Exploring iPS Cell Culture Techniques and Their Impact
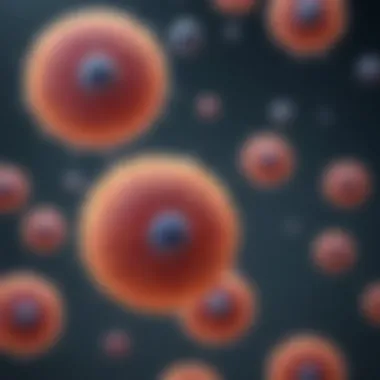
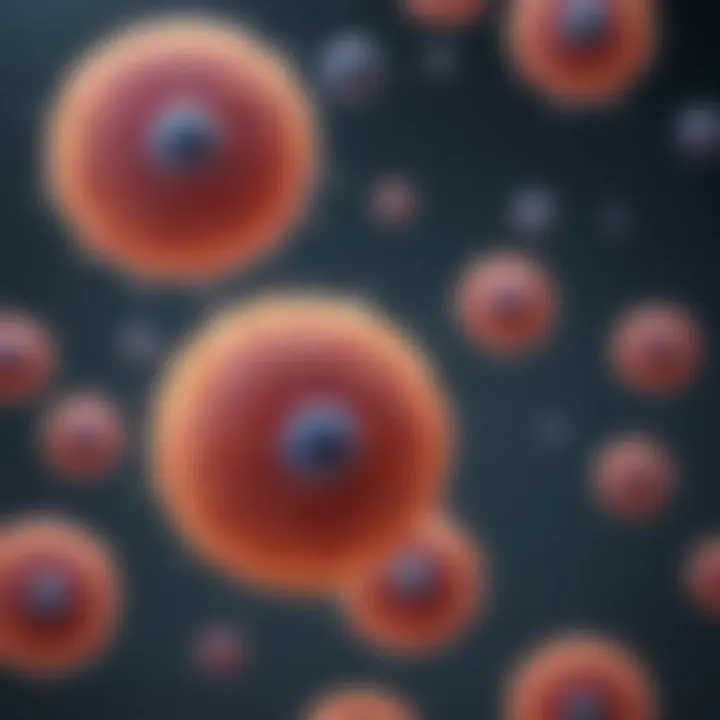
Intro
Induced Pluripotent Stem (iPS) cells are like a phoenix rising from the ashes of traditional stem cell research. They breathe new life into the field of regenerative medicine by allowing scientists to reprogram ordinary somatic cells into pluripotent cells, which closely resemble embryonic stem cells. This groundbreaking capability has opened the floodgates for potential therapies for a plethora of diseases. Yet, it doesn't come without its own set of challenges and ethical dilemmas.
As we plunge into this narrative, we’ll explore the multifaceted layers of iPS cell culture—from the methods employed in deriving these cells to the unique characteristics they possess. Understanding the fundamentals of their application in medicine and their ethical implications is essential, especially for students and professionals delving into the realm of cellular biology.
In the subsequent sections, we will methodically unpack vital findings and methodologies that highlight the advances researchers have made in this exciting field. We will also dive deeper into in-depth analyses that contrast current knowledge with historical perspectives, ensuring a well-rounded understanding of this innovative area of study.
Prelims to iPS Cell Culture
The rapid advancement of biomedical science has positioned Induced Pluripotent Stem (iPS) cell culture as a cornerstone of modern research and medical applications. This section serves as a gateway into understanding iPS cells, their origins, and their transformative capabilities. The relevance of iPS cell culture is evident in its potential to revolutionize regenerative medicine, offering novel solutions for tissue repair and disease modeling. Moreover, these cells allow for personalized approaches to treatment, making them essential in the quest for tailored therapeutics.
Defining Induced Pluripotent Stem Cells
Induced pluripotent stem cells are a unique class of cells that have been engineered from somatic cells. Through a process called reprogramming, these cells regain the ability to differentiate into any cell type in the human body. This characteristic of pluripotency means they can become neurons, cardiac cells, or even insulin-producing cells in the pancreas. This flexibility is akin to having a blank canvas that can transform into various forms, depending on how it's sculpted.
