Deep Dive into Confocal Microscopy Techniques

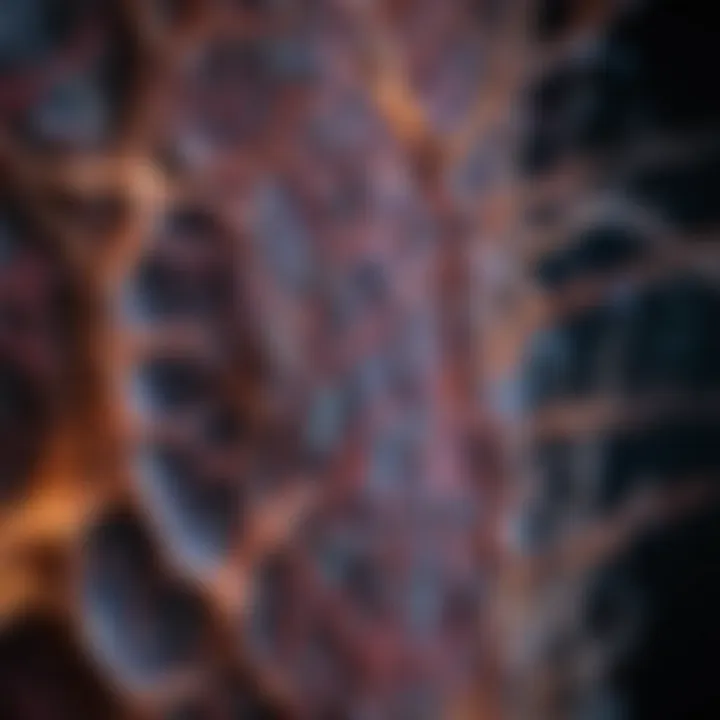
Intro
Confocal microscopy is more than just a fancy tool in the biological sciences; it’s a game-changer, really. With its ability to capture high-resolution images of cells, it allows scientists to peek into the intricate structures that make up life. This method uses a laser beam to focus on a single point in a sample, capturing sharp images layer by layer, which can be assembled into a 3D representation. This precision is what makes it essential for both research and diagnosis.
Research Overview
Summary of Key Findings
One of the notable discoveries in the realm of confocal microscopy is its significant role in cellular studies. Researchers have uncovered that this technique does not only improve resolution compared to traditional microscopy but also enhances the depth of field. This means that structures that were once blurred and indistinct are now clear and detailed. Furthermore, confocal microscopy has paved the way for multi-color imaging, enabling scientists to visualize different cellular components simultaneously without losing clarity.
Methodologies Employed
To achieve these remarkable results, a variety of methodologies have been applied. High-speed scanning, for instance, is essential in reducing photodamage to live samples. Advanced software tools aid in reconstructing images through algorithms that analyze the data captured. These innovations, coupled with improvements in fluorochrome development, mean that confocal microscopy can be utilized across various fields, from cancer research to neuroscience.
In this exploration, we will dive deeper into what makes confocal microscopy tick, understand its advantages and limitations, and identify its diverse applications in contemporary scientific research.
In-Depth Analysis
Detailed Examination of Results
An evaluation of recent studies highlights how confocal microscopy has transformed our approach to cellular biology. For example, a 2022 study utilized this imaging technique to observe synaptic changes in real time, revealing crucial insights into neurodegenerative diseases. The clarity afforded by confocal setup allowed for the detection of minimal changes in neuronal structures that were previously imperceptible.
Comparison with Previous Studies
Looking back, earlier forms of microscopy such as light and electron microscopy lacked the resolution and convenience that confocal microscopy provides. Traditional microscopes would often blur details due to light scattering, whereas electron microscopy, although precise, comes with its own set of limitations, including sample preparation challenges. This side-by-side comparison not only underscores the superiority of confocal systems in various scenarios but also highlights the continuous advancement made by researchers seeking to refine this method.
"Confocal microscopy stands at the forefront of scientific inquiry, merging technology with biology to unlock secrets hidden within cells.”
Prologue to Confocal Microscopy
Confocal microscopy represents a critical advancement in imaging technology, shaping the landscape of cellular biology and medical research. By forming sharp, high-resolution images of specimens, this technique enables scientists to observe intricate cellular structures and functions in ways that traditional light microscopes simply cannot. This powerful tool has become indispensable not just in basic research but also in clinical diagnostics and innovative therapeutic solutions.
Historical Background
The origins of confocal microscopy date back to the 1950s, when a scientist named Marvin Minsky proposed a method that allowed for clearer images of specimens by eliminating out-of-focus light. Minsky's vision was revolutionary. Fast forward to the 1980s, and the first commercial confocal microscope was introduced, making it accessible to researchers and opening new avenues for imaging techniques. This period marked a sharp increase in interest and development in microscopy, with enhanced capabilities such as laser scanning and improved optical components.
