BCI Prosthetics: Merging Neuroscience with Robotics
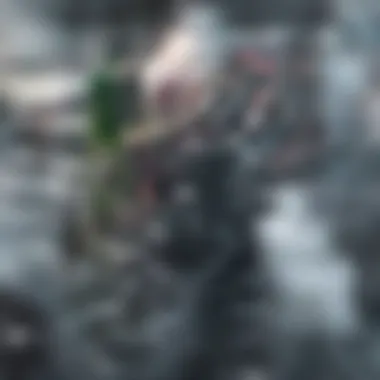
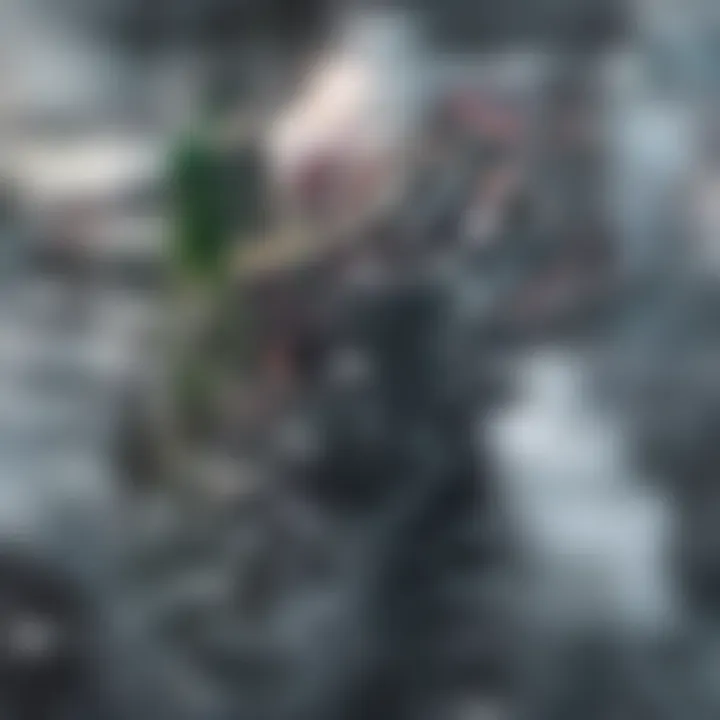
Intro
The integration of neuroscience and robotics has led to remarkable advancements in healthcare, particularly for individuals with limb loss. The development of brain-computer interface (BCI) prosthetics exemplifies this progress. BCIs enable direct communication between the brain and external devices, revolutionizing how prosthetic technology is utilized. As research in this field continues to evolve, understanding its foundations, current applications, and implications becomes essential.
This article aims to examine how BCI prosthetics work and the profound impacts they have on users. Through an exploration of neuroscience principles, technological innovation, and real-world applications, we seek to impart a comprehensive overview. Furthermore, ethical considerations and future possibilities will also be addressed.
Research Overview
Summary of Key Findings
Recent studies indicate that BCI technology significantly enhances the functional capabilities of prosthetics. Users often report improved control and adaptability of these devices compared to traditional options. Moreover, the synergy of neuroscience and robotics is creating more intuitive interfaces that respond to neural signals.
Key findings include:
- Increased User Agency: Much research underscores how BCI technology allows users to exert more control over prosthetic limbs, thus improving independence.
- Adaptation and Learning: Studies show that users can learn to control their prosthetics with more ease through feedback mechanisms, making the devices respond better to user intentions.
- Reduction in Phantom Limb Pain: Some research highlights that effective BCI use has potential to alleviate sensations associated with limb loss.
Methodologies Employed
A variety of methodologies have been adopted in researching BCI prosthetics. These often include:
- Clinical Trials: Testing BCI prosthetics on diverse patient groups to assess efficacy and usability.
- Neuroimaging: Utilizing techniques such as fMRI or EEG to analyze brain responses during prosthetic use.
- Machine Learning Algorithms: Implementing AI to interpret neural signals, thus improving device responsiveness.
In-Depth Analysis
Detailed Examination of Results
The exploration of results from recent studies provides valuable context regarding the efficacy of BCI prosthetics. Many trials demonstrate notable improvements in user experience post-implementation. For example, participants often exhibit enhanced dexterity and improved movement quality.
Comparison with Previous Studies
When compared to prior research, the findings of recent studies reflect substantial advancements. Earlier iterations of prosthetics focused primarily on basic mechanical functions. The latest BCI devices, however, emphasize creating a more seamless interaction with the userβs neural pathways.
"BCI prosthetics are not merely tools; they are extensions of the userβs body, fundamentally altering the perception of mobility and agency."
The ongoing development indicates that the field is actively addressing historical limitations. By investing in improved methodologies and technologies, researchers are paving the way for a future where prosthetic limbs are virtually indistinguishable from natural limbs in function and interaction.
Foreword to BCI Prosthetics
Brain-computer interface (BCI) prosthetics represent a significant advancement in the integration of neuroscience and robotics. This emerging field is vital for enhancing the quality of life for individuals with limb loss or paralysis. BCI systems bridge the gap between human intent and machine response, thus allowing users to control prosthetic limbs through their brain signals. Understanding BCI prosthetics is crucial, as it encompasses not only the technological aspects but also the potential benefits, limitations, and ethical considerations involved in developing these systems.
The intersection of neuroscience and robotics in BCI prosthetics addresses a broad range of issues. These issues include improving motor functions, advancing rehabilitation strategies, and fostering greater autonomy for users. The importance of BCI prosthetics extends beyond individual applications; they signify a fundamental shift in how we approach disability, rehabilitation, and human-machine interaction.
Definition and Overview
BCI prosthetics can be defined as advanced devices that facilitate direct communication between the brain and external devices, typically prosthetic limbs. These systems utilize brain signals to control the movements of prosthetic arms, hands or legs, replacing lost functionalities of natural limbs. By decoding neural activities and translating them into mechanical commands, BCI prosthetics represent a leap in assistive technologies.
