Exploring the Complexities of Bet Adsorption
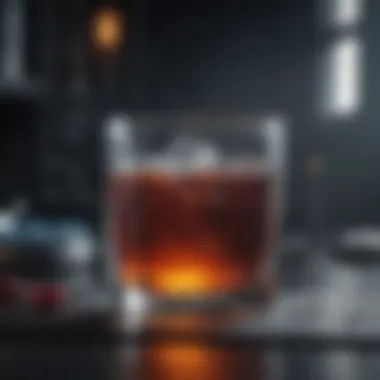
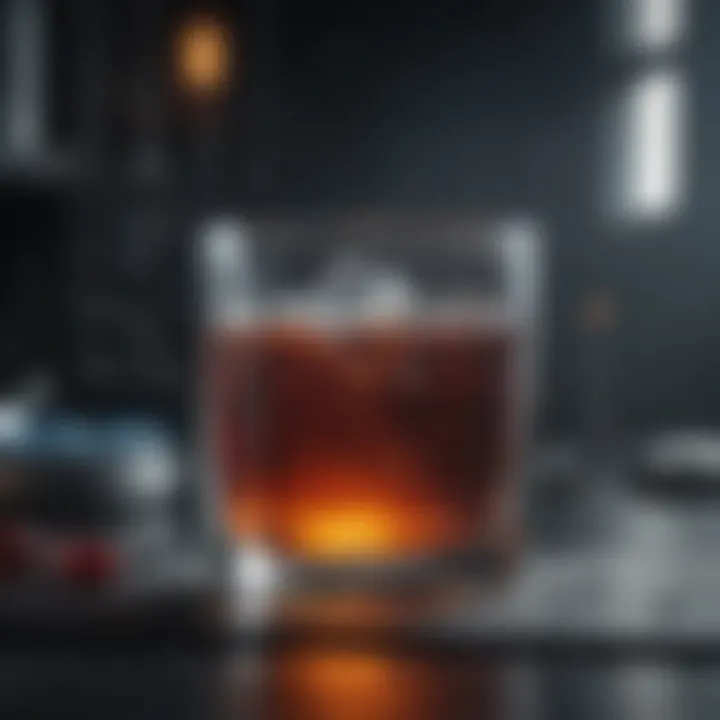
Research Overview
Bet adsorption plays a critical role in both chemistry and biological systems. This review aims to illuminate the concept, mechanisms, and diverse applications of bet adsorption. Understanding this phenomenon is vital to several fields, spanning environmental science to pharmacology. By examining various methodologies used to study bet adsorption, readers can grasp its significance in practical contexts.
Summary of Key Findings
The analysis reveals that bet adsorption is influenced by several factors, such as surface characteristics, temperature, and the nature of the adsorbate. Key findings from recent studies highlight that:
- Adsorption mechanisms include physical adsorption and chemical adsorption, each with distinct pathways and energy considerations.
- Types of bet adsorption can be generally classified into monolayer and multilayer adsorption, depending on the arrangement of adsorbates on the surface.
- Real-world applications extend from enhancing reactions in catalysts to understanding drug delivery systems.
These findings underscore the relevance of bet adsorption across diverse scientific arenas, offering insights into optimizing processes.
Methodologies Employed
Various methodologies have been utilized to study bet adsorption extensively. These include:
- Gravimetric methods: These involve measuring the weight change of the adsorbent before and after adsorption.
- Isotherm studies: The Langmuir and Freundlich isotherms are pivotal in understanding how adsorbates interact with a surface under equilibrium conditions.
- Spectroscopic techniques: Methods like IR spectroscopy help in identifying surface interactions at a molecular level.
Each methodology brings unique advantages, shaping our understanding of how bet adsorption operates in different scenarios.
In-Depth Analysis
Detailed Examination of Results
The results from recent experiments show a significant correlation between surface area of adsorbents and their capacity for bet adsorption. For instance, materials with high surface area, such as activated carbon or zeolites, exhibited superior efficiency in capturing adsorbates compared to those with lower surface areas. This relationship is crucial for the development of more effective filtration systems and catalytic converters.
Furthermore, temperature variations impact adsorption dynamics, with increased temperatures typically reducing adsorption due to enhanced kinetic energy disrupting the interactions. This observation is especially relevant in industrial applications where temperature control is essential.