With the advent of computers in the late 20th century, confocal microscopy underwent transformative developments. The ability to digitize images and process them using sophisticated algorithms allowed researchers to create detailed three-dimensional reconstructions. This transformed the way scientists approached their investigations, providing them with tools that could unveil secrets hidden deep within cellular structures.
Basic Principles
At its core, confocal microscopy employs a laser light source to illuminate a specimen, which may contain fluorescently labeled components. The key feature that sets it apart from traditional light microscopy is the use of a pinhole aperture. This pinhole filters out the light that does not originate from the focal plane, resulting in images that boast significantly enhanced contrast and resolution.
The basic process of confocal microscopy involves several critical steps:
- Laser Excitation: A laser beam is directed onto the specimen. This laser excites fluorescent dyes within the sample, causing them to emit light.
- Image Localization: The emitted light is collected through the same objective lens that illuminated the sample. As the laser scans across the specimen, it captures light from a single plane at a time, aiding in the production of sharper images.
- Pinhole Limitation: The optical pinhole ensures that only light from the focal plane passes through to the detector. This further clarifies the image, significantly reducing background noise.
- Detector Capture and Image Assembly: Detectors convert the emitted light into an electronic signal, which is then processed and assembled into a detailed two-dimensional image.
In essence, the combination of laser scanning and pinhole technology creates a three-dimensional representation of the sample, allowing researchers to view intricate cellular details. The elegance of confocal microscopy lies in its ability to reveal information that is not just an approximation of reality but rather a nuanced portrayal of biological phenomena.
Confocal microscopy is not merely a tool; it's a means to visualize the unseen intricacies of life itself.
In summary, understanding the historical context and fundamental principles of confocal microscopy allows one to appreciate not just its utility but also its profound impact on scientific inquiry. As we continue through this article, readers will gain further insight into the components that make up this sophisticated instrument, its advantages, challenges, and the depths it allows us to explore within biological sciences.
Key Components of Confocal Microscopes
The realm of confocal microscopy hinges on several core components that must seamlessly work together. Each part—ranging from the laser light source to the optical pinhole—plays a distinct role in achieving high-resolution, in-focus images of cellular structures. Understanding these components not only clarifies the workings of confocal microscopy but also highlights their significance in various research applications.
Laser Light Source
At the heart of confocal microscopy is the laser light source. Unlike traditional bright-field microscopy that uses incoherent light, confocal microscopy harnesses the power of lasers to produce coherent light. This coherence ensures that the beam remains focused, allowing it to penetrate deeply and illuminate the sample uniformly. Moreover, lasers can produce specific wavelengths which makes them invaluable for exciting fluorescent dyes, commonly used in biological samples.
A wider range of laser wavelengths can shine a spotlight on various structures, enabling researchers to visualize everything from proteins to entire cell organelles. For instance, using a green fluorescent protein might only require a specific laser that emits in the green spectrum. This specificity can lead to far cleaner images, thus enhancing the clarity and depth information captured in 3D reconstructions.
Objective Lenses
The objective lenses form the next critical link in the confocal microscopy chain. These lenses are key modifiers of light, focusing it onto the sample and then onto the detector. They are often categorized by their numerical aperture (NA) – a measure of the lens's ability to gather light and resolve fine specimen detail at a fixed object distance. Higher NA values translate to improved resolution, enabling scientists to resolve features as small as a few hundred nanometers.
Using an objective lens with a high NA is especially essential when observing biological samples that are often delicate and inherently complex. For instance, a lens with a NA of 1.4 can double the resolution compared to one with a NA of 0.7. Therefore, selecting the right lens per the imaging goal is not merely a choice; it is crucial for obtaining optimal results.
