is that when light behaves as a wave, it can cause interference patterns, which are essential in various optical applications.
Wave-particle duality is particularly relevant to this article as it highlights the versatility of light. By understanding this property, researchers can develop light chambers that maximize the benefits of these dual characteristics. The unique feature of wave-particle duality is its application across numerous technologies, such as lasers and photonics.
However, there are disadvantages. For instance, while wave behavior allows for phenomena like diffraction, it also complicates calculations and predictions in certain scenarios. Thus, while the dual nature of light is beneficial, it also poses challenges in practical applications.
Electromagnetic Spectrum
The electromagnetic spectrum represents the range of all types of electromagnetic radiation, from radio waves to gamma rays. This spectrum is crucial for defining how light interacts with different materials in light chambers. A key characteristic of the electromagnetic spectrum is its classification of waves by wavelength and frequency, which influences their properties and interactions.
In terms of relevance to this discussion, the electromagnetic spectrum allows researchers to select specific wavelengths for targeted applications. For example, ultraviolet light can be utilized for sterilization processes, while infrared light can aid in thermal imaging.
The unique feature of the electromagnetic spectrum is its wide variety of wavelengths that can be harnessed for diverse technologies. This adaptability enhances the potential applications of light chambers. Nonetheless, working within the electromagnetic spectrum necessitates careful consideration of material properties, as some wavelengths may be absorbed rather than transmitted, which could limit the effectiveness of the system.
Photon Interaction with Matter
Absorption
Absorption refers to the process where photons are taken up by matter, resulting in energy transfer. This process is vital in many light chamber applications, especially for sensors. A key characteristic of absorption is its dependency on the wavelength of light and the material's properties. This characteristic is crucial in environmental monitoring tasks, as it allows for the detection of specific substances.
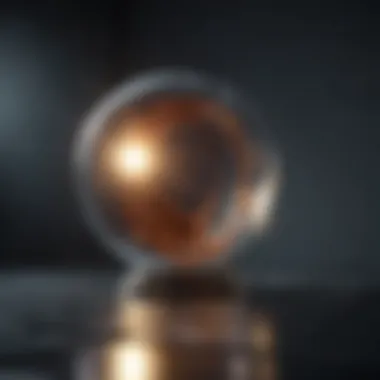
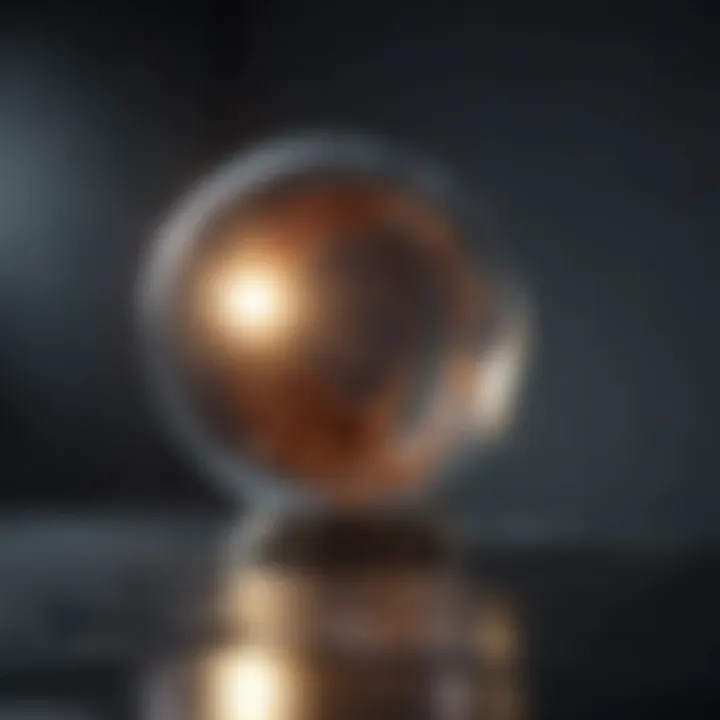
Absorption is beneficial because it enables the selective detection of various pollutants or biomarkers in different applications. For instance, sensors that rely on light absorption are used in pollution monitoring systems. The unique feature of absorption is its ability to provide quantitative data about sample concentrations.