In essence, these systems consist of electrodes implanted in the brain or placed on the scalp. The electrodes capture neural activity and transmit the data to a computer. Sophisticated algorithms then interpret these signals to control the prosthesis. As a result, users can execute movements, such as grasping or reaching, with a degree of independence previously thought unattainable.
Historical Context
The development of BCI technologies began in the late 20th century. The initial research focused primarily on understanding neural activity and how it could correlate to physical movements. Early experiments mostly involved animal subjects. It was not until the early 2000s, however, that BCI technology began to transition into practical applications for humans.
Groundbreaking projects, such as those at the University of California, Los Angeles, and the Tohoku University in Japan, pioneered the development of invasive BCIs, which involves implanting electrodes directly into the brain. These advances opened the door for more complex prosthetic control, but they also introduced ethical questions regarding safety and long-term effects on human subjects.
Meanwhile, non-invasive methods began to garner interest as they promise a safer alternative, minimizing risks associated with surgical procedures. Today, BCI prosthetics continue to evolve, fueled by the collaboration between neuroscientists, engineers, and clinicians aiming to optimize their capabilities and expand their applicability.
"The integration of BCI technology into prosthetics not only restores functionalities but also redefines the limits of human-machine interaction."
The Science Behind BCI Technology
Understanding the fundamental principles behind brain-computer interface (BCI) technology is crucial for grasping its implications in prosthetics. This section lays the groundwork for how BCIs function, detailing the mechanisms that connect neural activities to robotic systems. Knowledge in this domain not only enhances our technical know-how but also fosters innovation in prosthetics. The integration of neuroscience and robotics challenges existing paradigms and presents new avenues for rehabilitation and restoration of motor functions.
Neuroscience Foundations
The role of neuroscience in BCI technology is foundational. Neuroscience studies the nervous system, particularly the brain, which is the source of neural signals. In the context of BCI prosthetics, researchers focus on decoding these signals into actionable commands for prosthetic devices. Understanding neural pathways and activity is essential. Neurons communicate through electrical impulses and biochemical processes, signaling intentions for movement.
Neuroscience provides insight into how these signals can be interpreted. This process involves mapping brain regions responsible for various motor functions. For instance, motor cortex activity correlates with finger movements. Advances in neuroimaging techniques have enabled researchers to observe brain activity in real time, allowing for more accurate signal interpretation. The challenge, however, lies in the variability of brain signals across individuals. Thus, personalization becomes a significant component in developing effective BCI systems.
"Decoding brain signals to control external devices marks a substantial leap in assistive technology."
β Thought-provoking insight into BCIβs potential.
Electrode Technology
Electrode technology is a critical aspect of BCI systems. Electrodes are the interface between the brain and the prosthesis. They capture neural signals and transmit them for processing. There are various types of electrodes used, notably invasive and non-invasive electrodes.
- Invasive electrodes are implanted directly into the brain tissue. These electrodes can provide high-resolution data from individual neurons. Models like the Utah Array showcase this approach, offering a grid of electrodes that can map precise neural activities. However, this method carries risks, such as infection and the potential for harmful tissue responses.
- Non-invasive electrodes, such as those used in electroencephalography (EEG), offer a safer alternative by placing electrodes on the scalp. While they are easier to use, non-invasive techniques often suffer from lower signal resolution and are affected by external noise.
The choice of electrode type depends on the specific needs of the user and the complexity of the tasks intended for the prosthesis. Therefore, ongoing research into improving electrode design and functionality remains vital. The aim is to enhance signal clarity while maintaining user safety and comfort.
Overall, the intersection of neuroscience and electrode technology shapes the future of BCI prosthetics, highlighting the significance of meticulous research and development in creating effective and user-friendly devices.
Types of BCI Systems
Brain-computer interface (BCI) systems have gained traction in both scientific research and medical applications. Understanding the types of BCI systems is essential because each category serves specific needs and presents unique advantages and challenges. This section delves into two primary classifications: invasive and non-invasive BCIs. The implications of selecting one type over another can significantly affect user experience, application outcomes, and even developmental costs.
Invasive BCIs
Invasive BCIs involve surgical procedures where electrodes are implanted directly into the brain. This invasive nature allows for a high-resolution signal from neural activity. The primary advantage is that these systems can capture detailed brain signals, enabling precise control of prosthetic devices. Invasive BCIs have shown promising results in clinical settings, helping users regain some degree of lost functionality.
Noteworthy benefits include:
- Enhanced signal quality due to close proximity to neurons.
- Less interference compared to non-invasive methods.
- The potential for improved performance in executing complex tasks.
However, the invasive approach carries risks. Surgical complications, infection, and the bodyβs natural response to foreign materials are significant concerns. Compliance with rigorous safety protocols and ethical considerations regarding patient consent are critical in this context.
Non-Invasive BCIs
Unlike their invasive counterparts, non-invasive BCIs gather brain activity data without requiring surgery. Methods such as Electroencephalography (EEG) are typical in this category. Non-invasive BCIs come with distinct advantages, mainly their accessibility and reduced risk profile. This makes them more suitable for broader applications ranging from rehabilitation to gaming technology.
Benefits of non-invasive BCIs include:
- Easy setup, allowing for quicker deployment in various settings.
- Lower risk of complications since no surgical procedure is necessary.
- Greater potential for repeated use in research or therapeutic interventions.