Detectors
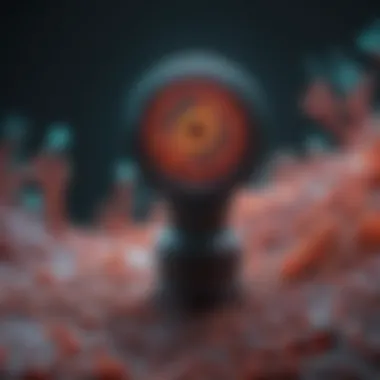
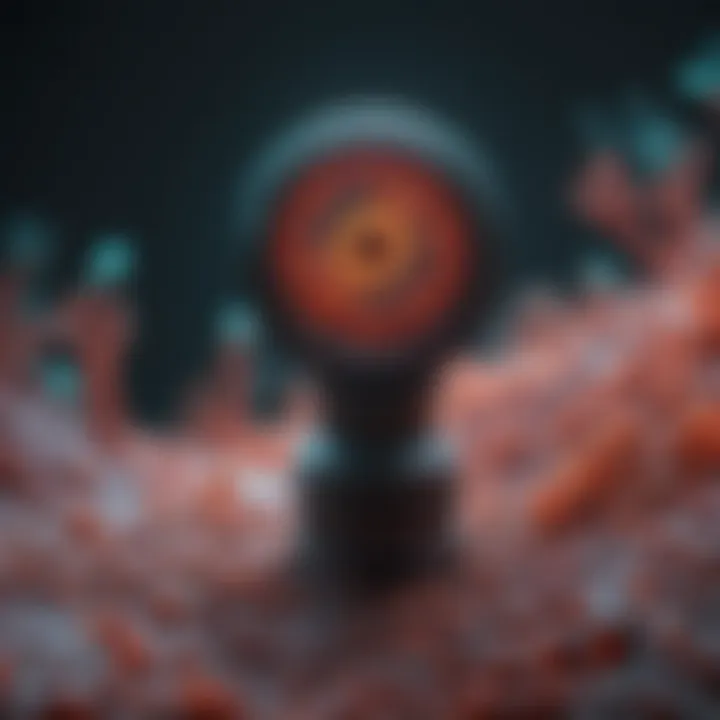
Once the light has been gathered and sculpted by the objective lens, it makes its way to the detectors. These are typically photomultiplier tubes (PMTs) or more recently, dark field cameras, both known for their sensitivity to light. PMTs convert light into an electrical signal, allowing for the quantification of fluorescence emitted by the sample. The efficacy of the detector can significantly impact image quality. Higher sensitivity detectors can pick up the faintest signals, which is critical when studying samples with low fluorescent expression.
In a nutshell, detectors serve as the ultimate sensory organs of the confocal microscope, translating biochemical happenings into concrete visual data that scientists can interpret. This capability enables them to navigate through various questions in toxicology, biomedical research, and more.
Optical Pinhole
Another defining component is the optical pinhole. Positioned in the light path, this pinhole eliminates out-of-focus light that could otherwise blur the image. By allowing only in-focus light to reach the detector, it plays a pivotal role in increasing image contrast and clarity. The size of the pinhole can be adjusted based on the specific imaging needs, such as if the sample has strong fluorescence or if a more defined depth of field is required.
When adjusted correctly, the optical pinhole not only enhances resolution but also permits optical sectioning. This process allows researchers to capture a slice of a thick specimen without interference from other layers, revealing the meticulous architecture of cells and tissues.
In summary, the integrated functioning of these components—the laser light source, objective lenses, detectors, and optical pinhole—is what makes confocal microscopy a powerful tool in biological research. Each element complements the others, crafting an experience that allows for the detailed investigation of cellular processes and structures.
The Mechanism of Image Acquisition
Understanding the mechanism of image acquisition is fundamental to grasp the overall capabilities and functionality of confocal microscopy. In essence, this process allows scientists to capture high-resolution images with remarkable clarity, giving researchers insights into cellular structures that were previously elusive. Within this realm, two critical elements emerge: scanning techniques and image reconstruction. They work hand-in-glove, each contributing unique advantages that enhance microscopy outcomes while addressing inherent challenges.
Scanning Techniques
Scanning techniques utilized in confocal microscopy play a vital role in how images are formed. These techniques determine how light interacts with the sample, ultimately leading to the creation of the detailed imagery researchers rely on. One prominent approach is the point scanning method, where a focused laser beam moves across the specimen in a raster pattern. It systematically illuminates the sample, with each point contributing to the final image, akin to painting a picture one stroke at a time.
However, there’s more in the toolbox than just point scanning.
- Line Scanning: Light is swept across the specimen in parallel lines, leading to faster acquisition times. This is especially useful for dynamic samples that change over time.
- Area Scanning: In this method, the entire field of view is illuminated simultaneously, which can be beneficial for large samples, though it often sacrifices some resolution.
The choice of scanning technique often correlates with the desired outcomes of the experiment. Fast-moving samples may require quicker scanning methods, while dense structures might benefit from slower techniques that focus on detail. Regardless, the end goal remains consistent: obtaining high-quality images that reveal intricate biological features.
Image Reconstruction
Once the scanning is done, the next phase is image reconstruction, a crucial step in confocal microscopy that transforms collected data into coherent images. This process involves the compilation of signals received from various points in the specimen, allowing segmentation of different layers, much like assembling a puzzle from scattered pieces.