However, energy losses occur during absorption, which limits the efficiency of light transmission. This is especially a concern in long-range applications, where signal strength can diminish considerably.
Reflection
Reflection is the change in direction of light when it encounters a reflective surface. This aspect is essential in light chambers as it enables the manipulation of light pathways. A notable characteristic of reflection is the law of reflection, where the angle of incidence equals the angle of reflection. This property is fundamental for designing optical components in light chambers.
Reflection is particularly advantageous as it allows for the reuse of light within chambers, increasing overall system efficiency. The unique feature here lies in the ability to direct light precisely towards sensors or detectors, enhancing measurement accuracy.
However, not all materials reflect light effectively. Imperfections on surfaces can scatter light, introducing errors in measurements and reducing the system's reliability.
Refraction
Refraction occurs when light passes from one medium to another, causing a change in speed and direction. This principle is critical for lens designs used in light chambers. A critical characteristic of refraction is described by Snell's law, which relates the angles of incidence and refraction to the indices of refraction of both media.
Refraction is beneficial as it enables the design of optical devices that can focus or disperse light. A unique feature of refraction is its ability to impact how light behaves when entering different materials. This has implications for how light chambers can be engineered to suit specific applications, such as imaging or sensing tasks.
However, refraction can lead to distortions in image quality if not carefully managed. This challenge necessitates precision in engineering and material choice to ensure optimal performance in light chambers.
Design and Construction of Light Chambers
The design and construction of light chambers are critical in understanding their functionality and effectiveness. These chambers are not merely vessels for containing light; their architecture and materials play a significant role in their performance in various applications. An effective design improves efficiency, accuracy, and reliability, which are essential in fields ranging from environmental science to biomedical research.
Materials Utilized
Optical Components
Optical components are vital in the creation of light chambers. They include lenses, mirrors, and prisms that manipulate light pathways. The key characteristic of optical components is their ability to precisely control and direct light. This control is essential for applications such as imaging and sensing. The precision of these components contributes to the overall effectiveness of light chambers in capturing accurate data.
One unique feature of optical components is their ability to filter light based on wavelength. This characteristic can be advantageous in experiments where specific light wavelengths are needed. However, high-quality optical components can be expensive, which is a disadvantage for budget-conscious research projects.
Structural Materials
Structural materials are also crucial in the design of light chambers. Common materials include glass and polymers, which offer transparency while providing structural integrity. The main characteristic of structural materials is their ability to withstand environmental stresses without compromising the function of the light chamber. Selecting suitable structural materials ensures the durability of the chamber under various conditions.
A unique feature of structural materials like tempered glass is that it offers enhanced resistance to thermal strains. This makes it particularly beneficial in applications where temperature fluctuations are a concern. On the downside, heavier structural materials may complicate the installation and transport of the light chambers, presenting a tangible trade-off in certain scenarios.
Engineering Considerations
Thermal Management
Thermal management is an essential aspect of designing light chambers. Effective thermal regulation prevents overheating, which can affect the performance of sensitive components. The key characteristic of a good thermal management system is its ability to dissipate heat efficiently while maintaining a stable operating environment. This feature is particularly useful for light chambers utilized in long-term studies.
Unique thermal management techniques, such as heat sinks and ventilation, provide benefits by extending the operational lifespan of the equipment. However, integrating these systems can increase the complexity and cost of the design, potentially limiting accessibility for smaller labs.
Light Management
Light management is another crucial engineering consideration. This aspect deals with how light is harnessed and directed within the chamber. A key characteristic of effective light management systems is their capacity to enhance light intensity and uniformity across the target area. Achieving optimal light conditions is crucial, especially in applications focused on imaging and detection.