To put it into perspective, consider fashion: you have a basic fabric (the somatic cell) that, when taken to a skilled tailor (the reprogramming process), can be fashioned into countless garments (the diverse cell types). This remarkable ability is pivotal for research, enabling scientists to create precise cellular models that resemble human disease, or to generate cells for potential therapeutic uses.
Historical Context and Development
The journey of iPS cell culture dates back to a groundbreaking discovery made in the early 21st century. In 2006, Shinya Yamanaka, a prominent stem cell researcher, brought the concept of reversing somatic cell differentiation to the forefront. He identified a combination of four transcription factors—Oct4, Sox2, Klf4, and c-Myc—that could induce this reprogramming.
This pioneering work threw open the doors to endless possibilities. Before iPS cells came into play, the field heavily relied on embryonic stem cells, which drew ethical concerns due to their origin. iPS cells provide a solution by sidestepping these issues.
In a sense, iPS cells can be viewed as a bridge between the technological advances in genetics and the pressing needs in regenerative medicine. By 2009, Yamanaka’s breakthrough earned him a Nobel Prize, signaling the importance of this technology in the future of medical science. The evolution of this field continues at a rapid pace, pushing boundaries and sparking new inquiries into the nature of human cells and their potential.
Mechanisms of Reprogramming
Understanding the mechanisms of reprogramming is essential for grasping how induced pluripotent stem (iPS) cells are created and utilized. This process is not just about switching cells back to a more primitive state; it combines intricate biological pathways and factors that transmit information across various cellular systems. Essentially, the goal is to induce a state of pluripotency in somatic cells, allowing them to develop into almost any cell type. By understanding these mechanisms, researchers can optimize the protocols for iPS cell derivation, leading to enhanced potential applications in regenerative medicine, disease modeling, and drug testing.
Core Factors in Reprogramming
The reprogramming of somatic cells into iPS cells heavily relies on a handful of core transcription factors, commonly referred to as the Yamanaka factors, which include Oct4, Sox2, Klf4, and c-Myc. These chosen factors perform synergistic roles, effectively orchestrating the transcriptional activity necessary for cellular reprogramming.
- Oct4 plays a protective role in maintaining the pluripotent state and preventing differentiation.
- Sox2 is crucial because it collaborates with Oct4 to regulate genes that maintain pluripotency.
- Klf4 enhances the ability of Oct4 and Sox2 to activate pluripotency genes while inhibiting differentiation pathways.
- c-Myc boosts cellular proliferation and enhances reprogramming efficiency but carries risks such as tumorigenicity, which researchers must mitigate.
Methods of Induction
Inducing iPS cells through various methods carries its own advantages and challenges. The selected method plays a pivotal role in determining not just the efficiency of reprogramming but also the characteristics of the resultant cells. There are several strategies to induce this fascinating transition, which can be categorized into three major approaches.
Viral Vector Methods
Viral vectors, specifically lentiviruses and retroviruses, are among the most traditional methods used to introduce the core transcription factors into somatic cells. This approach is popular primarily because of its efficiency in delivering genes directly into the host cell's genome, leading to a high rate of reprogramming.
One of the key characteristics of viral vector methods is their ability to integrate into the host genome, thereby ensuring stable and long-term expression of the reprogramming factors. This feature significantly increases the reprogramming efficiency. However, there are also notable disadvantages. The integration of viral DNA can lead to genomic instability, raising concerns about potential oncogenic consequences. Thus, while highly effective, caution is necessary when utilizing this method.
Non-viral Methods
Non-viral methods have gained traction due to their safety profiles and fewer associated risks. Techniques such as electroporation, microinjection, and the use of episomal vectors fall under this category. Non-viral methods are appealing because they avoid the integration of foreign DNA into the host genome, thus decreasing the likelihood of mutagenesis.
The standout characteristic of non-viral approaches is their versatility. They can be modified and tailored to meet specific experimental needs. However, one of their weaknesses is often lower reprogramming efficiency compared to viral methods. This drawback leads to increased experimental time and resource investment.
Small Molecule Approaches
Small molecule approaches represent an innovative perspective on reprogramming. Instead of using factors to induce pluripotency directly, this method leverages small compounds to modulate signaling pathways and epigenetic modifications influencing somatic cell fate.