Several technologies are at play during image reconstruction.
- Signal Processing Algorithms: These algorithms refine the data by increasing contrast and reducing noise. Techniques such as deconvolution can particularly enhance resolution, enabling visualization of finer details.
- 3D Reconstruction: Given that confocal microscopy captures images at various depths, reconstructing these into three-dimensional representations can provide profound insights into spatial relationships within the sample.
- Software Integration: Advanced imaging software aids researchers in monitoring and manipulating captured images for detailed analysis.
The success of image reconstruction largely hinges on how data is managed. The combination of effective scanning and robust reconstruction algorithms ensures images are not just clear, but also informative.
"High-quality imaging results from the perfect marriage of scanning techniques and reconstruction processes. It's a dynamic interplay that elevates confocal microscopy to the forefront of biological investigation."
Advantages of Confocal Microscopy
Confocal microscopy presents a remarkable leap in optical imaging, providing advantages that spectrum across various scientific fields. As researchers continuously seek more precise tools for exploring cellular structures and their intricacies, confocal microscopy emerges as a critical ally. Its promise lies not only in enhanced imaging quality but also in its capacity to adapt to diverse experimental needs.
Enhanced Resolution
One of the standout features of confocal microscopy is its ability to produce images with significantly enhanced resolution. This is achieved through a combination of focused laser illumination and spatial filtering, which allows for the capture of sharp, clear images at cellular resolution. Unlike traditional microscopy methods, where out-of-focus light can muddle the final image, confocal microscopy mitigates this issue effectively.
By utilizing a pinhole aperture that allows only in-focus light to reach the detector, confocal microscopes eliminate much of the background noise that often interferes with image clarity. This optical sectioning technique results in images that reveal fine details that might otherwise go unnoticed—a real boon for studies requiring meticulous observation of subcellular structures. Consequently, researchers can discern even the most delicate cellular changes with reliability.
Optical Sectioning
The principle of optical sectioning further amplifies the appeal of confocal microscopy. In this context, the term implies the capacity to selectively capture images from distinct layers within a specimen rather than obtaining a flat, two-dimensional projection of the entire specimen simultaneously. This layered approach permits researchers to examine thick specimens with unprecedented accuracy and detail.
Through this mechanism, confocal microscopes allow for the reconstruction of three-dimensional images from a succession of two-dimensional slices. This capability plays a crucial role in various biological studies, enabling scientists to investigate complex structures like tissue architecture and the spatial interactions of cells within their environment. The result is a comprehensive depiction of cellular dynamics that is invaluable for understanding biological processes.
Real-time Imaging
Another compelling advantage is the potential for real-time imaging. Confocal microscopy can facilitate continuous observation of live specimens, granting researchers insights into dynamic biological processes as they occur. This aspect opens a window into temporal studies that unveil how cells interact, respond to stimuli, or undergo physiological changes over time.
The integration of fast scanning techniques and advanced detectors not only enhances the speed of image acquisition but also maintains high-resolution data. Such capacity is essential in the study of processes like cell migration, signaling pathways, or developmental biology—fields that thrive on real-time data to construct narratives of cellular behaviour.
Real-time imaging capabilities of confocal microscopy bring forth a new dimension of biological research, where observations can lead to immediate interpretations, thus accelerating discoveries.
In summary, the advantages of confocal microscopy are manifold, making it an indispensable tool for countless scientific investigations. With enhanced resolution, optical sectioning, and real-time imaging, it paves the way for a more profound understanding of the biological world, driving innovations and fostering advancements in various disciplines.
Limitations and Challenges
When engaging with confocal microscopy, it's essential to not only appreciate its many strengths but also to be mindful of the limitations and challenges that accompany this impressive technology. While it excels in providing detailed images, it doesn't come without its set of hurdles. Acknowledging these challenges becomes pivotal for researchers aiming to optimize their methodologies and realizing the full potential of their experiments. Let's delve into these issues more deeply.