Comparison with Previous Studies
Comparative analysis with earlier research highlights advancements in methodology and insights gained in the field over the years. Previous studies were often limited by less sophisticated measurement techniques, leading to less accurate data on adsorption behaviors. Modern techniques, however, offer detailed kinetics and thermodynamics.
Recent studies indicate that the integration of nanotechnology into adsorbent materials has opened new pathways, enhancing adsorption performance significantly compared to traditional methods. This progress illustrates a clear trajectory towards more efficient applications in both environmental cleanup and pharmaceuticals.
"The development of advanced adsorbents could reshape how we think about environmental remediation."
Preamble to Bet Adsorption
Bet adsorption is a crucial topic within the realms of both chemistry and biology. Understanding this phenomenon is essential for various scientific applications, including catalysis, environmental science, and material development. As researchers and practitioners delve into the intricacies of bet adsorption, they uncover not only fundamental principles but also their far-reaching implications in real-world scenarios. This importance stems from the role bet adsorption plays in facilitating interactions between molecules and surfaces, hence influencing various processes such as reaction kinetics and product stabilization.
Defining Bet Adsorption
Bet adsorption refers to the process by which adsorbate molecules adhere to a surface or interface. This process can be influenced by several factors, including temperature, pressure, and the chemical nature of the interacting entities. Specifically, it involves two key stages: the formation of a physical or chemical bond between the adsorbate and the substrate, followed by the stabilization of this bond. A clear definition is vital as it forms the foundation for understanding subsequent mechanisms and applications associated with this phenomenon.
In a simplistic context, one can view bet adsorption as the capacity of surfaces to attract and retain specific molecules. This characteristic is governed by various interactions, such as van der Waals forces in physical adsorption and covalent bonding in chemisorption. Thorough comprehension of these principles is pivotal for advancements in material sciences and nanotechnology.
Historical Background
The study of bet adsorption originated from early investigations in physical chemistry and materials science. Historically, significant milestones can be traced to the development of adsorption theories in the late 19th and early 20th centuries. Key figures such as Langmuir and BET (Brunauer, Emmett, and Teller) established foundational models that described adsorption isotherms and the behavior of multilayer adsorption on surfaces.
Over the decades, the evolution of analytical techniques has enabled deeper insights into the complexities of bet adsorption. The application of spectroscopic methods and surface analysis techniques has advanced understanding, making it possible to study adsorption at the molecular level. This progression illustrates how the historical context of bet adsorption has paved the way for contemporary research and applications.
"Understanding bet adsorption is critical for innovations in catalysts and nanomaterials, impacting both industry and environmental sustainability."
Mechanisms of Bet Adsorption
Understanding the mechanisms of bet adsorption is pivotal because they provide insights into how substances interact at the molecular level. The mechanisms govern the efficiency and effectiveness of adsorption processes across various fields, including catalysis, environmental science, and material development. Recognizing these mechanisms allows researchers to optimize conditions for desired outcomes in practical applications. Each mechanism, whether physical or chemical, offers unique perspectives and implications for controlling adsorption phenomena.
Physical Mechanisms
Physical mechanisms of bet adsorption primarily involve non-covalent interactions. These forces include van der Waals forces, hydrogen bonds, and electrostatic attractions. Physisorption is typically characterized by a lower energy change compared to chemisorption.
In physisorption, molecules adhere to a surface due to weak intermolecular forces. This type of adsorption is notable because it is often reversible, allowing molecules to detach and reattach without significant energy input. The strength of physisorption is dependent on surface roughness and molecular size.