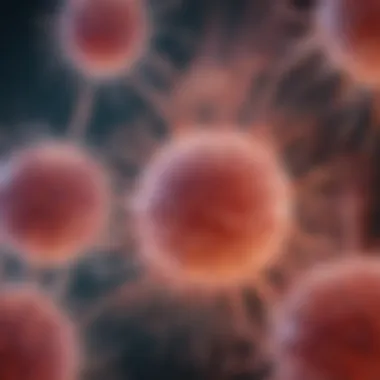
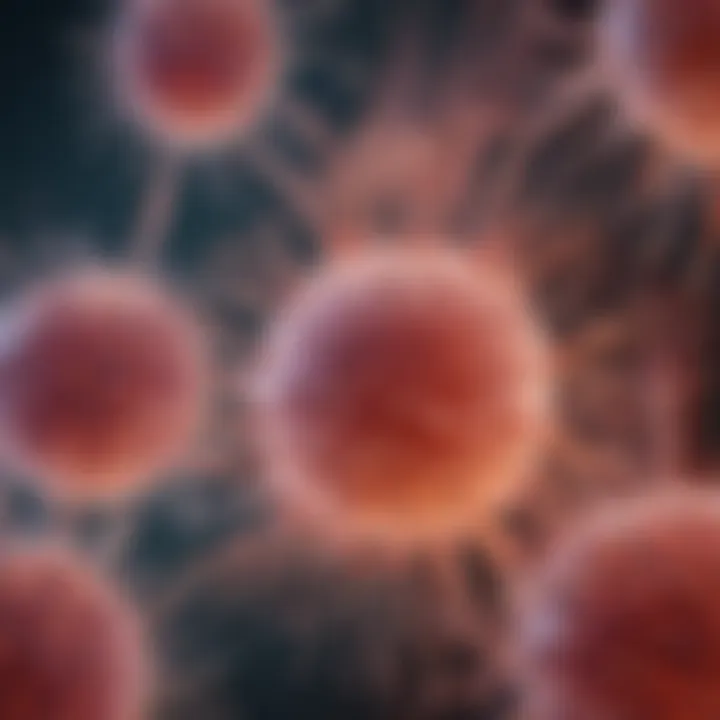
One of the key advantages of this approach is the reduced risk of genomic alterations since no foreign DNA is introduced. Furthermore, small molecules can be fine-tuned and used in combinations to enhance efficiency and cell quality. Yet, the research around small molecules is still in developmental stages; thus, their generalizability and effectiveness across various cell types remain under investigation.
"Understanding the different methods of iPS cell induction enables researchers to optimize their reprogramming protocols to better suit their specific goals, whether it's regenerative therapy or drug development."
Characteristics of iPS Cells
Understanding the characteristics of induced pluripotent stem (iPS) cells is pivotal in grasping their potential within scientific and medical advancements. iPS cells possess unique traits that differentiate them from other cell types, making them invaluable in various research applications. This section will elaborate not just on the scientific properties of iPS cells but also on their implications for rejuvenating medicine, disease modeling, and innovative therapies.
Pluripotency and Self-Renewal
One of the hallmark features of iPS cells is their pluripotency, which refers to their ability to differentiate into any cell type in the human body. This capability allows them to regenerate and repair damaged tissues, which holds immense promise for treating conditions like Parkinson's disease, heart disease, and diabetes. The ability to self-renew is equally remarkable; iPS cells can undergo unlimited mitotic divisions while maintaining an undifferentiated state, which is fundamental for long-term research and therapeutic applications.
Pluripotent stem cells can give rise to approximately 200 cell types found in a mature human body, marking a significant leap forward for regenerative therapies.
To put it simply, the pluripotency and self-renewal capacities enable researchers to create a vast array of cell types from a single iPS cell line, facilitating studies on cellular behavior, development, and disorders. These characteristics collectively allow scientists to generate tissues for transplantation, develop disease models that mimic human pathologies, and trial drug efficacy with high precision.
Gene Expression Profiles
The gene expression profiles of iPS cells reveal insightful information about their functionality. These profiles are typically a reflection of the cells’ pluripotent nature and their potential to differentiate into various cell lineages. Special transcription factors like Oct4, Sox2, and Nanog play crucial roles in maintaining pluripotency. The presence or absence of these proteins can determine the fate of the stem cells.