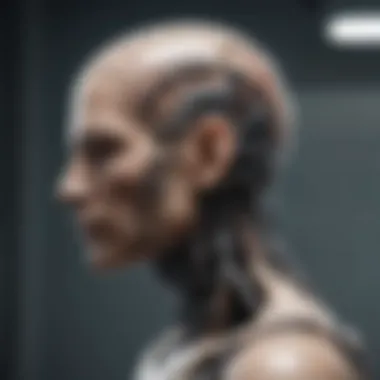
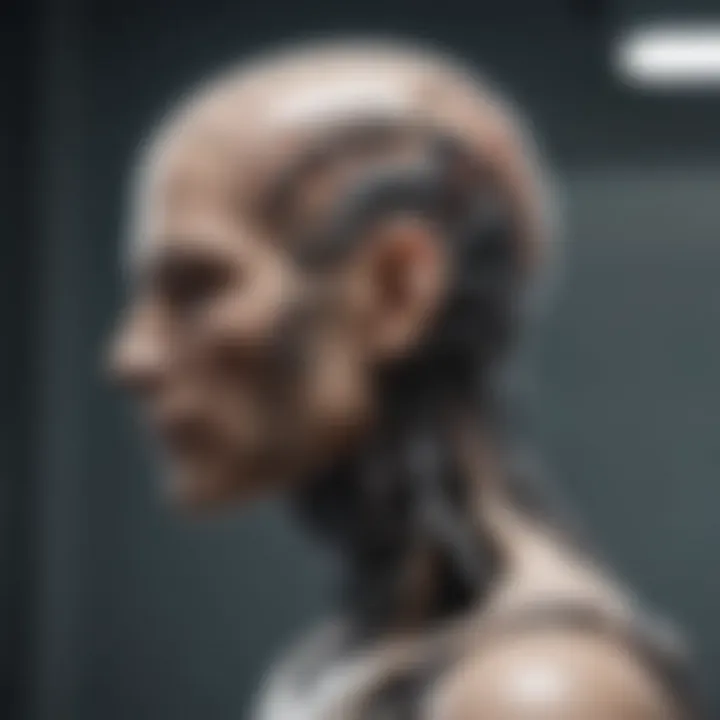
On the downside, the signal quality from non-invasive BCIs is generally less robust, often requiring advanced algorithms for effective signal processing. The spatial resolution is not as high, meaning user control may be less accurate.
In summary, the choice between invasive and non-invasive BCIs hinges on the specific context of use, desired precision, and risk tolerance. Each type contributes uniquely to the evolution of BCI prosthetics, paving the way for more integrated neural interfaces in the future.
Applications of BCI Prosthetics
The exploration of applications within BCI prosthetics is essential to understand the transformative impact of this technology on individuals with limb loss. BCI prosthetics enable seamless interaction between neural signals and devices, allowing users to regain functionality and independence. The potential applications stretch across various fields, most notably medicine and rehabilitation, where the focus is on enhancing motor functions and improving quality of life.
Current Uses in Medicine
In the field of medicine, BCI prosthetics offer revolutionary solutions. One of the most prominent applications is in the restoration of movement for individuals who have lost limbs due to injury or illness. These systems utilize neural signals to control prosthetic devices directly. For instance, a user can think about moving their arm, and a well-designed prosthetic equipped with brain-computer interface technology interprets these signals, translating them into movement.
Key benefits include:
- Enhanced Mobility: Users can perform tasks such as grasping objects or manipulating tools much like natural limbs.
- Psychological Benefits: The ability to regain control can significantly uplift mental health, providing a sense of agency.
- Personalized Interfaces: Advances in BCI allow devices to be tailored to individual user preferences, improving usability and satisfaction.
These medical applications are not only about improving physical capabilities. They also entail significant implications for emotional and psychological recovery, showcasing the holistic advantages of BCI technology in prosthetics.
Advancements in Rehabilitation
The landscape of rehabilitation has also shifted significantly with the integration of BCI prosthetics. Rehabilitation programs now utilize BCI systems to enhance recovery outcomes through better engagement and tailored therapies. One of the prominent advancements includes real-time feedback mechanisms, which use neural data to adjust therapy strategies based on user needs.
Some important points regarding advancements in rehabilitation:
- Adaptive Learning: BCI systems can adapt to the user's progress, offering increasing levels of challenge as abilities improve.
- Motivational Aspects: Modern BCI technologies can gamify rehabilitation exercises, inspiring users to engage more actively in their recovery process.
- Data-Driven Insights: Enhanced data collection from BCI devices provides better insights into motor recovery patterns, aiding professionals in designing effective therapeutic interventions.
The trajectory of rehabilitation is now more data-informed, promoting a user-centric approach. As technologies evolve, these developments signify a promising future for BCI prosthetics and their applications in medicine and therapy.
"Advancements in BCI technology offer not only restoration of function but also an avenue for psychosocial healing for amputees."
The applications of BCI prosthetics thus showcase the profound impact this technology has on enhancing both physical capabilities and quality of life.
Enhancing Motor Control through BCIs
Enhancing motor control through BCIs is a significant aspect in the development of brain-computer interface prosthetics. Motor control refers to the ability to regulate and direct movements of muscles to perform tasks effectively. In the context of BCI technology, improving motor control can lead to restored independence for individuals with limb loss or severe motor impairments. This enhancement not only offers practical benefits but also impacts the quality of life for users.
The integration of neural signal decoding and real-time feedback mechanisms plays a vital role in this process. By translating neural impulses into actionable commands for prosthetic devices, BCIs enhance the user's ability to control these devices seamlessly. The ultimate goal is to enable intuitive and precise movement, minimizing the cognitive load required to operate the prosthetics.
Moreover, improving motor control requires an understanding of the variations in neural activity across different individuals. This means that flexibility in BCI design is crucial to accommodate varying levels of neural signals and user capabilities. As BCIs advance, the potential for enhanced motor control will only grow, paving the way for more natural interactions with prosthetic devices.
Decoding Neural Signals
Decoding neural signals forms the backbone of BCI technology, allowing the translation of thoughts into movements. This process involves capturing electrical activity from the brain, which can then be interpreted into command signals for prosthetic devices. Through techniques such as electrocorticography or EEG, researchers are able to identify specific patterns and signals related to intended movement.
Understanding how different neural patterns correlate with specific movements is a complex task. However, recent advancements in machine learning and artificial intelligence have shown promising results in improving the accuracy of neural signal decoding. By training algorithms to recognize these complex patterns, BCIs can achieve greater precision in translating thoughts into commands, improving the functionality of prosthetics.
Accurate neural decoding is critical for the effectiveness of BCI prosthetics, helping users regain autonomy in their movements.
Feedback Mechanisms
Feedback mechanisms are essential in BCIs as they provide users with real-time information about their movements. Effective feedback can come in various forms, such as visual, auditory, or tactile cues, helping users adjust their movements for better control. The integration of feedback mechanisms enhances the interactive experience, allowing users to become more attuned to their prosthetic devices.