- Characteristics of Physical Mechanisms:
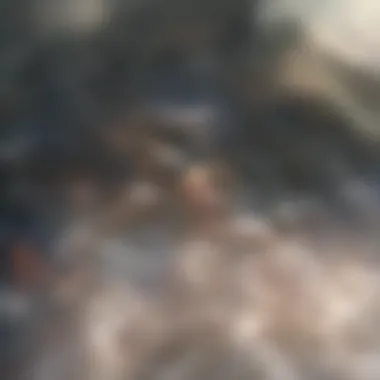
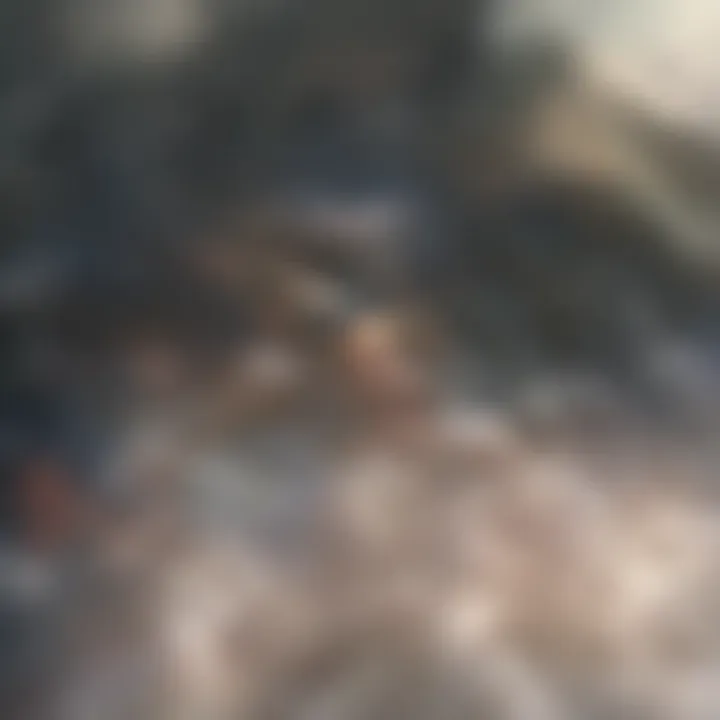
- Low energy changes during adsorption.
- Reversible process, allowing rapid desorption.
- Influenced by temperature and surface characteristics.
The significance of physical mechanisms in bet adsorption is highlighted through its applications in gas storage, where physisorption is employed to capture and release gases efficiently. For instance, materials like activated carbon rely on physisorption to adsorb a large amount of gas due to their high surface area. This property is advantageous in various applications, from air purification to energy storage.
Chemical Mechanisms
Chemical mechanisms of bet adsorption, or chemisorption, involve the formation of stronger chemical bonds between the adsorbate and adsorbent. This process entails a higher energy change compared to physical adsorption. Chemisorption is generally characterized by a specific orientation of molecules at the surface, leading to a higher energy barrier for desorption.
Chemisorption is essential because it affects the reactivity of surfaces in catalytic applications. The formation of covalent or ionic bonds can lead to the alteration of the electronic properties of both the adsorbent and adsorbate.
- Key Features of Chemical Mechanisms:
- Requires significant energy input to break the bonds.
- Often irreversible, facilitating permanent attachment.
- Strongly influenced by temperature and pressure.
The implications of chemisorption are critical in fields such as heterogeneous catalysis, where the interaction between reactants and catalyst surfaces determines the rate of reaction. Understanding chemical mechanisms allows chemists to enhance catalytic efficiency by optimizing surface properties and reaction conditions.
"The distinction between physical and chemical mechanisms in bet adsorption is fundamental for scientists looking to harness and manipulate adsorption processes for advanced applications."
Types of Bet Adsorption
Understanding the various types of bet adsorption is crucial because these mechanisms determine how substances interact at interfaces. The classification into physisorption and chemisorption helps in predicting and optimizing adsorption behavior for different applications. This distinction is essential in fields such as catalysis, material development, and environmental science. Each type offers unique benefits that can be harnessed in specific scientific contexts.
Physisorption
Physisorption is characterized by the weak van der Waals forces that hold molecules together at surfaces. These interactions are relatively weak compared to chemical bonds, allowing for reversible adsorption. A key feature of physisorption is its dependence on intermolecular forces rather than specific chemical bonding.
The following aspects are significant in physisorption:
- Low Energy Requirements: Physisorption occurs with minimal energy input. This allows for easy desorption under slight changes in conditions, making it suitable for applications where a reversible process is desirable.
- Temperature and Pressure Dependence: The rate and extent of physisorption can be influenced by both temperature and pressure. Generally, low temperatures enhance adsorption, while increasing pressure typically increases the amount of adsorbate retained.
- Broad Applicability: Physisorption applies to a variety of materials, including gases and liquids on solid substrates. This flexibility makes it a valuable process in many industrial applications.