Depth Penetration Issues
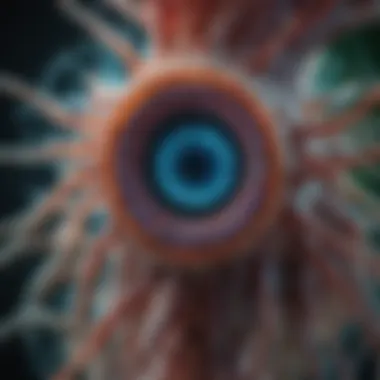
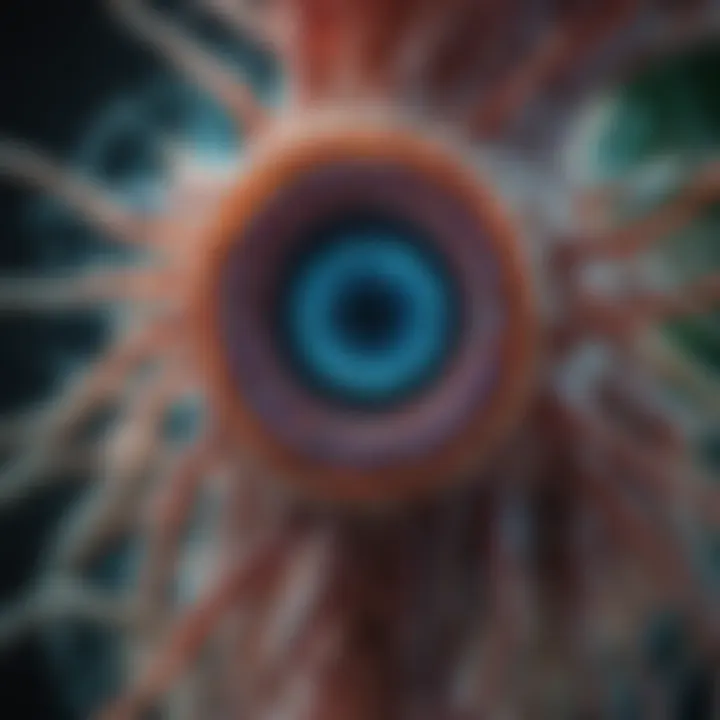
One significant limitation that often surfaces in confocal microscopy is the matter of depth penetration. The technique predominantly utilizes laser light, which, here lies the issue, doesn't always penetrate deeply into thicker samples. This creates a conundrum, especially when trying to visualize structures that reside several micrometers beneath the surface of the specimen. For instance, imaging within tissues can prove particularly problematic; the deeper you go, the less clear the images become due to scattering and absorption of light.
The implication of this restriction is particularly critical when the aim involves studying three-dimensional biological structures. Therefore, researchers frequently find themselves needing to thin samples to acquire clearer images or utilizing alternative techniques, potentially complicating the overall experimental design and thereby consuming precious time and resources.
Photobleaching Risks
Another concern that researchers must grapple with is the risk of photobleaching. This phenomenon occurs when fluorescent dyes lose their ability to fluoresce over time when exposed to the excitation light. Imagine being in the middle of a fascinating observation only to find your fluorescent marker starts dimming right before your eyes. Frustrating, right?
With prolonged exposure, not only can this lead to loss of critical data but can also complicate the interpretation of results. Strategies to minimize photobleaching involve:
- Reducing the duration of laser exposure.
- Using photostable fluorescent markers to maintain signal integrity.
- Applying light-sensitive imaging settings to preserve sample viability.
Nonetheless, while these strategies help mitigate photobleaching, they add another layer of complexity to quantitative studies which can turn data visualization into quite the balancing act.
Cost and Accessibility
Cost can be a significant barrier when it comes to confocal microscopy. High-quality confocal microscopes are expensive investments. They may include sophisticated components and advanced technology, making them unattainable for smaller organizations or labs with limited funding.
Besides the initial purchase, ongoing maintenance and operational costs can further exacerbate financial constraints. For example, the specialized training for personnel to operate these complex systems and interpret results accurately adds another layer to the cost.
In many cases, accessibility becomes a major point of contention; institutions with limited resources may miss out on employing confocal microscopy altogether, thereby hindering their research capabilities. Hence, recognizing these economic challenges is vital for stakeholders to develop sound strategies that could help bridge the accessibility gap in this realm of scientific exploration.
In summary, while confocal microscopy stands as a remarkable technique for imaging cellular structures, researchers must stay alert to its limitations concerning depth penetration, photobleaching, and overall cost. Understanding these challenges can encourage innovation in methodologies and preparation, ultimately enriching the realm of biological research.
Applications Across Biological Sciences
Confocal microscopy has transformed the landscape of biological sciences, allowing researchers to delve deeper into the intricacies of cellular structures and functions. This technique provides high-resolution images, enabling scientists to visualize details that were once obscured or unattainable. The significance of confocal microscopy lies in its versatility; it finds applications across various branches of biology, from studying individual cells to understanding complex neural networks. Here’s a closer look at three pivotal areas where confocal microscopy has made substantial contributions.
Cell Biology
In cell biology, imaging of the cellular architecture is critical. Confocal microscopy allows for the observation of cellular compartments with precision. For instance, researchers can study the dynamics of organelles like mitochondria and lysosomes in real time. This is particularly invaluable in observing how cells respond to stress or other external stimuli.