It's fascinating to observe that while iPS cells share similarities with embryonic stem cells, their gene expression profiles can vary slightly. The molecular signature of iPS cells often points to their derivation process from somatic cells, making them distinguished but fundamentally similar to embryonic stem cells.
In research, understanding these profiles is vital for optimizing culture conditions and differentiation protocols, ensuring that iPS cells are efficiently guided towards specific cell types, such as neurons or cardiomyocytes. Researchers justify that a deeper understanding of gene expression can enhance their manipulation, improving the utility across various applications.
Comparative Analysis with Embryonic Stem Cells
When comparing iPS cells to embryonic stem cells (ESCs), both share fundamental properties but exhibit notable differences. iPS cells resemble ESCs in their pluripotency, yet they are derived through reprogramming adult cells, which sidesteps some ethical concerns inherent in using embryonic cells.
Here are key points of comparison:
- Source of Cells: iPS cells are derived from adult somatic cells, while ESCs are obtained from pre-implantation embryos.
- Ethics: iPS cells circumvent the ethical dilemmas linked with embryo destruction, making them more favorable in the public and scientific spheres.
- Genomic Stability: Some studies suggest that iPS cells may exhibit genomic abnormalities due to the reprogramming process, but ongoing research aims to address these concerns to ensure their safety for therapeutic applications.
- Functional Efficiency: There can be variances in differentiation capabilities; for example, certain lineage-specific markers may express differently in iPS cells compared to ESCs.
In summary, while iPS cells emerge as a revolutionary alternative to embryonic stem cells, their full potential is still unfolding in research labs and clinics around the globe. The ability to produce patient-specific cell lines opens new avenues in personalized medicine, which is becoming a cornerstone of contemporary healthcare.
Understanding the characteristics of iPS cells provides a foundational insight into their applications and challenges within the evolving field of regenerative medicine.
Culturing iPS Cells
Culturing induced pluripotent stem (iPS) cells serves as a cornerstone in the field of regenerative medicine. A well-cultured population of iPS cells can differentiate into virtually any cell type, making them invaluable for both research and therapeutic applications. The dependence on well-established culturing techniques cannot be understated; they not only affect the viability of the cells but also their pluripotency and subsequent differentiation potential.
The importance of proper culturing lies in various factors, from the selection of the culture medium to the choice between feeder-free or feeder-dependent systems. Each aspect plays a crucial role in maintaining the characteristics of iPS cells, and any misstep may lead to compromised cell functionality. More importantly, it lays the groundwork for applications in disease modeling, drug discovery, and potential therapeutic interventions.
Culture Medium and Conditions
The selection of the right culture medium is pivotal in iPS cell maintenance. These cells thrive in a unique environment rich in nutrients, growth factors, and cytokines that create optimal conditions for self-renewal and pluripotency. A common medium used is KnockOut DMEM, which is supplemented with essential growth factors like bFGF (basic Fibroblast Growth Factor) and EGF (Epidermal Growth Factor). It’s crucial to avoid any contamination during the preparation and handling of the medium, as even a small mishap could lead to cell death or differentiation.
Additionally, environmental conditions such as pH, oxygen tension, and temperature must be rigorously controlled. iPS cells require an atmosphere low in oxygen (around 5% O2) for optimal growth. Small fluctuations in these conditions can significantly impact the cells.
"Culture conditions can make or break your iPS cell efforts. It's the difference between research gold and a failed experiment."
Feeder-free vs. Feeder-dependent Cultures
When it comes to culturing iPS cells, researchers face the choice of feeder-free or feeder-dependent systems. Feeder-dependent cultures involve coculturing iPS cells on a layer of fibroblast feeder cells, typically mouse embryonic fibroblasts. This system is favored for its ability to better maintain pluripotency; however, it brings along ethical and logistical complications—mainly due to the use of animal-derived components.
On the other hand, feeder-free cultures eliminate these ethical concerns and allow for a more controlled environment. They often utilize specialized matrices or coatings that support iPS cell growth. While feeder-free systems can simplify the scaling up of cultures and enhance reproducibility, they require a precise balance of growth factors and monitoring of cell confluence to avoid differentiation. Each system has its pros and cons, so the choice often hinges on specific research objectives or regulatory requirements.