Physisorption plays a significant role in gas separation, storage (such as hydrogen storage systems), and adsorption chillers.
Chemisorption
Contrasting with physisorption, chemisorption involves the formation of stronger chemical bonds between the adsorbate and the adsorbent. This type requires a higher energy input, leading to a generally irreversible process. The nature of the chemical bond can vary but typically includes covalent or ionic bonds.
Key elements of chemisorption include:
- Chemical Stability: Once adsorbed, chemisorption tends to be more stable than physisorption. This stability can be advantageous in processes requiring long-term retention of substances, such as catalyst surfaces in chemical reactions.
- Saturation: Chemisorption can reach saturation quickly. Once all available sites are bonded, no additional adsorbates can attach. This characteristic can limit the efficiency of storage systems if not designed correctly.
- Specificity: The interactions in chemisorption are specific. Different materials and conditions will yield different results in terms of adsorption capacity and rates. Understanding this can help optimize designs for chemical reactors and sensors.
Examples of chemisorption include catalytic converters in automobiles, where active sites on catalysts permanently bind reactant molecules, facilitating chemical transformations.
"The balance between physisorption and chemisorption is vital in designing efficient catalytic processes and adsorption systems."
Understanding these two types of bet adsorption provides a foundation for further exploration into their implications and applications in real-world scenarios.
Factors Affecting Bet Adsorption
Understanding the factors that affect bet adsorption is essential for scientists and researchers working in this domain. These factors govern how and why adsorption occurs, which in turn impacts various applications in fields like chemistry, materials science, and environmental science. Knowing these factors allows researchers to manipulate conditions for optimal results, leading to efficient processes.
Temperature Effects
Temperature plays a crucial role in bet adsorption. As temperature increases, the kinetic energy of molecules also increases. This can lead to several outcomes:
- Enhanced Kinetics: Higher temperatures typically lead to faster adsorption rates, as molecules move more energetically and collide more frequently with the surface.
- Desorption Risk: However, if the temperature exceeds a certain threshold, it can cause desorption, where adsorbed molecules leave the surface. This balance must be carefully managed in practical applications.
- Thermodynamic Implications: The adsorption isotherm can also shift with temperature changes. This indicates that different temperatures can affect the capacity of a material to adsorb.
Maintaining optimal temperature conditions in experiments and processes is critical to achieving the desired adsorption outcomes.
Pressure Influence
Pressure is another significant factor affecting bet adsorption, especially in gaseous systems. Its effects can include:
- Direct Correlation: For gases, increasing pressure usually enhances the amount adsorbed. This is described by various adsorption models that account for the pressure applied.
- Phase Behavior: It can also alter the phase behavior of adsorbates. In some cases, increasing pressure can lead to phase transitions that change how molecules interact with adsorbent surfaces.
- Material Specific: Different materials will respond uniquely to pressure. For example, zeolites may show different adsorption characteristics under varying pressures, making it essential to know the specific material properties involved.
In research and industry, understanding these pressure dynamics is vital for optimizing processes like gas separation and storage.
Surface Area Considerations
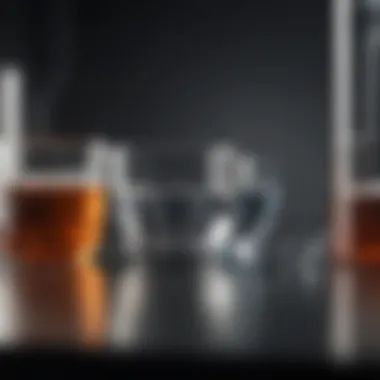
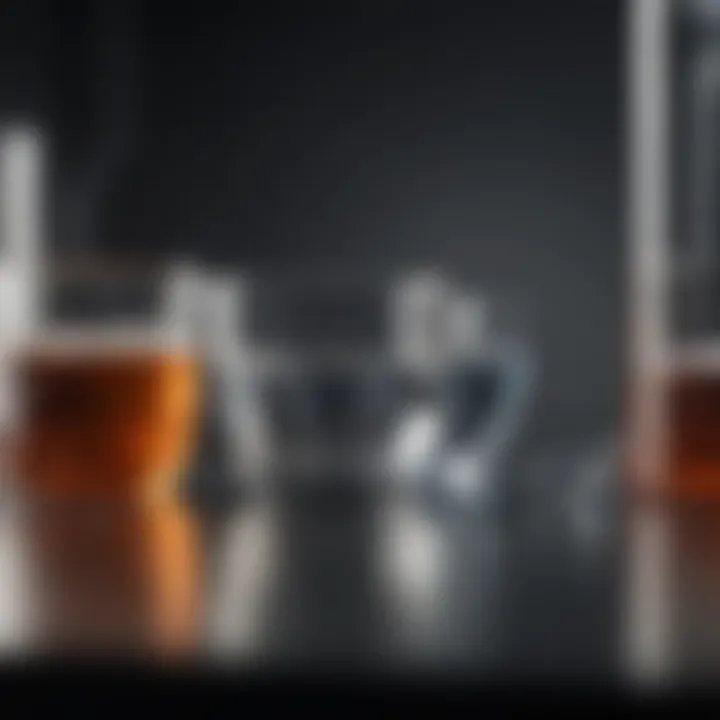
The surface area of an adsorbent material is a fundamental factor influencing bet adsorption. It directly relates to adsorption capacity and efficiency. Key points include:
- Greater Surface Area: Materials with a larger surface area can provide more sites for molecules to adsorb. This characteristic is often exploited in materials like activated carbon or silica gel.