Research indicates that effective feedback can significantly improve user performance and satisfaction. For instance, when users receive immediate feedback about their movements, they are more likely to make necessary adjustments, leading to better outcomes.
The development of closed-loop systems, where motor commands and sensory feedback work together, represents a significant leap toward enhancing motor control. These systems provide a continuous feedback loop that helps users refine their control strategies, leading to more natural and fluid movements.
The field continues to evolve, with ongoing research focused on precision in both decoding signals and delivering feedback, aiming for an optimal user experience.
User-Centric Design in BCI Prosthetics
User-centric design is critical in the field of BCI prosthetics. It ensures that devices are not only functional but also truly meet the needs of the users. As technology advances, the expectation for prosthetics goes beyond mere mechanical replacements. They should enhance independence, restore functionality, and fit seamlessly into the user's lifestyle.
Personalization and Adaptation
Personalization in BCI prosthetics involves tailoring devices to fit individual users. Each person's neurological and physical condition varies. Therefore, customization is vital. Factors such as limb loss level, user environment, and personal preferences influence how a prosthetic device should be designed.
Adaptation occurs over time. Users may grow more accustomed to their devices as they learn how to control them effectively. This requires systems that can learn and adjust to their userβs movements and preferences. For instance, software in the BCI can adapt to the neural signals of the user, making the experience more intuitive.
Some methods to achieve personalization include:
- User Feedback: Collecting input directly from users helps in modifying the design process according to real-world experiences.
- Modular Designs: Prosthetics can be made modular, allowing users to change components based on their activities or needs.
- Adaptive Learning Algorithms: Using algorithms that evolve with user commands leads to improved control over time.
By implementing these methods, manufacturers can create prosthetics that feel like a natural extension of the userβs body, increasing comfort and usability.
User Experience Studies
User experience studies play a pivotal role in designing effective BCI prosthetics. They assess how real users interact with the devices. A strong focus on these studies can identify pain points and areas for improvement.
Understanding the user experience involves:
- Observational Research: Watching users interact with their prosthetic devices provides insight into functioning and areas of difficulty.
- Surveys and Interviews: Gathering qualitative data through user surveys and interviews helps gain deeper insights into user satisfaction and practical issues they face.
- Longitudinal Studies: Studying users over an extended period reveals changes over time and the adaptation process.
The findings from these studies can lead to iterative design changes that enhance the overall interface and usability. When user feedback is integrated, the resulting prosthetics are more likely to align with user expectations and lead to better adaptation and satisfaction.
User-centric design in BCI technology is not just about technology advancement; it is about ensuring that technology works for the users, facilitating their journey of adaptation and functionality.
Ethical Considerations in BCI Development
As brain-computer interface (BCI) technology evolves, so too do the ethical considerations surrounding its development and use. These issues intersect with multiple domains, including neuroscience, technology, and human rights. Understanding the ethical landscape is vital for stakeholders involved in BCI development, as it ensures that advancements are made responsibly and with respect for the users' rights and dignity.
One significant aspect of the ethical discourse involves the privacy and data security of neural information. Since BCIs interact with sensitive neural activity, there is a heightened need for policies protecting user data. This is essential not only to safeguard personal information but also to build trust between users and developers.
Furthermore, regulatory frameworks play a critical role in establishing boundaries and standardizing practices within the BCI space. Effective regulation can ensure that BCI technology is not only safe but also ethically sound. By fostering clear guidelines, we can facilitate more effective collaboration among researchers, engineers, and ethicists.
In summary, attending to the ethical considerations in BCI development is fundamental for creating advancements that serve the greater good while promoting user dignity and privacy. The following subsections will explore these topics in greater detail.
Privacy and Data Security
Privacy is paramount in the BCI field, where the collection and interpretation of neural data can reveal deeply personal insights about an individual. As BCIs gather data that can be classified as sensitive, there is an urgent need to establish robust measures to protect this information.
- Informed Consent: Users should be fully aware of what data is collected and how it is used. This ensures that they can make an informed decision about their involvement.
- Data Encryption: Safeguarding data through strong encryption is necessary to prevent unauthorized access. Ensuring data security not only protects users but also enhances the overall credibility of BCI technology.
- User-Controlled Data: Allowing users choice in how their data is used or shared can foster empowerment and enhance trust in the technology.
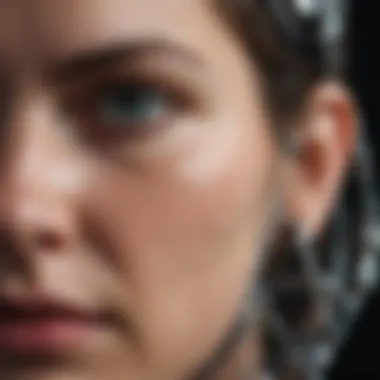
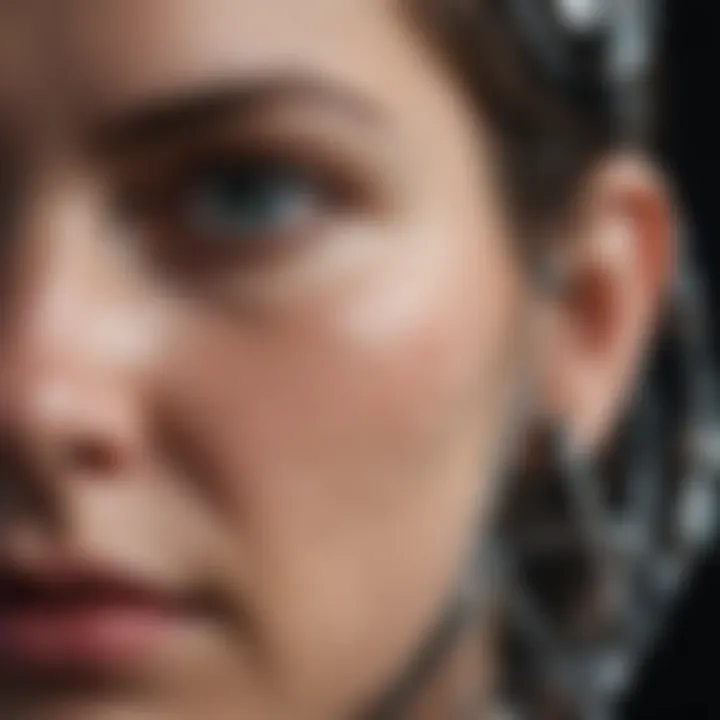
"The intersection of ethics and BCI technology reflects our commitment to ensuring that innovation does not come at the cost of individual rights."