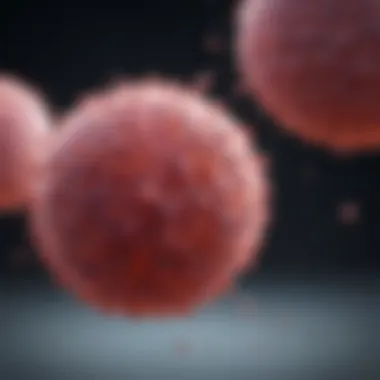
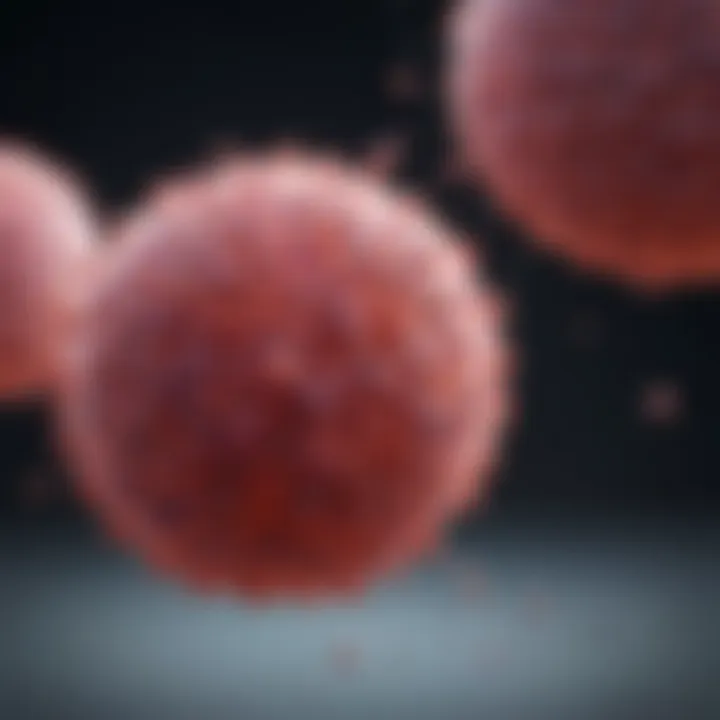
Cryopreservation Techniques
Cryopreservation techniques are vital for managing the long-term storage of iPS cells. Their ability to be frozen and thawed without losing their pluripotency is a boon to researchers, allowing them to maintain cell lines for future experiments. The most commonly used cryoprotectant is Dimethyl Sulfoxide (DMSO), which helps to prevent ice crystal formation that could damage the cells.
The process of freezing typically involves a gradual decrease in temperature, often achieved via a controlled-rate freezer. This method helps in preserving cell integrity. After storage, thawing the cells must be done promptly and careful to minimize cellular stress. The thawed cells usually need to be immediately transferred to appropriate culture conditions, as they are quite sensitive post-thaw.
In summary, successful culturing of iPS cells involves a multifaceted approach. From understanding the intricacies of culture media to adopting the right culturing systems and employing effective cryopreservation techniques, each step is vital for the fidelity and utility of these powerful cells.
Applications of iPS Cells
Induced Pluripotent Stem (iPS) cells have brought a seismic shift in our approach to biology and medicine. The potential applications of these cells are as diverse as they are groundbreaking, touching on multiple domains such as regenerative medicine, disease modeling, and drug development. Recognizing their significance is essential for grasping the broader picture of contemporary scientific progress.
Regenerative Medicine
In the realm of regenerative medicine, iPS cells stand out like a lighthouse in a storm. Their ability to differentiate into various cell types makes them a promising tool for treating degenerative diseases, injuries, and conditions resulting from cellular defects. For instance, a key point is their application in heart diseases. Researchers have been able to derive cardiomyocytes from iPS cells, which can help develop therapies to repair damaged heart tissues post-heart attacks. This is important not only for recovery but also for enhancing the quality of life for patients.
When we dive deeper, other applications include:
- Neurodegenerative diseases: By creating neurons from iPS cells, scientists are crafting models to study diseases like Alzheimer’s and Parkinson’s.
- Diabetes: iPS cells can differentiate into insulin-producing beta cells, offering hope for those suffering from type 1 diabetes.
- Spinal cord injuries: The use of iPS-derived neural stem cells in restoring connectivity after injuries shows promising results.