The integration of advanced light management systems can lead to improved performance and data accuracy. Nevertheless, these systems can be complex to install and calibrate, requiring additional training and expertise from users. This can pose a challenge for organizations with limited technical resources.
Applications of Light Chambers
Applications of light chambers are diverse and multidimensional. They play a significant role across several fields, enhancing both research and practical implementations. Their ability to manipulate light makes them ideal for applications in environmental science, biomedical research, and materials science. The integration of light chambers into these domains provides a depth of analysis and methods that were previously unattainable. Understanding their role helps unlock potential advancements that can lead to impactful solutions in various industries.
Environmental Science
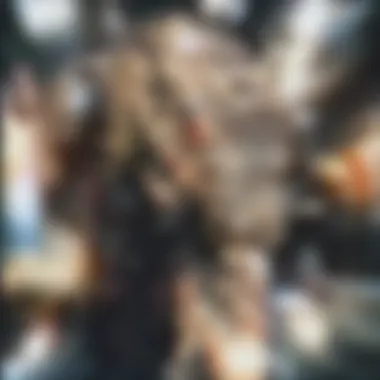
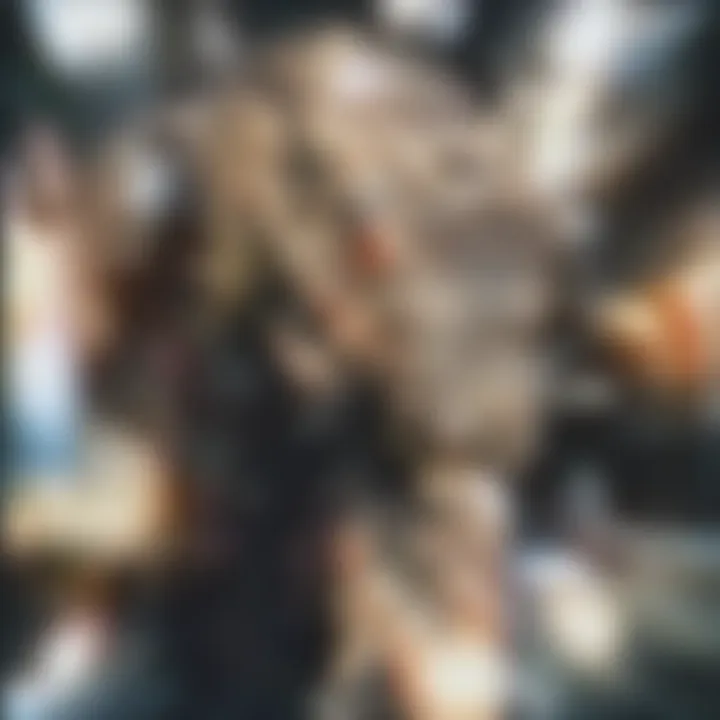
Pollution Monitoring
Pollution monitoring represents a critical area where light chambers are invaluable. Through the use of light-sensitive sensors, these chambers can detect changes in light caused by various pollutants. This real-time monitoring enables researchers to track contamination levels in air and water, leading to timely interventions. A key characteristic of pollution monitoring via light chambers is its precision. This method stands out because it provides accurate and continuous data, making it a reliable choice for studies related to environmental protection.
However, there are unique features to consider. For instance, while light chambers can detect a wide range of pollutants, their effectiveness may vary depending on the specific environmental conditions. There is also a potential limitation related to resolving high levels of interference, which can hinder effectiveness in certain scenarios. Nevertheless, the advantages of this technique in accurately monitoring pollution contribute significantly to environmental science.
Remote Sensing
Remote sensing is another area where light chambers shine in their application. By using various wavelengths of light, researchers can gather information about the Earth's surface, vegetation, and water bodies without physical contact. This method allows for extensive collection of data over large areas, which is crucial for environmental assessments. The ability to gather data from a distance makes remote sensing highly efficient.