Regulatory Frameworks
Regulatory frameworks are essential for guiding BCI development in ethical and safe directions. These policies must adapt to the fast-paced changes in technology while ensuring that user safety and ethical standards are upheld.
Key components of effective regulatory frameworks include:
- Standardization of Practices: Establishing benchmarks for safety and efficacy ensures that all developers meet a minimum safety threshold.
- Oversight Bodies: Regulatory agencies like the FDA in the United States must be engaged in reviewing BCI technologies to expedite approvals while ensuring thorough evaluations.
- Impact Assessments: Conducting regular assessments of the social and ethical impacts of BCIs can identify potential issues early on, promoting responsible development.
Such frameworks not only protect users but also help foster a culture of accountability among companies working in the BCI space, ensuring innovations benefit society as a whole.
Challenges in BCI Technology
The development of brain-computer interface (BCI) prosthetics is an exciting field. However, it is not without its challenges. Addressing these challenges is crucial for advancing BCI technology, improving user experience, and ensuring effective integration with existing systems. This section will delve into the specific issues faced in BCI technology, focusing on technical limitations and integration concerns.
Technical Limitations
Technical limitations pose significant hurdles in the progress of BCI prosthetics. The efficacy of these devices relies heavily on accurately decoding neural signals, which is inherently complex. Various factors come into play, including signal noise, variability in brain patterns, and the sophistication of the algorithms used for signal processing.
One major limitation is the resolution and precision of electrodes used to capture neural activity. Invasive electrodes, while offering high-resolution data, come with risks such as infection and tissue damage. On the other hand, non-invasive electrodes provide a safer alternative but typically yield lower quality signals, which affects performance.
Moreover, the adaptability of current BCI systems to different user conditions requires improvement. Each individual's brain activity is unique, and tailoring BCI devices to personalize functionality can be time-consuming and often requires extensive training of the algorithms. This can create a barrier for users seeking immediate assistance and usability.
In summary, technical limitations in signal capture, processing, and individual variability affect the reliability and effectiveness of BCI prosthetics, necessitating continuous research and innovation to overcome these challenges.
Integration with Existing Technologies
Integration with existing technologies is another critical challenge in the realm of BCI prosthetics. As BCI systems continue to evolve, they must work seamlessly with other technological platforms and medical devices. This poses both technical and logistical difficulties.
First, compatibility issues may arise during integration. Many users may have pre-existing medical devices, which must function in harmony with new BCI solutions. Engineers must ensure that communication between devices is secure and effective to prevent operational conflicts.
Another factor to consider is user training. For BCI technology to be effective, users need to understand how to interact with it. This requires educational resources tailored to different user groups, including patients and healthcare providers. If users struggle to adapt to integrated systems, it diminishes the potential benefits of BCIs.
Additionally, the development of standardized protocols is essential. Without clear guidelines on how BCIs should interface with existing systems, varying implementations can lead to inefficiencies and safety concerns. Collaboration among manufacturers, regulatory bodies, and healthcare professionals will be key to achieving effective integration.
Future Directions in BCI Prosthetics
The development of Brain-Computer Interface (BCI) prosthetics stands at the forefront of advancements in medical technology. As science progresses, future directions take on significant importance. These directions not only encompass technological innovations but also aim to address the practical needs of users. Understanding what lies ahead in BCI prosthetics helps stakeholdersβincluding researchers, developers, and usersβprepare for upcoming changes and enhancements.
Emerging Technologies
Emerging technologies play a crucial role in shaping the future of BCI prosthetics. Researchers are continually developing new materials, algorithms, and devices that enhance the functionality and usability of BCIs. Some areas of focus include:
- Flexible Electronics: These are lightweight and adaptable, improving comfort and user experience.
- Advanced Signal Processing: Sophisticated algorithms will allow to decode neural signals more accurately, leading to refined control over prosthetic devices.
- Artificial Intelligence: AI can improve the responsiveness of BCIs while learning individual patterns of user behavior.
- Wireless Technology: Enhanced connectivity ensures that BCIs can operate seamlessly within varied environments.
These technologies represent not just progress but the potential for a new wave of prosthetic devices that better integrate with the user's intentions.
Potential Societal Impact
The societal implications of advancements in BCI prosthetics are vast and multifaceted. As the technology develops, several areas could profoundly change:
- Increased Independence: Enhanced prosthetics can significantly improve the quality of life for individuals with disabilities, allowing them greater autonomy.
- Rethinking Rehabilitation: New approaches in therapy and recovery could emerge, focusing on integrating BCIs into everyday activities.
- Economic Considerations: Investment in BCI technology may influence healthcare costs, as effective BCIs could reduce long-term care needs.
- Ethical Discussions: As capabilities expand, ongoing discussions about the ethical implications will be vital. Issues surrounding privacy, consent, and data security are factors that society must navigate thoughtfully.
Understanding the potential societal changes driven by BCI technology is essential for ensuring a beneficial future.
In sum, the future of BCI prosthetics is promising and laden with possibilities. As technology advances, it will be imperative to consider both its technological and societal implications. Bridge-building between these sectors will foster an inclusive and innovative environment for all involved.
Case Studies in BCI Prosthetic Implementation
Case studies in BCI prosthetic implementation play a critical role in advancing our understanding of brain-computer interfaces. They offer real-world examples that illustrate both successful outcomes and the challenges faced in the integration of neurotechnology with robotic systems. By examining specific instances, researchers can evaluate the effectiveness of various designs and functionalities, leading to more refined and user-centered developments in the field.