The journey from bench to bedside, however, invites caution. While the potential is vast, the translations from laboratory successes to clinical applications unveil challenges, including the risks of tumorogenesis and immune rejection that must be scrutinized rigorously.
Disease Modeling
Modeling diseases using iPS cells has ushered in a new era of understanding. Scientists can now recreate the disease’s environment in vitro, which makes it easier to study and unravel complexities that were once elusive. This approach also empowers researchers to observe how diseases progress on a cellular level, providing insights that could lead to innovative treatments.
Take cystic fibrosis, for example. By utilizing skin cells from patients, scientists can convert them into iPS cells, and subsequently into lung cells. This allows for an authentic glimpse at the pathophysiology of the disease, enabling the testing of new therapies in a patient-specific manner.
A few advantages of iPS cell-based disease modeling include:
- Patient-specific models: Allows for personalized medicine.
- Reduced animal testing: Ethical implications are greatly diminished.
- High-throughput screening: It becomes feasible to test many compounds rapidly.
Drug Development and Testing
The landscape of drug development is another area where iPS cells manifest remarkable potential. They provide a platform for high-throughput drug screening, allowing pharmaceutical companies to test a myriad of compounds on human cells, thus streamlining the search for effective treatments. Furthermore, testing drugs on iPS cells derived from specific patients can illuminate variations in drug responses due to genetic backgrounds, assisting in the move towards personalized medicine.
Some merits of utilizing iPS cells in drug development include:
- Toxicity testing: As derived from human cells, iPS cells can highlight adverse effects earlier in the development process.
- Predictive modeling: Helps in predicting how a drug will behave in the human body much more accurately than traditional models.
- Accelerated timelines: Reducing the time from discovery to market approval by refining the screening processes.
"Harnessing the power of iPS cells for drug testing could redefine our understanding of pharmacology and personalize therapeutic approaches."
Challenges in iPS Cell Research
The journey of iPS (Induced Pluripotent Stem) cell research is riddled with both immense promise and significant hurdles. While the potential applications of iPS cells in regenerative medicine and disease modeling are noteworthy, they do not come without challenges that need to be addressed. Understanding these issues is not merely academic; it shapes the trajectory of research endeavors and their future applications.
Among the most pressing considerations include genomic stability, ethical dilemmas, and the evolving regulatory frameworks that govern the use of these cells. Each of these aspects not only influences the research objectives but also affects public trust and acceptance of stem cell technologies. The impact of overcoming these challenges is far-reaching as it has the potential to enhance the efficacy and safety of therapeutic approaches using iPS cells.
Genomic Stability and Variability
When it comes to genomic stability, the nature of iPS cells is complex. While they are touted for their versatility, the reprogramming process can lead to various genomic alterations. Studies have shown that some iPS cell lines can harbor mutations that pose risks when they are utilized in therapeutic applications.
- Understanding the Variability: Such variability can surface from differences in the methods of cell reprogramming, the origins of somatic cells used, and the culture conditions employed. Notably, researchers have found that certain reprogramming methods may introduce different kinds of genomic changes, thereby affecting cellular function and behavior.
- Implications for Future Research: One of the major concerns is the potential compromise of genomic integrity, which can result in undesirable phenotypic changes. Thus, the importance of rigorous genetic screening in the development of iPS cells cannot be overstated. Ensuring genomic stability is vital for utilizing iPS cells in clinical applications, as compromised genetic characteristics can lead to tumorigenicity.
"Maintaining genomic integrity is not just a technical requirement; it's a matter of ensuring safety for future therapeutic applications."