- Porous Structures: The presence of pores also significantly impacts adsorption. Pore size and distribution can determine how effectively different substances can interact with the surface.
- Material Composition: The chemical composition of the adsorbent affects surface reactivity, further influencing adsorption characteristics.
An understanding of surface area and its implications is paramount for developing adsorbents tailored to specific applications, whether for catalysis or environmental cleanup.
Key Insight: The interplay of temperature, pressure, and surface area is essential for optimizing bet adsorption processes and obtaining reliable, reproducible results.
Instruments for Studying Bet Adsorption
Understanding bet adsorption is crucial in many scientific realms, particularly in chemistry and material science. The study relies heavily on various instruments that allow for detailed analysis of adsorption phenomena. These instruments help researchers identify the mechanisms at play, quantify adsorption capacity, and explore the properties of adsorbates and adsorbents. Choosing the right instrument can significantly improve the accuracy of the data obtained and influence subsequent research outcomes.
Spectroscopic Methods
Spectroscopic techniques are vital in studying bet adsorption because they provide insight into molecular and atomic interactions. These methods include Nuclear Magnetic Resonance (NMR), Infrared (IR) Spectroscopy, and Raman Spectroscopy. Each of these methods reveals different aspects of the adsorption process.
- NMR is particularly useful for understanding the chemical environment around specific nuclei within a molecule. It can highlight changes in molecular structure before and after adsorption.
- IR Spectroscopy helps in identifying functional groups involved in adsorption. It detects changes in vibrational modes that occur when molecules bind to surfaces.
- Raman Spectroscopy is complementary to IR and can provide additional information on molecular vibrations and symmetries.
These spectroscopic methods allow researchers to derive correlations between molecular structure and adsorption behavior, enhancing their overall understanding of the phenomena.
Surface Analysis Techniques
Surface analysis techniques are equally important when studying bet adsorption. These methods evaluate the physical and chemical properties of surfaces where adsorption takes place. Prominent methods include Scanning Electron Microscopy (SEM), Atomic Force Microscopy (AFM), and X-ray Photoelectron Spectroscopy (XPS).
- SEM provides high-resolution images of surface topographies, allowing scientists to observe how adsorbates spread on surfaces.
- AFM adds dimension by enabling three-dimensional imaging with atomic resolution, revealing minute changes in surface morphology after adsorption.
- XPS gives elemental composition data and chemical state information of the adsorbent surface, which is crucial to understand changes after adsorption.
By applying these surface analysis techniques, researchers gain detailed insights into how adsorbent surfaces interact with various materials, assisting in the development of more efficient adsorbents.
Other Analytical Methods
Beyond spectroscopic and surface analysis, other analytical methods also contribute to the understanding of bet adsorption. Techniques such as Thermogravimetric Analysis (TGA) and Gas Chromatography (GC) play significant roles.
- TGA measures changes in weight as a function of temperature, allowing researchers to gauge the thermal stability of adsorbates and the extent of adsorption.