Successful Applications
Successful applications of BCI prosthetics showcase how neural signals can be effectively translated into movements, allowing users to regain functionality lost due to injury or disease. An important example is the work done with the BrainGate2 system. This pioneering effort involves direct brain signals being captured from the motor cortex in individuals with paralysis. The neural data is processed and used to control a robotic arm, allowing the user to perform tasks like grasping and moving objects. Successful outcomes from this study demonstrate the potential for increased independence and improved quality of life for individuals with severe disabilities.
Other cases include the use of implantable devices like the CARMEN system, which has allowed users to manipulate a computer cursor through thought alone. This application confirms the feasibility of non-invasive or minimally invasive BCIs that can facilitate communication and interaction with digital devices efficiently.
In a clinical trial involving the fitting of prosthetic hands, individuals experienced noticeable improvements in their ability to perform daily activities. Users reported feeling a greater sense of control and reduced frustration in engaging with their environment.
Lessons Learned from Failures
While many case studies highlight successes, it is equally important to address failures encountered in BCI prosthetic implementation. One notable lesson comes from early attempts to connect multiple electrodes without sufficient calibration. These systems often suffered from low signal fidelity, leading to inaccurate movements and user disappointment.
Moreover, some projects underestimated the psychological impact on users. Individuals may experience challenges in adjusting to BCI devices, leading to frustration and disengagement. Lessons emphasize the necessity of comprehensive user training and continuous psychological support during rehabilitation.
Failure to ensure robust data privacy measures has also been observed. Cases where user data was mishandled highlight the importance of ethical standards and regulatory frameworks to protect individual sensitivity.
"Every failure brings with it a lesson, paving the way for future advancements."
Through these insights, future research can enhance existing BCI systems by addressing past shortcomings and better tailoring collaboration and technology to user needs.
BCI Prosthetics and Neuroplasticity
The relationship between BCI prosthetics and neuroplasticity is both profound and significant. Neuroplasticity refers to the brain's ability to reorganize itself by forming new neural connections throughout life. This malleability enables the brain to adapt to new situations or challenges, which is particularly important for individuals using BCI prosthetics. Understanding this connection is essential for developing effective neural interfaces that can provide better control and functionality for users with limb loss.
With BCI technology, prosthetic limbs can translate neural signals into movements. This requires the interface to not only read these signals but to also develop a smooth integration between the brain and the device. Neuroplasticity plays a critical role in achieving this integration. As users engage with their BCI prosthetics, their brain learns to associate specific neural patterns with corresponding actions, allowing for more intuitive control over time.
Concept of Neuroplasticity
Neuroplasticity encompasses various mechanisms by which the nervous system can change its activity in response to intrinsic or extrinsic stimuli. It includes functional plasticity, which involves the brain's capacity to shift functions from damaged areas to undamaged ones. In the context of BCI prosthetics, this means that patients may experience changes in their brain's adaptive functions, leading to better user experiences with the prosthetics.
Additionally, structural plasticity, which refers to physical changes in the brain's structure, is also vital. As individuals adjust to BCI usage, repeated interactions can lead to synaptogenesis, where new synapses form, further enhancing the connection between the brain and the prosthetic. Studies show that individuals who extensively practice with their BCIs develop more robust neural pathways that correspond to the device's functionality.
Implications for BCI Users
The implications of neuroplasticity for BCI users are significant in several ways:
- Enhanced Control: As users experience improved learning and adaptation, they may gain enhanced control over their prosthetics. Regular practice with BCIs can retrain the brain, allowing users to generate signals that are more precise.
- Improved Quality of Life: The ability to gain better control over a prosthetic can lead to increased independence. Users will be able to perform daily tasks with greater ease and confidence.
- Potential for Recovery: Research indicates that engaging in activities using a BCI can stimulate neuroplastic responses that may aid in recovery, especially for those with recent limb loss. This could mean that the brain may not only learn to operate the device but also gradually regain lost capabilities.
- Tailored Rehabilitation Programs: Understanding neuroplasticity could lead to customized rehabilitation plans focused on encouraging neural growth and adaptation, resulting in better integration of BCIs into users' lives.
Neuroplasticity opens a new dimension in the effectiveness of BCI prosthetics, suggesting that with proper engagement and rehabilitation, users may experience profound improvements in both their physical capabilities and mental well-being. This intersection of neuroscience and technology is crucial as research continues to advance in the field.
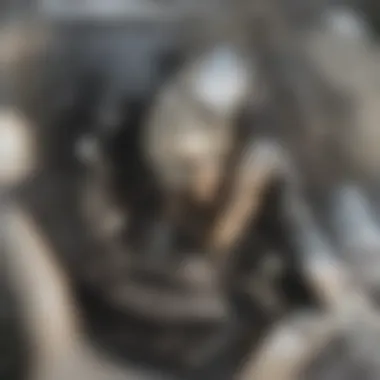
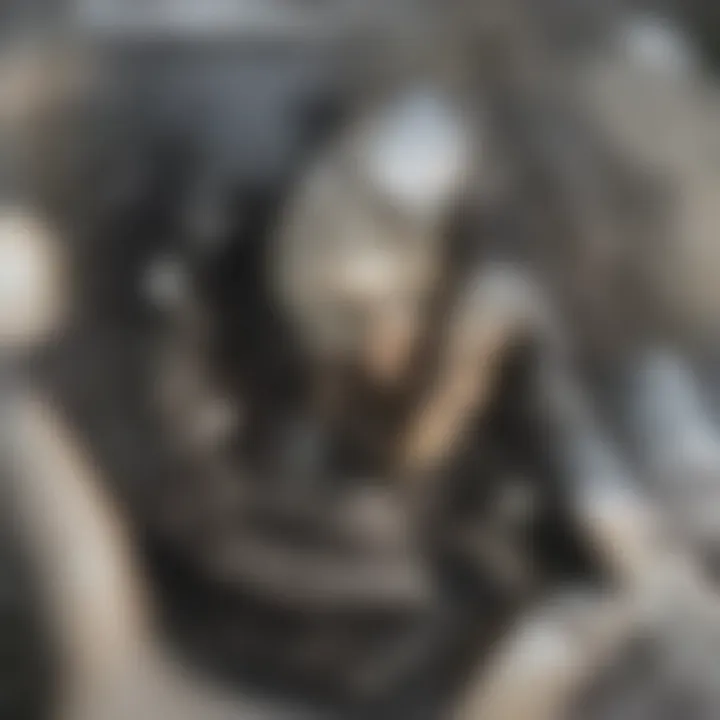
Interdisciplinary Collaboration in BCI Research
Interdisciplinary collaboration plays a crucial role in advancing brain-computer interface (BCI) prosthetics. This complex field requires the integration of knowledge from multiple disciplines to tackle the various challenges involved in developing effective and functional prosthetic devices. The blending of neuroscience, engineering, computer science, and ethics provides a more holistic approach to BCI research.