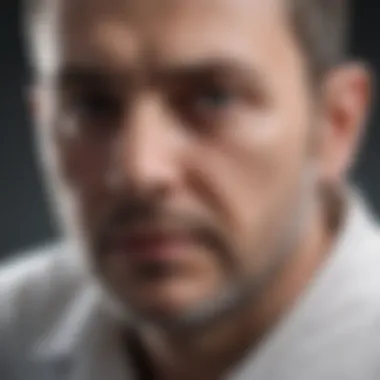
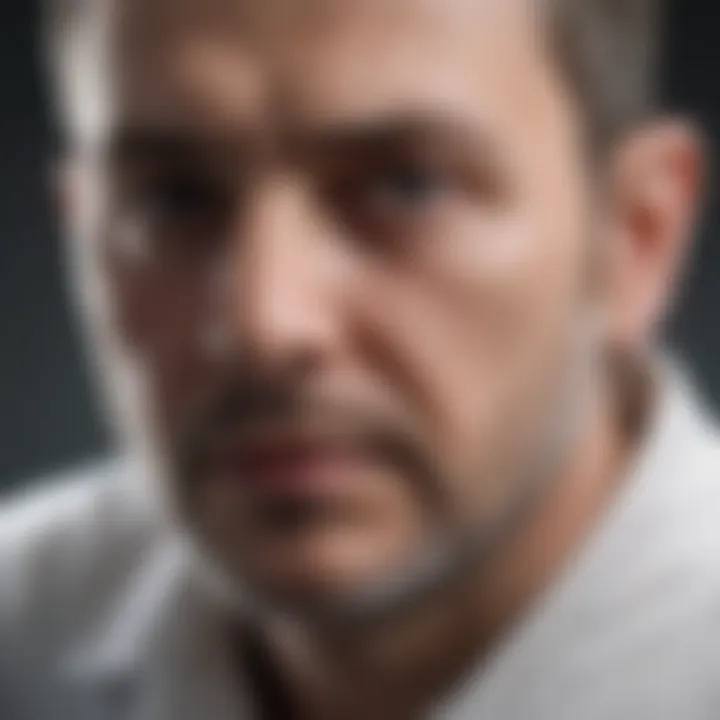
Ethical Considerations
Navigating the ethical landscape surrounding iPS cell research is another major hurdle. The excitement surrounding iPS technology often clashes with ethical concerns, particularly related to donor consent, accessibility, and the potential for misuse of stem cell technologies.
- Informed Consent: Ensuring that donors fully understand the implications of their contributions to iPS technology is crucial. This is especially relevant in contexts where genetic materials may be used for research without the donor’s ongoing consent.
- Accessibility and Equity: The distribution of advances in iPS cell technology raises ethical questions about equality in healthcare. Will these advanced treatments be available to only a select few or will they be accessible and inclusive?
- Potential Misuse of Technology: Another ethical consideration arises from the prospect of germline editing using iPS cells. This potential for editing could lead to ethical dilemmas that society is not yet prepared to handle.
Regulatory Landscapes
As iPS cells have found their place within the scope of biomedical sciences, regulatory frameworks are continuously evolving to keep pace with advancements. Navigating the legalities surrounding iPS cell research is critical for researchers and institutions alike.
- Changing Regulations: Each country has its own regulatory standards and guidelines that govern the use of stem cells. For instance, regulations in the U.S. might differ significantly from those in Europe and Asia, creating a complex landscape for global research collaboration.
- Approval Processes: Navigating through the approval processes for clinical trials that utilize iPS cells can be cumbersome. The need for robust oversight means that researchers must be thoroughly prepared to demonstrate both safety and efficacy before their studies can move forward.
- Future Directions: As research progresses, the need for varying regulatory approaches that balance innovation with public safety is becoming increasingly clear. Stakeholders, including policymakers, scientists, and ethicists, must engage in dialogue to establish guidelines that promote responsible research while fostering scientific progress.
Future Prospects of iPS Cell Technology
Induced Pluripotent Stem (iPS) cell technology stands at an exciting crossroads in its development. The potential for transformation in regenerative medicine and therapeutics is monumental. As researchers peel back more layers of this complex field, the future of iPS cells looks promising and multi-faceted. This section delves into three key areas: advances in cell engineering, the integration with gene editing technologies, and the nuanced landscape of clinical trials and regulatory pathways. Each of these elements holds significance for the broader application of iPS technology in medical science.
Advances in Cell Engineering
Recent strides in cell engineering have drastically altered the landscape of iPS cell technology. Techniques in tissue engineering and cell manipulation are now more sophisticated, allowing scientists to create iPS cells that can resemble more closely various adult cell types. For instance, researchers have begun using biomechanical stimuli to enhance the differentiation process. This method takes advantage of the physical cues in the cellular environment to direct stem cell fate.
Moreover, advancements in bioreactor technology enable large-scale production of iPS cells. Increased efficiency in cell culture means that therapeutic applications can become a reality. These enhancements in engineering mean that not only can we generate enough cells for clinical trials, but we can also fine-tune their properties for specific uses.