The unique feature of remote sensing is the utilization of multispectral and hyperspectral imaging. This characteristic facilitates detailed analysis of various materials and conditions. However, it is essential to acknowledge that remote sensing can sometimes face challenges related to data interpretation and atmospheric interference. Despite these challenges, the benefits of remote sensing in environmental science are substantial, offering insights that drive policy and conservation efforts.
Biomedical Research
Imaging Techniques
In biomedical research, imaging techniques powered by light chambers have transformed diagnostic capabilities. These methods, such as fluorescence microscopy, allow scientists to visualize cellular structures with unparalleled precision. A key characteristic of imaging techniques in light chambers is their ability to use specific wavelengths of light to excite fluorophores. This leads to high-resolution images that become instrumental in understanding complex biological processes.
The unique feature here involves the capability to conduct live-cell imaging. This aspect allows researchers to observe dynamic processes in real-time. However, a limitation associated with such techniques relates to phototoxicity, where high-intensity light can damage cells. Despite this, the advantages in providing detailed imaging make it a popular choice within biomedical research.
Cell Culture Studies
Cell culture studies benefit immensely from light chambers, particularly for assessing cellular responses to various stimuli. Light chambers allow researchers to control and manipulate light exposure during experiments to test different conditions. A distinctive characteristic of this application is the control over environmental variables. Researchers can maintain consistent conditions, crucial for obtaining reliable experimental results.
The ability to precisely modify and measure light conditions serves as a unique feature. However, one must consider the potential drawback of equipment costs and complexity in setting up such systems. Despite this, the advantages of conducting thorough cell culture studies contribute richly to our understanding of cellular processes.
Materials Science
Photonic Crystals
In the field of materials science, photonic crystals are significant materials that employ light chambers. These structures can manipulate light to create applications in telecommunications and sensors. A defining characteristic of photonic crystals is their capacity to control light propagation through photonic band gaps. This allows for advanced functionalities in electronic devices.
A unique feature is the design flexibility these materials offer, enabling the creation of customized optical responses. Nevertheless, photonic crystals do come with challenges such as fabrication complexities and the need for precise geometric design. Regardless, their advantages in improving device performance make them an essential topic in materials science.
Nanomaterials
Nanomaterials serve as another vital application within materials science linked to light chambers. Their properties can be exceptionally altered at the nanoscale to enhance optical performance. A critical characteristic of nanomaterials is their large surface area relative to volume, which enhances interaction with light. This results in unique optical phenomena that can be exploited in various applications, such as drug delivery systems and solar cells.
The ability to engineer materials at the nanoscale is a powerful feature. However, challenges remain regarding the scalability of production and potential health effects of nanomaterials. Nonetheless, the contributions of nanomaterials to advancements in materials science are undeniably impactful.
Innovative Technologies in Light Chambers
Innovative technologies play a crucial role in enhancing the functionality and efficiency of light chambers. These technologies allow for greater precision in experiments and applications involving light. The integration of advanced sensor systems and connectivity options notably expands the potential of light chambers. This section focuses on recent advancements and future prospects that shape the landscape of this fascinating subject.
Recent Advancements
Smart Sensors
Smart sensors in light chambers represent a significant leap in capability. These devices are designed to capture data with high accuracy and are known for their ability to adapt to changing environments. The main contribution of smart sensors is their capacity to provide real-time feedback. This feature is essential for applications that require immediate response based on light measurements.
One key characteristic of smart sensors is their wireless connectivity. This allows for easy data transmission to external devices for analysis. The benefits of using smart sensors include improved data reliability and the ability to monitor conditions remotely. However, integrating these sensors may pose challenges, including higher costs and potential complexity in setup.
Integration with IoT
The integration of light chambers with Internet of Things (IoT) technology is reshaping data collection and analysis processes. This integration ensures that light chambers are not merely isolated systems but contribute to a broader network of devices. The primary advantage is the enhanced ability to share information across platforms. This fosters better collaboration and efficiency in research and applications where multiple data sources are crucial.