One specific example is in the examination of cancer cells. By using confocal microscopy, scientists can monitor morphological changes in cells subjected to various treatments. This helps in understanding the effectiveness of certain drugs and can even lead to the discovery of novel therapeutic strategies.
Moreover, confocal microscopy's ability to perform optical sectioning means that images can be captured at various depths within the sample. This makes it easier to construct 3D models of cells, offering unparalleled insights into cellular architecture. Such imaging capabilities enable better abilitiy to analyze processes like apoptosis or cellular migration.
Neuroscience
Neuroscience benefits immensely from confocal microscopy, especially in the study of brain structure and function. Imaging techniques like this enable scientists to visualize neuron connections—synapses—and observe embryonic neural development in animals.
A noteworthy application is in the exploration of neurotransmitter release. Confocal microscopy allows researchers to see real-time interactions between neurons, shedding light on how signals propagate throughout neural networks. For instance, tracking calcium ions in neuronal cells can reveal a lot about how synaptic connections form and strengthen.
Additionally, confocal microscopy can be applied to investigate neurodegenerative diseases, such as Alzheimer's. By imaging brain slices, scientists can visualize amyloid plaques or tau tangles, markers of such conditions. This type of imaging supports efforts to develop targeted interventions and enhances understanding of the pathophysiology of neurodegeneration.
Developmental Biology
In the field of developmental biology, confocal microscopy is pivotal in tracing the fate of cells during organismal development. The technique has been instrumental in studies that analyze how specific genes control the growth and differentiation of cells. This is crucial for understanding complex processes like embryogenesis or limb formation.
In practice, confocal microscopy is used to label particular cell types with fluorescent markers, allowing visualization during different stages of development. For example, investigations into zebrafish development use this microscopy to monitor how stem cells differentiate into various tissues and organs.
Additionally, confocal imaging can reveal cellular interactions and signaling pathways that are crucial during development. This information can lead to insights into congenital disorders and developmental plasticity, which observes how organisms adapt their growth in response to environmental changes.
"Confocal microscopy bridges the gap between visual artistry and scientific inquiry, allowing researchers to paint a detailed picture of life at the smallest scales."
Through its diverse applications across cell biology, neuroscience, and developmental biology, confocal microscopy stands out as a powerful tool in the biological sciences. Its ability to provide clear, detailed images enriches our understanding of life at the cellular level, advancing both research and education in the field.
Innovations in Confocal Microscopy
As the world of biological sciences evolves, the techniques used for microscopy must also adapt to meet the growing demands for precision, accuracy, and depth of information. The innovations in confocal microscopy are crucial as they enhance both the capability and the versatility of this already powerful imaging technique. Not only do these advancements allow for deeper exploration into cellular structures, but they also facilitate a higher level of detail that can lead to groundbreaking discoveries in various research fields.
Spectral Imaging
Spectral imaging is a cutting-edge innovation that allows for the simultaneous capturing of multiple wavelengths of light during imaging. By employing multiple detection channels, this method can separate various fluorescent signals emitted from biological samples. The beauty of spectral imaging lies in its ability to reduce overlapping signals, which can be a common headache when using traditional fluorescent markers. This capability enables researchers to better distinguish closely located cellular components, leading to more accurate analysis of complex samples.
In practical terms, spectral imaging can significantly boost the quality of results in live cell imaging, where changes occur rapidly and must be captured in real-time. The ability to collect and separate signals from different fluorophores means that scientists can track multiple targets in a single specimen without losing clarity. Methods for interpreting the spectral data have also matured, allowing a more nuanced analysis that can yield insights into the interactions between different cellular molecules.
Multi-Photon Excitation
Multi-photon excitation is another remarkable advancement that changes the game in confocal microscopy. This technique employs two or more photons of lower energy to excite fluorophores. The result? A deeper penetration into biological tissues without the side effects usually associated with higher-energy photons, like photodamage. By utilizing this method, researchers can capture images from much greater depths within thick samples—something traditional confocal methods struggle with.