The integration of nanotechnology is yet another cutting-edge aspect of cell engineering, where nanomaterials are employed to influence cell behavior and enhance the efficiency of iPS cell applications. It’s an exhilarating area of research that combines biology with innovative materials science.
Integration with Gene Editing Technologies
The synergy between iPS cell technology and gene editing represents a paradigm shift in the way we can approach genetic diseases. Tools such as CRISPR/Cas9 have gained favor for their precision in editing genomes. Coupling this with iPS cells can lead to corrective treatments for genetic disorders that once seemed insurmountable.
By creating disease-specific iPS cells and then employing gene editing, researchers can study disease mechanisms in a controlled environment. This not only allows for a clearer understanding of the pathologies but also paves the way for possible interventions. The potential to correct mutations in real-time provides a hopeful prospect for tackling genetic diseases.
Further, gene editing can enhance how iPS cells are used for modeling disease, providing better approximations of human conditions. The precision of gene editing enhances the relevance of these models, making them more useful for drug testing and development.
Clinical Trials and Regulatory Pathways
Moving from bench to bedside involves an extensive process of clinical trials and regulatory scrutiny, which is vital for validating the safety and efficacy of new therapies based on iPS cells. The road can be fraught with challenges due to the intricate regulations governing stem cell research. Navigating these regulatory landscapes is paramount for researchers aiming to transition discoveries into therapeutic applications.
Currently, there are a number of clinical trials incorporating iPS cells in treatment protocols, particularly in regenerative medicine. Strategies range from treating degenerative diseases to addressing injuries that require cell replacements. However, developing new protocols requires stringent adherence to ethical considerations and regulatory frameworks, which differ widely across regions.
"Navigating the complex world of clinical trials with iPS cells requires not just scientific acumen but also a strong grasp of ethical implications and regulatory requirements."
The evolution of this regulatory landscape is crucial for the future of iPS cell applications. Collaborative efforts among researchers, ethicists, and regulators will be essential in streamlining pathways for trials without compromising on safety.
In summary, the future of iPS cell technology holds promises that are both tantalizing and substantial. With ongoing advancements in cell engineering, clever integrations with gene editing technologies, and a likely evolution in clinical trials and regulations, the landscape is set for remarkable breakthroughs that could redefine our approach to medicine.
Epilogue
The final section of this article serves to underscore the enduring significance of induced pluripotent stem (iPS) cell technology in the landscape of biomedical research and therapy. As we bring together the various threads of discussion, the importance of iPS cells cannot be overstated. These cells hold vast potential to not only reshape our understanding of cellular biology but to also transform clinical practices that address a myriad of diseases.
Summarizing the Impact of iPS Cells
The impact of iPS cells extends beyond the laboratory bench, influencing fields ranging from regenerative medicine to pharmacology. By utilizing iPS cells, researchers can produce patient-specific cell types, allowing for the development of personalized therapies. This personalization is crucial in treating diseases that have long been difficult to manage, such as neurodegenerative disorders and certain types of cancer.
The technology provides a unique opportunity to mimic human disease in vitro, enabling researchers to study the progression of disease at a cellular level. As a result, this can lead to more effective drug testing and reduced reliance on animal models, which often fail to predict human responses accurately. Additionally, advancements in iPS cell technology continue to inspire innovation, creating new pathways for scientific inquiry and collaboration.
"iPS cells are paving the road to a new era in medicine, where treatment can be tailored based on individual patient needs."
Call for Continued Research and Collaboration
Despite the tremendous advancements, we are on the cusp of a paradigm shift. Continued research in iPS cell technology is critical for overcoming existing challenges. Issues such as genomic stability, ethical considerations, and regulatory compliance must be addressed to further expand the applicability of iPS cells in clinical settings.
Collaboration across interdisciplinary teams—comprising biologists, ethicists, regulatory experts, and clinicians—will foster innovation and address these challenges comprehensively. Open sourcing research findings and sharing methodologies can streamline advancements, leading to collective progress.
By investing in these collaborations and a shared commitment to ethical research practices, we can harness the full potential of iPS cells for future generations. Together, the scientific community can not only enhance our current understanding but also translate these findings into real-world applications that benefit society as a whole.