Through collaboration, researchers can pool their insights and skills. For instance, neuroscientists contribute their understanding of brain activity and neural signals, while engineers apply their technical expertise to develop the hardware and software needed to process these signals. This synergy enables the creation of BCIs that are not only scientifically sound but also practically applicable in real-world scenarios.
The benefits of this collaborative approach are manifold. It fosters innovation by encouraging creative problem-solving and the exchange of ideas. For example, recent breakthroughs in signal decoding have emerged from joint efforts between biomedical engineers and neurologists. This partnership has led to more sophisticated algorithms capable of interpreting complex neural signals. Thus, interdisciplinary collaboration is essential for pushing the boundaries of what is possible in BCI technology.
Furthermore, the ethical considerations surrounding BCI development necessitate multi-sector dialogue. Experts from ethics, law, and sociology can offer critical perspectives on user privacy and the implications of data collection. Addressing these concerns early in the research process is vital because it promotes responsible innovation and fosters public trust in BCI technologies.
"Collaboration not only accelerates discovery but also ensures that we remain responsible stewards of technology, considering its broader impact on society."
In summary, interdisciplinary collaboration in BCI research is fundamental for achieving advancements in prosthetics. By harnessing diverse expertise, researchers can drive innovation while addressing ethical and practical challenges. As BCI technology continues to evolve, this collaborative framework will be pivotal in realizing its full potential.
Role of Neuroscience
Neuroscience serves as the foundation of BCI technology. It provides essential insights into how the brain communicates and functions. Understanding the mechanisms of neural communication allows researchers to design effective methods for translating thoughts into actions.
Neuroscientists study the brain's electrical patterns, focusing on areas responsible for movement and sensory feedback. By deciphering these signals, they contribute significantly to the development of electrodes and signal processing techniques that are integral to BCI systems.
Contributions from Engineering
Engineering contributes the technical expertise needed to build the prosthetic devices that interface with the brain. Engineers focus on the design and fabrication of hardware, ensuring that it is both functional and comfortable for users.
Advances in material science and robotics, such as lightweight materials and precise motor controls, enhance the usability of BCI prosthetics. Moreover, engineering plays a vital role in software development, creating algorithms that efficiently decode neural signals and translate them into meaningful actions.
The collaboration between neuroscience and engineering ignites innovation, thus driving the field of BCI prosthetics forward.
Regulatory and Safety Aspects of BCI Prosthetics
The regulatory and safety aspects of BCI prosthetics are crucial in ensuring that these innovative devices provide benefits to users without compromising their health or well-being. This area of consideration encompasses multiple factors including clinical trials, safety standards, and ethical considerations. The integration of advanced technology in human health demands rigorous oversight, since BCIs interact directly with the brain and nervous system. Regulatory frameworks must thus be robust to guide the development, approval, and monitoring of these devices.
Clinical Trials and Approvals
Clinical trials serve as the backbone of verifying the effectiveness and safety of BCI prosthetics. Prior to wide-scale implementation, these devices must undergo rigorous testing to assess their functionality in real-world settings. Clinical trials typically follow a phased approach:
- Preclinical Studies: Focus on laboratory and animal testing to assess initial safety and efficacy.
- Phase I Trials: Test on a small group of human participants to evaluate safety, determine safe dosage ranges, and identify side effects.
- Phase II Trials: Involve a larger group to further assess efficacy and optimal dosages while continuing safety evaluations.
- Phase III Trials: Compare the BCI prosthetic against standard treatments to evaluate its effectiveness in diverse populations.
The pathway to regulatory approval typically involves submission of data to agencies like the FDA in the United States or EMA in Europe. Through these regulatory bodies, a thorough review process occurs to evaluate data from a variety of aspects, including user safety and device reliability.
Safety Protocols and Standards
Safety protocols and standards are essential for minimizing risks associated with BCI prosthetics. A few key safety considerations include:
- Device Biocompatibility: BCI implants must not trigger adverse reactions within the body. Testing ensures that the materials used are safe and compatible with human tissue.
- Electrical Safety: BCIs must operate without causing electrical interference with other medical devices or unintended neural stimulation. This aspect is further evaluated through rigorous testing protocols.
- User Training and Support: Users should be informed about potential risks, device operation, and maintenance to ensure safe usage. Support systems for ongoing monitoring and feedback during the trial and after commercial use are also vital.
Moreover, adherence to international standards such as ISO 14971 for medical devices risk management is crucial in the design and implementation stage. As BCIs evolve, continuous assessment of safety protocols must adapt to new findings from ongoing research.
"Understanding and addressing regulatory requirements is not just a legal obligation, it is an ethical responsibility that ensures user safety and promotes trust in emerging technologies."
Public Perception of BCI Technology
Public perception serves as a critical barometer for the development and implementation of brain-computer interface (BCI) prosthetics. Understanding how the general populace views this technology can significantly influence funding, research directions, and policy frameworks. Positive public perception can lead to increased investment and acceptance, while negative sentiment may hinder progress and adoption. Given the implications of BCI technology on mental health, autonomy, and personal privacy, it is essential to take into account the collective beliefs and feelings toward these advancements.