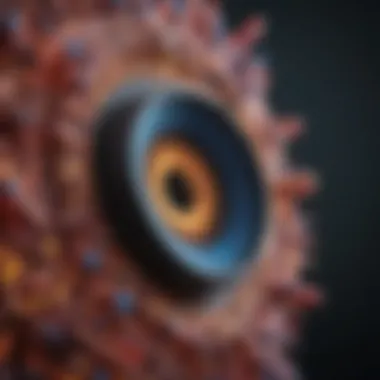
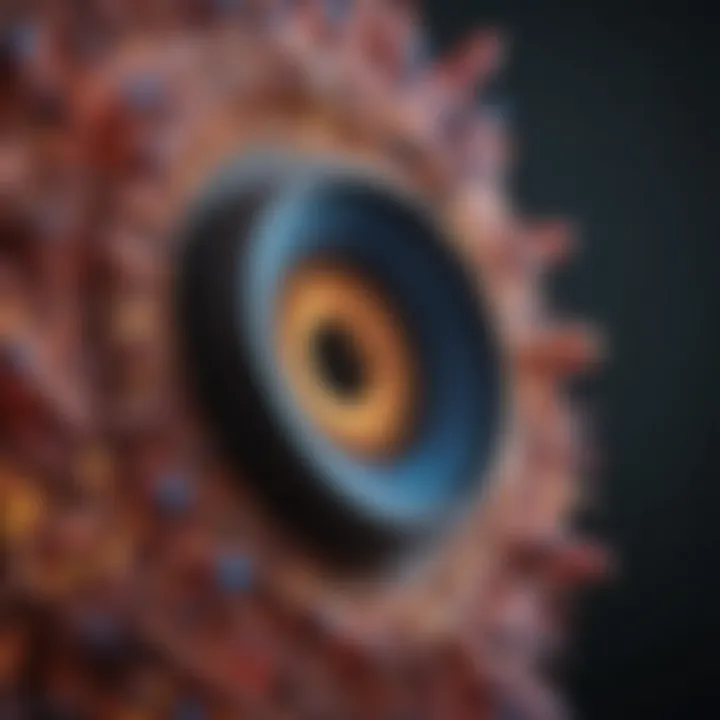
Notably, this approach is exceptionally beneficial in neuroscience and developmental biology, where samples can be quite thick and intricate. It opens doors to visualize neuron interactions or to map out developmental changes in embryos without the need to slice up the samples, preserving their natural state.
Moreover, multi-photon excitation reduces the overall photobleaching of the specimen since it uses lower energy, allowing longer imaging sessions. This is increasingly vital when studying dynamic processes over extended periods, ultimately adding value to in vivo research.
"Multi-photon microscopy redefines not just how we visualize, but also what we can discover within the realms of biological complexity."
As confocal microscopy continues to advance, mastering these innovations clusters the existing technologies together, redefining our capability in scientific research. With techniques like spectral imaging and multi-photon excitation, researchers gain access to a more multifaceted understanding of cellular mechanisms, pushing the limits of what we can see and learn in the intricate world of cells.
Future Directions in Confocal Microscopy Research
As the field of microscopy advances rapidly, confocal microscopy evolves too, openening up new avenues for exploration and application. Understanding the future of this technology is crucial for researchers and practitioners alike, as they seek to push the boundaries of what can be achieved in biological imaging. This section will delve into two significant pathways for the ongoing development of confocal microscopy: the integration with other techniques and improvements in hardware.
Integration with Other Techniques
The future is undoubtedly in collaboration between various imaging techniques. Integrating confocal microscopy with methods like super-resolution microscopy and electron microscopy is on the horizon. These combinations not only enable detailed imaging of cellular structures but also allow for a more comprehensive analysis.
For instance, super-resolution techniques can overcome some of the resolution limits imposed by traditional confocal setups. This fusion is critical when looking to quantify cellular dynamics in vivo, where understanding the fine details can influence biological conclusions.
Benefits of Integration:
- Enhanced Resolution: Combining the strengths of different techniques leads to clearer images and a more in-depth understanding of samples.
- Broader Applications: Capabilities in pathologies like cancer research and developmental biology can be expanded with data from synergistic imaging approaches.
- Real-time Analysis: Technologies such as fluorescence resonance energy transfer (FRET) benefit from confocal imaging when tracking cellular interactions swiftly.
Research groups around the globe are looking into these collaborative efforts, highlighting how blended techniques can unlock answers to complex biological questions. The potential for innovation lies within how these methodologies complement each other.
Improved Hardware Developments
Another promising direction for confocal microscopy is the ongoing improvements in hardware. With advancements in lasers, detectors, and even computational techniques, confocal microscopes are becoming more robust and versatile.
Specifically, the development of compact laser sources has transformed the magnitude of confocal imaging. These lasers are not only smaller and more energy efficient, but they also provide a wider range of wavelengths. This variety enhances the capabilities of multicolor imaging, allowing scientists to view multiple fluorescent markers simultaneously. The implications for research are profound, expanding what can be visualized in a single experiment.
Moreover, emerging technologies in detector sensitivity mean that researchers can capture low-abundance fluorescent signals that were once nearly impossible to resolve. Enhanced sensitivity leads to better detection limits, enabling scientists to study extremely rare cells or signals within complex samples, which could change the narrative in many fields.