- GC provides quantitative data on how much adsorbate is removed from the gas phase, which can correlate directly to the adsorption capacity of a material.
These methods offer complementary data that strengthen the overall analysis of adsorption processes, validating findings across multiple techniques.
"In the world of scientific research, the choice of instruments can dictate the quality and relevance of the results obtained."
The integration of these diverse methods forms a comprehensive toolkit for scientists and researchers. Understanding and utilizing advanced instruments for studying bet adsorption is key to unlocking its complexities and expanding its applications in real-world scenarios.
Applications of Bet Adsorption
The concept of bet adsorption plays a crucial role in multiple scientific disciplines. It serves as a bridge between basic research and real-world applications, facilitating advancements that benefit various sectors. Understanding how bet adsorption functions can lead to significant improvements in processes such as catalysis, environmental remediation, and material science. Recognizing the applications can inspire future innovations, making bet adsorption a key area of study for students, researchers, educators, and industry professionals.
Catalysis
Catalysis is one of the most prominent applications of bet adsorption. It refers to the process wherein reaction rates are enhanced by a substance that remains unchanged post-reaction, known as a catalyst. In many catalytic processes, the efficiency is directly tied to the adsorption characteristics of the reactants on the catalyst surface. For instance, heterogeneous catalysis commonly utilizes solid catalysts where reactants adsorb onto their surfaces. This process alters the reaction's pathway, leading to reduced energy requirements and faster rates of product formation. Key areas where catalysis via bet adsorption has major implications include:
- Industrial Synthesis: Processes like ammonia synthesis use iron-based catalysts, employing adsorption to increase the contact time of reactants.
- Petroleum Refining: Catalytic cracking employs zeolites, where hydrocarbon molecules adsorb and then transform into valuable products.
- Environmental Catalysis: Many technologies rely on bet adsorption to reduce harmful emissions, such as catalytic converters in cars that convert pollutants into less harmful emissions.
Environmental Remediation
Environmental challenges often necessitate innovative solutions. Here, bet adsorption emerges as a pivotal technique in the removal of contaminants from soil and water. By employing various adsorbents, including activated carbon and bio-sorbents, pollutants can be effectively captured. This method is essential in treating hazardous waste and restoring contaminated environments. Some notable applications are:
- Wastewater Treatment: Adsorption methods efficiently remove heavy metals and organic compounds, improving water quality before discharge or reuse.
- Air Purification: Adsorbent materials can capture airborne pollutants, volatile organic compounds, and odors, enhancing indoor air quality.
- Soil Decontamination: Using bio-sorbents, such as algae or plant-derived adsorbents, can help extract toxic metals from contaminated soils, facilitating ecological recovery.
Material Science
In material science, bet adsorption is essential for developing new materials and improving existing ones. The interactions between materials can be manipulated to create composites with desirable properties. For example, the adsorption capacity of a given material helps dictate its suitability for applications in sensors, membranes, and catalysts. Some primary uses include:
- Nanomaterials Development: Nanoscale materials often exhibit unique adsorption properties, leading to advancements in drug delivery systems and diverse electronics.
- Coating Technologies: Surface coatings in various industries benefit from controlled adsorption characteristics to improve durability and performance.
- Smart Materials: These materials utilize adsorption phenomena to react or adapt to environmental stimuli, broadening their functionality in everyday applications.
By embracing the principles of bet adsorption, researchers can unlock innovative solutions that address both contemporary issues and future challenges across diverse disciplines.