A notable characteristic of this IoT integration is the streamlined user interface that allows for easier monitoring and control. Benefits include better scalability and the ability to analyze large datasets in real-time. On the downside, reliance on internet connectivity can lead to vulnerabilities, and not all IoT systems are secure. Consideration of data safety and integrity remains a vital concern in this context.
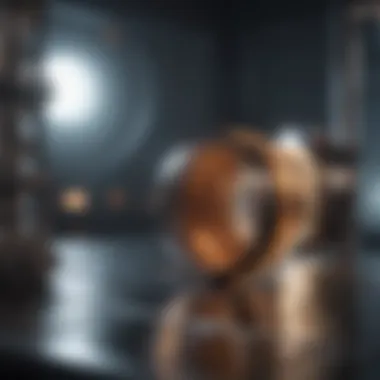
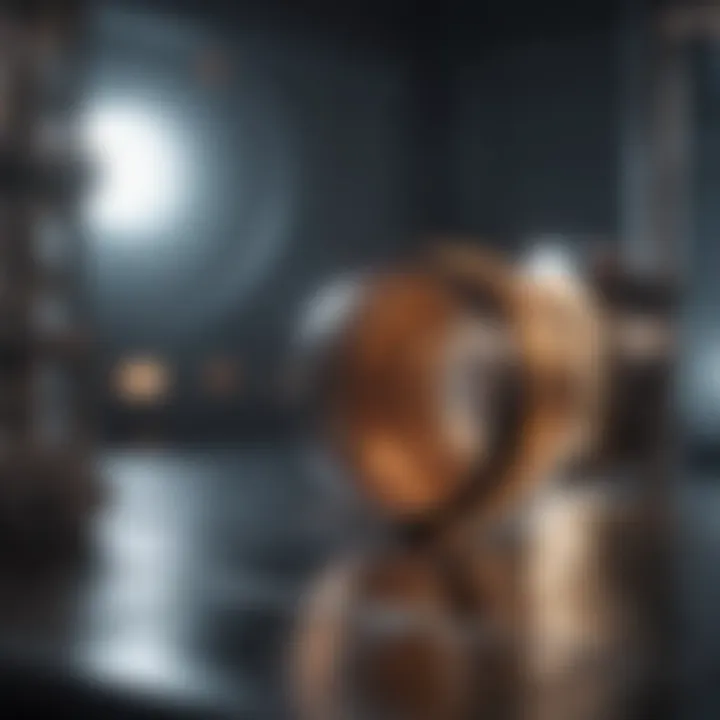
Future Prospects
Emerging Applications
Emerging applications of light chambers offer exciting possibilities. As technology advances, these chambers are expected to find use in novel fields such as autonomous vehicles and smart agriculture. The ability to detect and analyze light in diverse environments is key to these applications.
One key aspect of these emerging applications is their adaptability. Whether for precision farming or environmental monitoring, light chambers can be customized to meet specific operational needs. The advantages include improved efficiency and data collection in real-time. Nevertheless, entering new markets may require substantial investment and research to adapt existing technology adequately.
Research Directions
Looking at future research directions, there is a clear need for innovations that address current limitations. Research will likely focus on enhancing the materials used in light chambers to improve durability and performance. The key characteristic here is the ongoing quest for optimization in design and function.
Moreover, the focus on sustainability in technology makes developing eco-friendly materials increasingly important. Such innovations will not only contribute to efficiency but also ensure compliance with environmental standards. The significant advantage of pursuing these research directions is the potential for significant breakthroughs in various fields. However, the complexity of such research can often lead to longer development times.
Advances in light chamber technology promise to revolutionize multiple fields, offering new insights and greater efficiency.
Challenges and Limitations
Understanding the challenges and limitations of light chambers is essential for anyone involved in their design and application. These factors can significantly influence the performance and effectiveness of light chambers across various fields. Identifying and addressing these challenges allows for improved designs and enhances their usability in scientific research and industrial applications.