"As we look ahead, the drive for better resolution, faster imaging, and broader application of confocal methods cannot be overstated."
Investments in computational power are also noteworthy, with robust algorithms reaching new heights in processing and analyzing imaging data. This not only facilitates quicker results but also enhances the reproducibility of findings, a critical factor in scientific research.
Ethical Considerations in Confocal Research
When engaging with advanced imaging technologies like confocal microscopy, it's crucial to navigate the murky waters of ethical considerations. The implications of using such powerful techniques extend beyond the sheer scientific insights they offer. They hold the responsibility of ensuring that research practices honor both the integrity of biological samples and the sanctity of scientific inquiry. By exploring these intricate layers of ethics, we also seek to enhance the reliability of research outcomes.
Sample Preparation
Sample preparation can sometimes be the difference between obtaining high-quality images and muddled results. Researchers often require specimens that have not only been adequately prepared but are also representative of a real biological context.
When working with live samples, researchers must consider the humane treatment of organisms. This includes minimizing suffering and distress. Additionally, one must adhere to protocols that require proper consent, especially when human tissues are involved. This may involve obtaining informed consent from donors and ensuring their anonymity is preserved. Here are several key aspects to keep in mind:
- Transparency in procedures: It’s essential that all sample preparation methods are clearly documented. Transparency helps in fostering trust within the scientific community.
- Standardization of protocols: Ensures repeatability and comparability of results across studies. Established guidelines should be followed rigorously to mitigate variables that might compromise results.
- Environmental considerations: The origin of samples—ensuring they come from sustainable sources—can often weigh on the ethical scale and should not be taken lightly.
"The ethical treatment of biological materials is as vital as the scientific outcomes they yield. Without proper care, the integrity of our findings is at stake."
Data Integrity
Data integrity is the bedrock of credible scientific research; it encapsulates the authenticity, consistency, and reliability of data generated through confocal microscopy. For researchers using this technology, upholding data integrity serves not only to strengthen their findings but also to maintain public trust in scientific processes. Some core elements contributing to this complex issue include:
- Accurate data representation: Researchers must present their results in a manner that does not mislead. This includes avoiding practices like data fabrication or selective reporting.
- Reproducibility: The ability for other researchers to replicate studies based on the original data influences the credibility of the findings. Hence, documenting methodologies clearly is essential.
- Ethical data management: Proper methods must be in place for handling data throughout its lifecycle—from collection to storage and eventual sharing. This area has gained traction in recent years, emphasizing the importance of transparency and security.
Promoting ethical standards surrounds and envelops the entire process of confocal microscopy research. Keeping these considerations in check ensures that the advancements brought about by this imaging technique contribute positively to scientific discourse and societal welfare.
End
In the realm of scientific research, the conclusion acts as a vital cornerstone. Here, we consolidate the wealth of information explored throughout this article on confocal microscopy. The conclusion does not simply wrap up the discussion; it serves as a platform to stress the significance of confocal microscopy in diverse fields of study, notably in biology and medicine.
Recapitulation of Key Points
Throughout this article, we dived into the intricacies of confocal microscopy, shedding light on its historical journey, key components, and mechanisms.
- Historical Background: Understanding the evolution helps appreciate the technological advances that made confocal microscopy a mainstay in research labs.
- Key Components: From laser light sources to optical pinholes, each component plays a critical role in producing high-resolution images.
- Advantages: Features like enhanced resolution and optical sectioning allow for unprecedented insights into cellular structures.
- Limitations and Challenges: Acknowledging the fragilities, like photobleaching and cost issues, is crucial for realistic expectations in research.
- Applications: The versatility of confocal microscopy across cell biology, neuroscience, and developmental biology highlights its pervasive influences.
- Innovations and Future Directions: Owing to advancements like multi-photon excitation, the future holds promise for richer imaging experiences and findings.
The Impact on Scientific Research
The significance of confocal microscopy transcends its mere functionality; it profoundly impacts scientific inquiry. By providing detailed images, it pushes the boundaries of what researchers can observe and analyze.
For instance, in cell biology, it's revolutionized our understanding of cellular dynamics and interactions at a level that was unimaginable with traditional light microscopy. Similarly, in neuroscience, imaging the intricate workings of neuronal connections can lead to breakthroughs in understanding brain diseases. The developmental biology field also benefits, as tracking the changes during organism growth can yield insights into developmental disorders.
"In the dream of discovery, confocal microscopy stands as the lens through which complex worlds unfold, revealing mysteries layer by layer."
Moreover, the ethical considerations surrounding data integrity and sample preparation ensure that researchers maintain the highest standards in their work. This diligence is essential in fostering trust among the scientific community and in the dissemination of reliable findings.