End
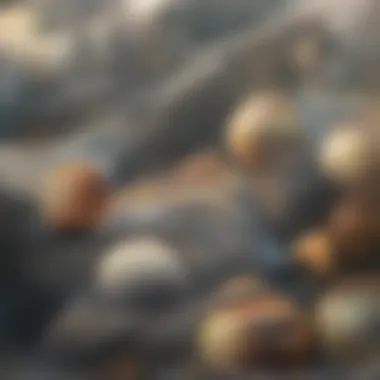
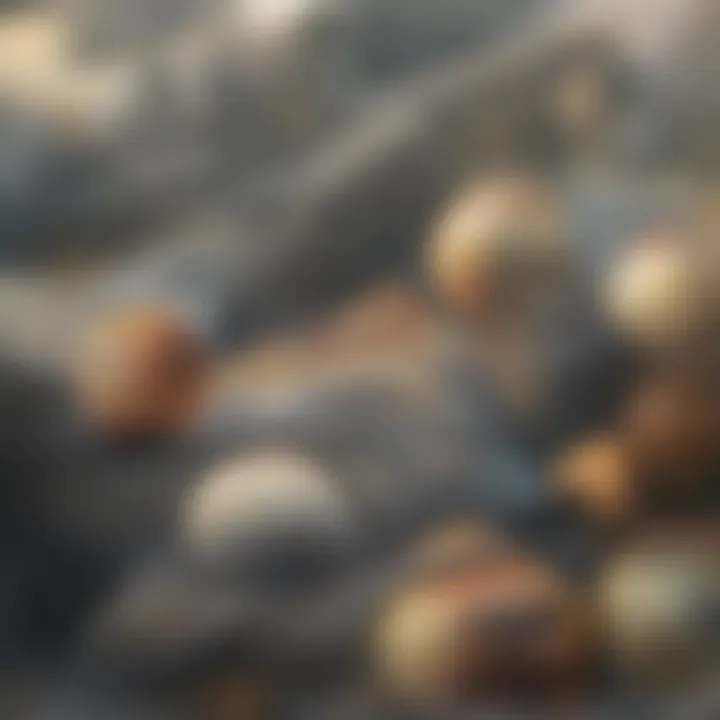
The applications of bet adsorption cut across various fields, showcasing its broad relevance. In catalysis, environmental remediation, and material science, understanding the underlying adsorption principles can lead to significant advancements. Continued research in these areas not only enhances our knowledge but also provides practical solutions that can benefit society at large. Accessibility to this knowledge can inspire future generations to push the boundaries of current technologies.
Challenges in Bet Adsorption Research
The field of bet adsorption research presents several challenges that require attention and innovation. These challenges are crucial for advancing our understanding of the phenomenon and for applying findings in practical contexts. Acknowledging these hurdles not only highlights the complexity of the subject but also emphasizes the need for rigorous research methodologies and thoughtful interpretation. The discussion on challenges can guide future scholars in focusing their efforts effectively.
Data Interpretability Issues
One of the primary challenges in bet adsorption research is the interpretability of data. This complexity arises from several contributing factors. The nature of data gathered from adsorption experiments is often multifaceted, influenced by various external conditions such as temperature and pressure. Inconsistencies in experimental results can occur due to variations in materials and techniques used.
A significant aspect of data interpretability is related to the modeling techniques employed. Many researchers rely on a set of mathematical models to predict adsorption behavior. However, the assumptions embedded in these models may not always be valid for every system. This discrepancy could lead to misinterpretation of results and hinder the development of universally applicable theories.
Furthermore, the lack of standardization in experimental protocols compounds these challenges. Without a universally accepted methodology, comparing results across different studies becomes problematic. Researchers may use different techniques for measuring parameters, which complicates efforts to create a cohesive understanding of the phenomena.
Modeling Challenges
Another intricate challenge is the modeling of bet adsorption processes. Several models exist to describe adsorption mechanisms, including Langmuir and Freundlich isotherms. While these models provide a framework for understanding adsorption behavior, they also have limitations.
The oversimplification of real conditions can lead to inaccuracies in predictions. Factors such as heterogeneous surface properties, competing adsorption, and multilayer adsorption can result in significant deviations from the expected outcomes based on ideal model assumptions. Addressing these complexities is essential for improving the reliability of model predictions.
Moreover, the dynamic nature of environments where adsorption occurs can further complicate modeling efforts. For instance, in environmental remediation applications, fluctuations in contaminant concentrations and varying material properties can alter the adsorption dynamics significantly.
Challenges in modeling are not merely a technical issue; they also have broader implications. If models do not accurately reflect the adsorption behavior, it can lead to failed applications in fields such as catalysis and material design. Therefore, a more nuanced approach to modeling that considers real-world complexities is necessary for advancing research in this area.
"Addressing the challenges in bet adsorption research is essential for unlocking its full potential in various applications."
Future Directions in Bet Adsorption Studies
The study of bet adsorption continues to evolve, revealing new opportunities and challenges. Future directions are vital to enhance the understanding of this complex phenomenon. Emphasizing innovative techniques and interdisciplinary collaborations hold significant promise for expanding our knowledge base. Here, we explore emerging methods and the value of collaborative approaches.