Surveys and Studies
Numerous surveys and studies aim to gauge public opinion on BCI technologies. Research often reveals a mixed understanding of the risks and benefits associated with BCIs. Many individuals express interest in how these devices can enhance quality of life but simultaneously harbor concerns regarding safety and ethical implications.
- A survey conducted by Pew Research Center found that about 60% of respondents viewed BCI technology as a positive development, especially in its ability to restore lost functions.
- Conversely, around 40% expressed worries regarding issues such as data privacy and the potential for misuse of neural data.
Key Points from Surveys:
- A significant portion of individuals is optimistic about the potential of BCI.
- Concerns about ethical considerations remain prevalent.
- There is a desire for more information and transparency from technologists and developers.
This psychological landscape underscores the necessity for ongoing dialogue between scientists, developers, and the public. Addressing these concerns in clear terms can foster a better understanding and acceptance of BCI technology.
Influence of Media
The media plays a pivotal role in shaping public perceptions of BCI technology. Coverage ranges from academic journals to mainstream news outlets, affecting how the average person perceives advancements in this field.
- Positive Media Influence: When the media highlights successful applications of BCI, such as individuals regaining mobility or improved rehabilitation outcomes, public interest tends to rise. This can stimulate funding and initiative from both public and private sectors.
- Negative Media Influence: Conversely, sensationalized reporting about risks and ethical dilemmas, like hacking of implanted devices, can generate misunderstandings and fear. Misrepresentation can lead to public pushback against innovations meant to help those with disabilities.
"Media representation can determine the extent to which BCI technology is embraced or feared in society. It is vital that media portrayals remain balanced and factual."
The narrative around BCI technology, therefore, must strive for accuracy while also communicating hope and potential. Educational campaigns can help demystify BCIs and create a more informed public that is receptive to these technologies.
The Path Forward for BCI Prosthetics
The intersection of neuroscience and robotics is progressing rapidly, especially in the area of BCI prosthetics. Evaluating the future path for these technologies is essential. This topic is increasingly relevant for understanding how we can enhance the quality of life for individuals with disabilities. Advancements in BCI prosthetics hold the promise for not only improved motor functions but also greater autonomy and dignity in the usersβ lives.
Goals for Future Research
- Enhancing Signal Acquisition Techniques
Future research should focus on improving how we collect neural signals from the brain. This includes exploring higher-resolution imaging techniques or advanced electrode arrays. Efficient signal acquisition is crucial. It determines how well the prosthetics will function. - Developing Adaptive Algorithms
Adaptive algorithms hold the potential for significant advances. Future systems need to learn from users over time. Such systems can adjust to the unique signals of the brain leading to a more intuitive control of prosthetics. These algorithms must be robust, able to adapt to changes in the user's neurological condition over time. - Improving User Interaction
Understanding the user experience is vital. Research must delve into how people interact with their devices. This entails user-centric designs that are responsive to user preferences and needs. An enhanced interaction could lead to better satisfaction and usability of prosthetics. - Exploring Neuroplasticityβs Role
Investigating how neuroplasticity affects brain-computer interaction can provide insights into training methods for users. Understanding this could allow for the development of tailored rehabilitation programs, maximizing the potential for adaptation to new devices.
Long-Term Vision for Integration
The long-term vision for integrating BCI prosthetics into society is multifaceted. Here are some critical aspects:
- Seamless Integration into Everyday Life
BCI prosthetics should become indistinguishable from natural limbs. Users should feel they are extensions of the body, not merely tools. Achieving this requires advancements in both technology and engineering, ensuring these devices can withstand daily use without hindrance. - Collaborative and Interdisciplinary Efforts
Collaboration among neuroscientists, engineers, and healthcare professionals is key. By working together, they can pool insights and develop holistic approaches to optimize outcomes. This interdisciplinary teamwork can push the boundaries of what BCI can achieve. - Responsible Ethical Practices
Future development must be guided by a strong ethical framework. As BCI technologies evolve, guidelines for usage should ensure the protection of personal data and promote equity in access to such innovations. Addressing ethical concerns from the start can help build trust and acceptance within the public. - Real-time Feedback Systems
Future systems should involve real-time feedback that simulate natural responses. This requires advancements in sensory mechanisms, letting users experience sensation through their prosthetics. Real-time input from the device can help refine control mechanics, further bridging the gap between robotics and human intent.
"The future of BCI prosthetics depends on continued research and collaboration across disciplines."
Through addressing these goals and maintaining a clear vision, BCI prosthetics can transform from experimental to practical applications that significantly enhance lif. Users can reclaim abilities once thought lost, fostering independence and improved human experience.
Closure
The exploration of BCI prosthetics represents not only a significant technological advancement but also a consequential turning point in the interface between human cognition and artificial devices. This intersection amplifies our understanding of neuroscience while pushing the boundaries of what robotic limbs can achieve. The insights gained throughout this article underline the multifaceted importance of BCI prosthetics, which integrate technology and biology in innovative ways.
Summary of Key Points
- Neuroscience Foundations: Understanding brain functionality is critical in developing effective BCI systems. Neural signals decoded from various areas of the brain inform how prosthetics can mimic natural movement.
- Types of BCI Systems: The distinction between invasive and non-invasive systems shapes the approach to treatment and user adaptability. Each type has its unique benefits and limitations.
- Applications and Advancements: Current uses of BCI technology in medicine, especially in rehabilitation, show promise. Enhanced motor control can lead to functional independence for individuals with limb loss.
- Ethics and Regulations: As with any technology that interfaces directly with the human brain, ethical considerations and adherence to regulatory standards are paramount. Data privacy and safety protocols cannot be overlooked.
- Future Directions: The constant evolution of BCI technology suggests a need for ongoing research focused on user experience, integration of new technologies, and a longer-term vision for functional improvement in prosthetics.
Final Thoughts on BCI Future
The future of BCI prosthetics looks promising, presenting both opportunities and challenges. The potential for enhanced life quality for users motivates researchers and developers to persist in their efforts. With advancing technologies, we expect to see better control, increased comfort, and more personalized experiences for users.
"The ongoing integration of artistic design, neuroscience, and advanced engineering positions BCI technology to revolutionize how we approach prosthetics and rehabilitation."