Technical Difficulties
Technical difficulties present a major hurdle in the development of light chambers. One challenge is achieving the desired optical performance. For instance, any minor misalignment of optical components can lead to significant energy loss or distortions in measurements. These complications require rigorous calibration and quality control during the assembly phase.
Moreover, the interaction between light and the materials used to construct the chambers can introduce complexities. Light chambers must be designed to optimize the transmission and reflection of light, necessitating the use of advanced materials that can withstand environmental stresses while maintaining their optical clarity.
There are also issues related to the integration of sensors and electronic controls into light chambers. Ensuring these components function effectively without interfering with light measurements can be difficult. For example, electromagnetic interference from components can lead to inaccurate readings.
Material Limitations
Material limitations are another significant challenge when designing light chambers. The choice of materials directly affects both durability and optical performance. Some materials may not be transparent enough for specific wavelengths of light, limiting their application in experiments or industries requiring precision measurements.
For instance, while glass is often considered a standard in optical applications, there are scenarios where specialized polymers or coatings are necessary to enhance light transmission or protect against environmental factors. The trade-offs between cost, performance, and availability of these materials further complicate the design process.
Additionally, aging of materials can affect their reliability over time. Changes in optical properties due to exposure to heat, light, or chemicals can shift the performance of light chambers, thus necessitating ongoing evaluation and potentially costly replacements.
End
The conclusion serves as an essential segment of this article, encapsulating the core ideas presented throughout. Understanding light chambers is vital for various specialized fields. They bridge theoretical principles and practical applications, showcasing the intersection of science and technology.
Summary of Findings
Over the course of this article, we have examined numerous aspects of light chambers. Key findings include:
- Scientific Basis: The dual nature of light and its interactions with matter establish the foundation for building effective light chambers.
- Design Innovations: Insights into materials and engineering considerations are crucial for creating efficient designs that optimize light management.
- Applications Across Disciplines: Environmental monitoring, biomedical research, and materials science all benefit from the unique capabilities of light chambers, proving their versatile utility.
- Technological Trends: Recent advancements anticipate a future where light chambers become integral components in emerging technologies like smart sensors.
These elements illustrate the significance of light chambers and their impact on modern science and engineering.
Final Thoughts
By understanding the mechanics and innovative uses of light chambers, students, researchers, and professionals alike can appreciate their importance. Continuous development in this field holds promise, as it offers new methodologies for research and practical solutions to complex problems.
"The study of light chambers provides a lens through which we can view the evolution of technology and its application across various domains."
Indeed, light chambers are not merely instruments; they are pivotal in shaping future scientific capabilities, reflecting how intertwined our understanding of light is with technological progress.
Cited Works
Cited works are the backbone of any academic discussion, particularly in a field as intricate as the study of light chambers. Here are the key elements to consider when dealing with references:
- Credibility: Utilizing peer-reviewed journals, reputable books, and validated online resources enhances the reliability of the information. For example, works published in journals like Nature or Physical Review Letters provide robust evidence for claims about light properties or photonic interactions.
- Diversity: Incorporating a range of sources, from historical texts to the latest research, creates a rich tapestry of knowledge. This enables a comprehensive understanding of both foundational theories and cutting-edge technologies.
- Contextualization: References not only substantiate claims but also provide a context for the topic. When discussing innovative technologies in light chambers, referencing recent advancements reported in specialized conferences or workshops helps illustrate current trends and future directions.
- Navigation: Well-structured citations allow readers to delve deeper into subjects of interest. For instance, links to original journal articles can direct researchers to detailed methodologies and results, enriching their comprehension and encouraging further exploration of specific areas.
Moreover, the selection of referenced works can reflect the interdisciplinary nature of light chambers, drawing from fields such as physics, engineering, and environmental science. Ultimately, carefully curated references enhance the article's value, appealing to students, researchers, educators, and professionals alike. They serve not only as support for the narrative but as invitations to expand one's knowledge base.