Emerging Techniques
One primary focus for future research lies in developing and refining techniques used to analyze and measure bet adsorption.
- Nanotechnology: Advances in nanomaterials allow researchers to enhance surface properties. For instance, nanosized adsorbents could significantly increase the efficiency of adsorption processes.
- Machine Learning: By leveraging machine learning, scientists can model complex interactions more accurately. This can lead to more effective predictions of adsorption behavior under various conditions.
- High-Throughput Screening: Development of high-throughput techniques can expedite the evaluation of adsorbent materials. This can help identify optimal candidates for specific applications much quicker than traditional methods.
As scientists embrace these emerging techniques, a greater understanding of the intricate details of bet adsorption will likely ensue. A focus on precision and efficiency can foster breakthroughs in application domains.
Interdisciplinary Approaches
Interdisciplinary approaches are crucial for advancing bet adsorption research. This allows for blending insights from various scientific fields, including:
- Chemistry and Material Science: Understanding the properties of materials at a molecular level enhances the design of better adsorbents.
- Physics: Insights on surface interactions and energy states can inform the optimization of adsorption processes.
- Biology: Knowledge from biological systems can inspire new avenues for developing environmentally friendly adsorbents. For example, mimicking natural processes might offer sustainable solutions to environmental remediation.
By fostering collaboration among disciplines, researchers can break traditional barriers. This synergy can lead to innovative solutions that address real-world challenges related to bet adsorption.
Collaboration is the key to unlocking new potentials in bet adsorption, as diverse perspectives drive creativity and innovation.
In summary, focusing on emerging techniques and interdisciplinary approaches offers a promising horizon for understanding bet adsorption. Continued exploration in these areas can yield significant advancements.
Finale
The conclusion serves as an essential component in emphasizing the significance of bet adsorption. It synthesizes the wealth of information discussed throughout the article, providing readers with a cohesive understanding of the subject. Recapping the mechanisms of adsorption, along with its diverse applications across chemistry and biology, highlights the multifaceted nature of this phenomenon.
Moreover, it underlines the importance of considering factors such as temperature, pressure, and surface area that affect adbsorption processes. By doing so, the final section not only reinforces the key points but also illustrates the practical implications of bet adsorption in real-world applications, whether it be in catalysis, environmental remediation, or material science.
"Understanding the intricacies of bet adsorption is pivotal for advancements in various scientific fields."
Reflecting on these points prompts a deeper appreciation for ongoing research, guiding future inquiries and potential breakthroughs.
Summary of Key Points
- Definition and Mechanism: Bet adsorption is defined as the process involving the adherence of molecules from a solution to a solid interface. It encompasses both physical and chemical mechanisms, each with unique characteristics.
- Types: The two main types, physisorption and chemisorption, differ fundamentally in terms of the forces involved and the energy changes during the adsorption process.
- Influences: A variety of factors such as temperature, pressure, and surface area significantly affect the adsorption efficiency and capacity.
- Instruments and Techniques: Several analytical methods, including spectroscopic and surface analysis techniques, are crucial for studying and understanding bet adsorption.
- Applications: The applications of bet adsorption range from catalysis to environmental cleanup and advancements in materials science.
- Challenges and Future Directions: There are intricate challenges within the field, particularly concerning data interpretation and model development, yet emerging techniques and interdisciplinary approaches point towards promising future avenues.
The Importance of Continued Research
Continuous research in bet adsorption remains vital for various reasons. First, as new materials and technologies emerge, understanding how molecules interact with surfaces becomes increasingly crucial. This interaction directly impacts fields such as catalysis, where the efficiency of chemical reactions can hinge on optimal adsorption characteristics.
In addition, environmental concerns necessitate innovative adsorption strategies for pollutant removal. Research in this area could lead to breakthroughs in reducing harmful substances from our ecosystems, thus protecting health and biodiversity.
Furthermore, interdisciplinary collaboration offers a robust framework to enhance understanding. Insights from chemistry, physics, biology, and engineering ensure that research remains nuanced and diverse, ultimately enriching the field of bet adsorption.
The strategic exploration of these topics will pave the way for innovative applications and sustainable practices, confirming that the study of bet adsorption is not just relevant, but essential.